FIGURE 28.1 jVO2 flow chart.
2. Elevated SjVO2 is a marker of either luxuriant blood flow (or hyperemia) or diminished ability of the brain tissue to extract oxygen. Hyperemia is most often a result of loss of autoregulation or a restoration of normal flow to an area that was previously distal to a stenotic lesion. Cautious lowering of the arterial blood pressure may be beneficial recognizing that other areas of the brain may then be put at risk for inadequate flow. Elevated saturations may also represent the presence of an arteriovenous fistula. In cases of extreme intracranial hypertension, arterial blood may actually be shunted past the capillary beds into the venous circulation, thereby elevating the SjVO2. Emergent management of intracranial hypertension is mandated in this case. Decreased oxygen extraction is the result of injury at the cellular level and remains difficult to treat.
CLINICAL PEARL
A low SjVO2 reflects an increase in oxygen extraction and should prompt a search for either increased metabolic demand or decreased supply of oxygen. A high SjVO2 may represent excess blood flow (hyperemia) or shunting of arterial blood into the venous drainage.
3. Pitfalls and limitations
a. Artifact. It may result from contamination by facial or other extra-CBF if the catheter is not sufficiently cephalad, so initial placement and interval reassessment of catheter position are crucial. Furthermore, most oximetric devices provide a measure of signal quality, which can be degraded by changes in pulsatility, hemodilution, adherent or adjacent thrombus, or contact with the vessel wall. Poor signal quality may be improved by flushing or repositioning the catheter. Fluctuations in serum hemoglobin concentration should also prompt manual recalibration of an indwelling catheter.
b. Focal or heterogeneous injury. As a global monitor, SjVO2 may be insensitive to focal pathology. Focal areas of increased or decreased supply on the one hand, or demand on the other, may end up mixing before the jugular bulb and result in a normal global saturation.
c. Bohr effect. In the setting of alkalemia, a left shift of the hemoglobin dissociation curve may falsely elevate SjVO2 readings.
d. Brainstem injury. The neurologic importance of the brainstem far exceeds its contribution to venous outflow; therefore, jugular oximetry is of little utility in monitoring the brainstem.
CLINICAL PEARL
As a global monitor, SjVO2 can guide therapy designed to increase DO2 and reduce excess demand, but it is insensitive to focal pathology and brainstem injury.
III. Transcranial Doppler
A. Physiologic rationale
1. In the early 1980s, Aaslid et al. [27] demonstrated that ultrasound waves of 1 to 2 MHz generated by oscillating piezoelectric crystals could be directed through the temporal bone of the human skull and reflected off large intracranial blood vessels (and more specifically the echogenic erythrocytes coursing through them), returning to a microphone in the ultrasound probe and allowing calculation of depth and blood flow velocity using the principle of Doppler shift in wavelength between the original transmitted signal and its returning echoes.
2. It is important to note that unlike duplex ultrasound (simultaneous two-dimensional b-mode and pulse-wave velocity) used in other parts of the body, TCD does not have the spatial resolution necessary to calculate volumetric flow. In fact, the diameter of the vessels of interest cannot be measured using this technique. However, across a wide variety of physiologic conditions, the largest intracranial vessels generally maintain a constant caliber and do not participate significantly in autoregulation [28,29]. If blood viscosity remains stable, changes in blood flow will be proportional to changes in flow velocity. TCD is thus a useful monitor for the identification of changes in blood flow over serial measurements.
6
3. By utilizing m-mode (motion-mode), TCD can also record multiple overlapping velocities over a range of depths. Since both particulate emboli and gas bubbles within the blood have higher reflectivity than the surrounding erythrocytes, this mode can be used to make a semiquantitative assessment of the density of these emboli, referred to as high intensity transient signals (or HITS).
B. Indications
7
1. Detection of vasospasm after subarachnoid hemorrhage: Delayed ischemic deficits remain one of the most important sources of morbidity in patients after rupture of an intracranial aneurysm. Vasospasm is often initially asymptomatic, making early detection of significant clinical interest. Although its predictive value has recently been questioned [30], TCD is routinely used in many centers for the daily assessment of flow velocities in the major intracerebral arteries in patients recovering from aneurysmal SAH. Relevant flow velocities vary by vessel, but in the best-validated vessel, the middle cerebral artery (MCA), velocities between 120 and 200 cm/s represent luminal narrowing of 25% to 50%, and velocities over 200 cm/s are indicative of greater than 50% reduction in luminal diameter [31]. Intracerebral flow velocities must be considered in the context of overall blood flow. The Lindegaard ratio or index compares the mean flow velocity in the MCA with that in the ICA. A ratio of less than 3 is considered representative of hyperemia. A ratio of greater than 5 or 6 is associated with a significant risk of clinically significant cerebral ischemia [32,33].
