30 Orthopedic and Spinal Surgery
ANESTHESIA FOR ORTHOPEDIC AND SPINAL SURGERY provides a multitude of challenges. Children often present with concomitant diseases that affect cardiovascular and respiratory function. The ability to maintain a clear airway during anesthesia is not straightforward for some children, such as those with arthrogryposis multiplex congenita.1 Operating times can be protracted. Significant blood loss can occur that requires strategies for blood product management and transfusion reduction (see Chapter 10). Major trauma causing orthopedic injuries invariably involves other organ systems that may adversely interact with or compromise anesthesia management (see Chapter 38). The risks of aspiration of gastric contents into the lungs and the requisite fasting times, after even minor trauma involving an isolated forearm fracture, continue to be debated. Fat embolus is uncommon in children with long-bone fractures but should be considered in any child with hypoxia and altered consciousness in the perioperative period.2 Tumor surgery may be complicated by chemotherapy, altered drug disposition, or bone grafting considerations akin to those for plastic and reconstructive surgery (see Chapter 33) and complex postoperative pain management may be required (e.g., phantom pain, reflex sympathetic dystrophy) (see Chapter 44).
Children with chronic illnesses present repeatedly for surgical or diagnostic procedures. A single bad experience can blight attitudes about anesthesia for a long time. Positioning children on the operating table involves care, especially for those with limb deformities and contractures (Video 30-1). Padding, pillows, and special frames are required to protect against damage from inadvertent pressure ischemia while achieving the best posture for surgery. Plaster application, particularly around the hip, should allow for bowel and bladder function, avoid skin breakdown due to pressure or friction, and allow access to epidural catheters. Postoperative management of casts on peripheral limbs must account for the possibility of compartment syndromes attributable to restrictive casts or compartment pathology. Major plexus blocks may mask pressure effects under plaster casts or compartment syndrome, but epidural blocks using low-dose amide anesthetics are ineffective against the discomfort of pressure.3,4 Intraoperative temperature regulation may be affected by tourniquet application or disease (e.g., osteogenesis imperfecta, arthrogryposis multiplex congenita). The use of radiology is common during orthopedic surgery, and precautions against excessive radiation exposure should not be neglected by the anesthesiologist.
Regional anesthesia (see Chapter 41) reduces anesthesia requirements intraoperatively and provides analgesia postoperatively. The use of ultrasound techniques to locate neural tissue improves success and reduces local anesthetic doses (see Chapter 42).5,6 This has heralded increasing use of peripheral nerve blockade rather than central blockade for unilateral lower limb surgery. Acetaminophen (paracetamol) and nonsteroidal antiinflammatory drugs (NSAIDs) are the most common analgesics prescribed for moderate pain. Regular administration of acetaminophen and NSAIDs decreases the amount of systemic opioids administered,7 but NSAIDs decrease osteogenic activity and may increase the incidence of nonunion after spinal fusion.8,9 Intravenous acetaminophen improves the early effectiveness of this drug before the child is able to tolerate oral intake, but this formulation is not available in all countries.10 Long-term pain associated with limb-lengthening techniques (e.g., Ilizarov frame) may require oral opioids after hospital discharge.
Scoliosis Surgery
Children presenting for scoliosis surgery represent a spectrum, ranging from uncomplicated adolescents to severely compromised patients with neuromuscular disease, respiratory failure, and cardiac problems. The age range at presentation varies from infancy to young adulthood. Anesthesia techniques for scoliosis surgery vary with individual patient requirements.11,12 Approaches aimed at minimizing blood loss and transfusion requirements have progressed from extremes of hypotension and hemodilution to a more balanced approach involving moderate degrees of both, use of antifibrinolytic agents, predonation programs, and intraoperative cell salvage. The impact of anesthetic agents on complex physiologic signals has become increasingly important as more sophisticated measurements of neural transmission using somatosensory evoked potentials (SSEPs) and motor evoked potentials (MEPs) have become the standard of care.
Terminology, History, and Surgical Development
Early Hindu literature (3500 to 1800 bc) describes Lord Krishna curing a woman whose back was “deformed in three places.”13 The terms scoliosis (i.e., crooked), kyphosis (i.e., humpbacked), and lordosis (i.e., bent backward) originated with the Greek physician Galen. Scoliosis is a lateral deviation of the normal vertical line of the spine, which is greater than 10 degrees when measured by radiographs. Scoliosis consists of a lateral curvature of the spine with rotation of the vertebrae within the curve. Lordosis refers to an anterior angulation of the spine in the sagittal plane, and kyphosis refers to a posterior angulation of the spine as evaluated on a side view of the spine. Curves may be simple or complex, flexible or rigid, and structural or nonstructural. Primary curves are the earliest to appear and occur most frequently in the thoracic and lumbar regions. Secondary (or compensatory) curves can develop above or below the primary curve and evolve to maintain normal body alignment. Various combinations of curve types have different pathophysiologic consequences.