2. Intracranial vascular occlusion
a. Carotid surgery. TCD has the potential to rapidly detect MCA flow disruption during carotid endarterectomy. In a 2008 meta-analysis, Schnaudigel et al. [34] found that neuromonitoring-guided selective carotid shunting during endarterectomy was associated with a statistically significant reduction in postoperative infarcts when compared with obligate use of shunts. TCD represents a rapid and noninvasive modality that may help guide selective shunting.
b. Cardiac bypass and cerebral perfusion. TCD has also been used to document cerebral perfusion during initiation of CPB for repair of aortic dissection, and to ensure flow during retrograde and selective antegrade cerebral perfusion in the setting of intraoperative circulatory arrest. Rapid identification of a low- or no-flow state allows repositioning of cannulae or relief cerebral outflow obstruction [35–38].
c. Acute ischemic stroke and thrombolysis. TCD can be used to diagnose acute obstruction in the setting of large vessel ischemic stroke [39]. Recent evidence also supports an independent effect of TCD as an adjuvant therapeutic agent versus TPA alone [40,41].
3. Assessment of cerebral autoregulation. Using TCD to measure the effects of intentional alterations in MAP or PaCO2 on cerebral blood flow velocity (CBFV) provides a dynamic test of cerebral autoregulation. TCD has also been used to derive an estimate of CPP in which CPP = (MAP × diastolic CBFV/mean CBFV) + 14 [42]. Continuous monitoring of CPP/MAP compared with time-averaged CBFV values has led to the development of a correlation coefficient known as the mean velocity index (Mx), which has been used as a measure of static autoregulation in conjunction with other multimodality monitoring data to target patient and context-specific optimal CPP [42]. Sorrentino et al. [43] have recently confirmed earlier studies suggesting worse outcomes in TBI patients with impaired autoregulation, and found that Mx less than 0.05 was associated with intact autoregulation whereas an Mx of greater than 0.3 implies significant impairment.
8
4. Noninvasive ICP estimation. Pulsatility index, defined as systolic CBFV − (diastolic CBFV/mean CBFV) has been used as a noninvasive approximation of ICP [43].
5. Detection of shunt and embolism. By providing semiquantitative detection of HITS (see above) in the intracranial circulation, TCD can facilitate the detection of intracardiac, intrapulmonary, or other right to left shunts, as well as ipsilateral microemboli in the setting to carotid or intracranial dissection, although it cannot reliably distinguish between these entities. TCD may also facilitate the rapid detection of massive intraoperative venous air embolism [44]. TCD-guided therapies have been noted to lessen the effects of embolization once recognized, for example, by encouraging less manipulation of the aorta or carotid [45], or by stimulating changes in surgical and perfusion techniques such as cardiotomy suction [46].
6. Stroke risk in sickle cell anemia. TCD has become a well-established screening tool in the management of sickle cell anemia. Strong evidence has accumulated that increased flow velocities over 170 cm/sec are indicative of a need for transfusion to reduce elevated stroke risk, and in a randomized trial, use of this threshold was associated with a 92% absolute stroke risk reduction [47].
C. Contraindications. There are essentially no contraindications to TCD. Continuous, high-power ultrasonography may have effects on body tissues, but these have not been clearly identified and there is no significant evidence of risk to date.
D. Technique
1. Accurate insonation of the relevant cerebral vasculature requires the probe to be perpendicular to the vessel of interest at the appropriate depth, making TCD highly operator dependent. Nevertheless, interobserver agreement appears to be acceptable for skilled practitioners who perform frequent examinations [48].
2. In addition to the transtemporal approach, additional windows have been standardized including the transocular, foramen magnum, and retromandibular approach.
3. In most patients it is thus possible to monitor blood flow through the internal carotid, the carotid siphon, the anterior cerebral artery (ACA), MCA, the posterior cerebral artery (PCA), the basilar artery, and the vertebral arteries.
4. Examinations are generally performed in serial fashion. Although often impractical, continuous monitoring is possible.
5. Due to variations in skull and vessel anatomy, a significant number of patients will be missing one or more sonographic windows, making a full TCD examination impossible in these patients. Alternative insonation points may be available but may also exacerbate interobserver variability.
CLINICAL PEARL
TCD is an appealing modality because it is noninvasive, real time, and has been applied to a wide range of clinical questions. In most patients, flow velocity can be monitored through the ACA, the MCA, the PCA, the basilar artery, and the vertebral arteries. Serial measurements can help to detect and treat alterations in flow velocity, an indicator of CBF.
E. Interpretation. TCD is an appealing modality because it is noninvasive, real time, and has been applied to a wide range of clinical questions. Nevertheless, interpretation of TCD data must be understood in terms of two of its limitations.
1. TCD measures flow velocity, not flow. In other words, although basal cerebral vessel diameter appears to change relatively little, certain conditions, such as severe acid–base disturbances, changes in blood viscosity, or significant hypercapnea may alter the relationship between velocity and flow. In these instances, a high-measured velocity may reflect either hyper- or hypoperfusion. Similarly, a low-measured velocity may represent vessel occlusion, low global flow, or a misplaced probe. In either case, alternative forms of investigation may be required.
2. TCD does not identify cause. Measurement of flow does not explain why the flow is high, normal, or low. The information TCD provides is best understood in context. For this reason, TCD is most often used in conjunction with additional diagnostic and monitoring modalities.
CLINICAL PEARL
TCD is highly operator dependent and is not diagnostic of anything other than blood flow velocity. Since it measures flow velocity and not blood flow itself, its utility relies on simultaneous interpretation of alternative sources of clinical and imaging data.
IV. Brain tissue oxygen monitoring
A. Physiologic rationale
9
1. What does it measure? Brain tissue oxygen monitoring (PbtO2) involves direct measurement of the partial pressure of oxygen in the interstitium of the brain. The underlying physiology, however, has yet to be fully elucidated. Experimental evidence suggests that PbtO2 depends, in part, on arterial oxygen tension (PaO2) and CBF. Other factors influencing PbtO2 include FiO2, hemoglobin concentration, MAP, and CPP. PbtO2 almost certainly reflects the balance between local oxygen delivery and cellular oxygen consumption [49]. But unlike the SjVO2 it is also a more direct measure of local diffusion of oxygen, or the amount of oxygen available to the surrounding brain tissue [50]. Drawing on experimental oxygen, PaCO2, and blood pressure challenges in a series of patients with TBI, Rosenthal et al. [51] have proposed that PbtO2 is equal to the product of CBF and the arteriovenous oxygen tension difference (AVTO2).
2. Normal values. Mitochondrial function in the brain is dependent on an oxygen tension of approximately 1.5 mm Hg [52]. Physiologic studies indicate that this threshold corresponds to a measured PbtO2 of 15 mm Hg to 20 mm Hg [50]. Estimates of normal brain tissue oxygen levels suggest a range of 25 mm Hg to 48 mm Hg [33,53]. Since small differences in cellular oxygen tension can have significant effects on cellular metabolism, it is biologically plausible that treatment strategies aimed at maintaining PbtO2 greater than 20 mm Hg will reduce secondary injury and improve outcomes in patients with acute brain injury. PbtO2 values less than 20 mm Hg have been defined as moderate brain hypoxia and values less than 10 mm Hg as severe brain hypoxia [54,55]. Alternatively, the Brain Trauma Foundation has proposed 15 mm Hg as a critical threshold for initiation of treatment aimed at increasing PbtO2 [56].
CLINICAL PEARL
PbtO2 reflects the balance between local oxygen delivery and cellular oxygen consumption. Thus it is useful in guiding changes in global factors to include FiO2, hemoglobin concentration, MAP, and CPP.