The magnitude of the scoliosis curve is most commonly measured using the Cobb method.14 Measurement is made from an anteroposterior radiograph and requires accurate identification of the upper and lower end vertebrae involved with the curve. These vertebrae tilt most severely toward the concavity of the curve. The Cobb method of angle measurement is shown in Figure 30-1.

FIGURE 30-1 Diagram of an anteroposterior spinal radiograph shows the Cobb method of scoliosis curve measurement.
Hippocrates (circa 400 bc) developed treatments that relied primarily on manipulation and traction, using an elaborate traction table called a scamnum.15 Nonsurgical treatments for spinal deformities persisted until 1839, when a surgical treatment in the form of a subcutaneous tenotomy and myotomy was described by a French surgeon, Jules Guerin.16
Posterior spinal fusion was first described by Russell Hibbs for tuberculous spinal deformity in 1911.17 The original spinal instrumentation system was the Harrington rod system.18 Modification of this technique that allowed segmental fixation of the rods and early mobilization followed.19 These systems treated the lateral curve but did not allow for correction of the axial rotation. Subsequent developments allowed both corrections by cantilever maneuvers using Cotrel-Dubousset instrumentation.20
Pedicle screws rather than hooks were the next advance. They were initially used as a distal anchor for lumber curves and were found to enhance correction and stabilization, even when used with hooks for the more proximal curves (i.e., hybrid constructs).21 Pedicle screw instrumentation techniques for total curve correction are a recent development that offer better curve correction than hook techniques 22 and the hybrid pedicle screw and hook technique.23
Classification
Classification of scoliosis deformities is imperfect because the systems used are clinically rather than etiologically based. Most classifications are surgically based and used for surgical decision making. Curves can be described on the basis of age at onset, associated pathology, and anatomic configurations of the curve, such as single, double, or triple curves; amount of pelvic tilt; curve flexibility; and three-dimensional analysis of the curve.24 A classification that could indicate the risk of an adverse outcome of anesthesia, particularly respiratory failure, would be of clinical benefit. Children younger than 5 years of age with early-onset scoliosis or with independent cardiac or pulmonary disease appear to be at increased risk for respiratory failure, whereas those with idiopathic scoliosis in whom the curve develops at adolescence appear to have minimal risk.25 A classification adapted from that proposed by the Scoliosis Research Society in 1973 remains relevant for anesthesiologists (Table 30-1).26
The Lenke classification system, developed in 2001 for idiopathic scoliosis, provides a means to categorize curves and guide surgical treatment.27 It is increasingly used by the surgeons as an integral part of their decision making.28 Major and structural minor curves included in the instrumentation and fusion are used for the classification, and the nonstructural minor curves are excluded. The system has three components: curve type, a lumbar spine modifier, and a sagittal thoracic modifier. The resulting six curve types have specific radiographic characteristics that differentiate structural and nonstructural curves as proximal thoracic, main thoracic, and thoracolumbar/lumbar regions such that the number, curve type, and main structural curves are related as follows:
Type 1, main thoracic: single; main thoracic structural curve
Type 2, double thoracic: double; proximal, and main thoracic structural curves
Type 3, double major: double; main thoracic (major curve) and thoracolumbar/lumbar structural curves
Type 4, triple major: triple; all three structural curves
Type 5, thoracolumbar/lumbar: single; thoracolumbar/lumbar structural curve
Type 6, thoracolumbar/lumbar main thoracic: double; thoracolumbar/lumbar (major curve) and main thoracic structural curves
In types 1 through 4, the main thoracic curve is the major curve, and in types 5 and 6, the thoracolumbar/lumbar curve is the major curve.
Pathophysiology and Natural History
Vertebral rotation and rib cage deformity usually accompany any lateral curvature. With progression of the curve, the vertebral bodies in the area of the primary curve rotate the convex aspect of the curve and the spinous process to the concave side. This vertebral rotation can be determined by measurement of the position of the pedicles from the midline (i.e., Moe method).29 The vertebral bodies and the discs develop a wedge-shaped appearance, with the apex of the wedge toward the concave side. On the convex side of the curve, the ribs are pushed posteriorly, which narrows the thoracic cavity and causes the characteristic hump. On the concave side, the same rotation forces the ribs laterally, with consequent crowding toward their lateral margins (Fig. 30-2). These changes result in an increasingly restrictive lung defect. Exactly when this becomes a problem depends on the child’s accompanying pathology. The thoracic and lumbar regions are the most common sites of the primary curve. In children in whom the primary curve is in the lumbar region, the rotation of the vertebral bodies and spinous processes should be taken into consideration when spinal or epidural insertion is attempted because the spinal canal is relatively displaced toward the convex aspect of the curve.

FIGURE 30-2 Characteristic distortion of the vertebra and ribs in thoracic scoliosis.