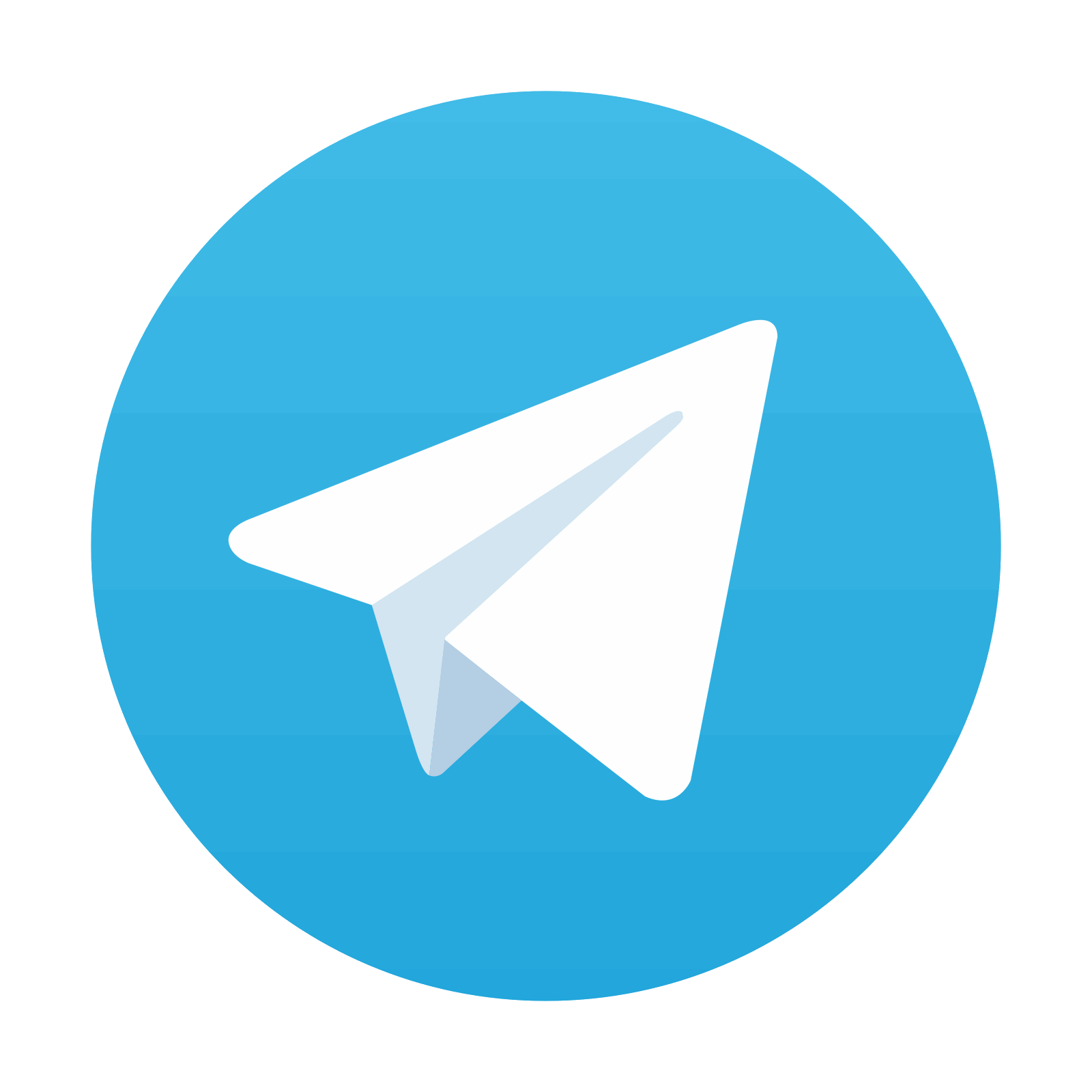
Stay updated, free articles. Join our Telegram channel

Full access? Get Clinical Tree
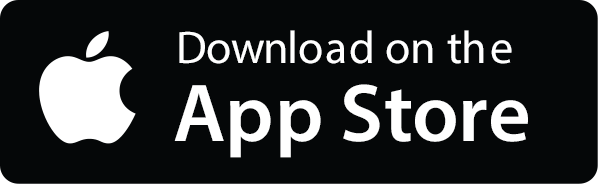
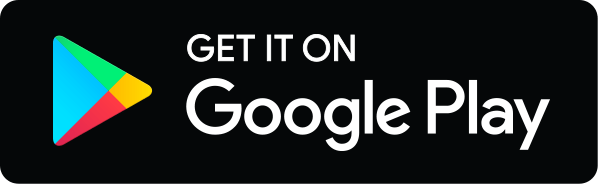