(Modified from Kleim HA. Scoliosis. Ciba foundation symposium, Vol. 1. Summit, N.J.: Ciba; 1978, p. 609; Gregory GA, editor. Anesthesia for orthopaedic surgery. In: Paediatric anesthesia. 3rd ed. Edinburgh: Churchill Livingstone; 1994.)
The physical distortion in the thorax results in restriction of lung volumes and function. Ventilation depends on the mobility of the thoracic cage, the volume of each hemithorax, and the muscle power and elastic forces required to move the thorax. Children with idiopathic scoliosis with a mild decrease in vital capacity also have reduced forced expired volume at 1 second (FEV1), gas transfer factor, and maximal static expiratory airway pressures (Pemax) (see Fig. 11-4). The predominant deformity of lateral flexion and vertebral rotation results in the lung on the concave side being able to achieve a near-normal end-expiratory position but not end-inspiratory position, whereas the lung on the convex side achieves a normal end-inspiratory position but cannot reach a normal end-expiratory position. The concave side contributes less than normal at total lung capacity, resulting in a decrease in Pemax. Similarly, because the convex side does not reach a normal end-expiratory position, the intercostal muscles and hemidiaphragm will be less efficient, resulting in a reduced maximum static inspiratory airway pressure (Pimax), although this reduction may not be quite so marked.30 The main effect of scoliosis on respiratory function is mechanical, and the anatomic changes in the chest wall cause impaired movement and reduced compliance. Potential long-term respiratory problems when these defects are left untreated include hypoxemia, hypercarbia, recurrent lung infections, and pulmonary hypertension.
Congenital, Infantile, and Juvenile Scoliosis
Congenital spinal anomalies are caused by failures of formation and segmentation that result in scoliosis and kyphosis. The hemivertebra, caused by failure of formation, is the most common anomaly. Fully segmented hemivertebrae contribute to progressive deformity during periods of rapid spinal growth (e.g., first 5 years of life). The most severe deformities are seen in the thoracolumbar spine. Congenital spinal anomalies may be associated with malformations of the ribs, chest wall, and hemifacial microsomia.31 Children with congenital scoliosis have a 25% chance of urologic abnormalities and 10% chance of a cardiac abnormality. Bracing or casting techniques are not effective for this form of scoliosis. Surgical options for these patients include fusion in situ, convex hemiepiphysiodesis, hemivertebra excision, growing rods, and vertical expandable prosthetic titanium rib (VEPTR) treatment.32 Although short-term correction is easily achievable, a short thoracic or even thoracic insufficiency syndrome can result.33 Approximately one half of the children who have extensive thoracic fusions and those whose fusions involve the proximal thoracic spine develop restrictive pulmonary disease (FEV1 <50%).34 Expansion thoracoplasty and stabilization using a VEPTR may be used.35
Infantile and juvenile scoliosis are part of the spectrum of idiopathic scoliosis but are considered here because they manifest and require treatment at an early age. Infantile scoliosis accounts for less than 1% of idiopathic scoliosis and is defined as scoliosis appearing between birth and 3 years of age.32 It usually occurs in the thoracic spine, and the curve is convex to the left. Bracing and serial casting techniques are used for infantile scoliosis. Improvement and resolution in some cases have been achieved at 9-year follow-up.36
Treatment of infantile scoliosis may begin as early as 4 to 5 months of age or as soon as the diagnosis of scoliosis has been made. Body casting appears useful in selected children, such as those with smaller, flexible spinal curves, but curve progression and the need for secondary treatments affect a significant proportion of these children.37 Bracing is considered when the curve reaches 30 degrees.32 Success has also been reported for more severe curves (60 degrees) when casting was started before 20 months.38 After induction of anesthesia, the child is positioned on the frame (first described by Cottrell and Morel), securing the pelvis to the caudal end of the frame and tethering the head by a chin strap to the rostral end. The spine is mildly distracted, but the main maneuver derotates the spine through the ribs (Fig. 30-3, A). General anesthesia with tracheal intubation is required to facilitate positioning the child, stretching the spine, and molding the body cast. Hemoglobin desaturation frequently occurs when the cast is molded to correct the spinal deformity. An oral airway is also needed to prevent compression of the tracheal tube after the chin strap is applied and tightened. After the cast has hardened, it is cut back and trimmed to maintain the correction to the spine while facilitating breathing, gastrointestinal function, and day-to-day living (see Fig. 30-3, B). Halo traction may be used to stretch and improve the curves. Pin infections occur in almost half of the children.39
Juvenile idiopathic scoliosis comprises 10% to 15% of idiopathic scoliosis and is defined as scoliosis that is first diagnosed between the ages of 4 and 10 years. Approximately 20% of these children and those with infantile scoliosis with a curve greater than 20% have an underlying spinal condition, particularly Arnold-Chiari malformation and syringomyelia.40 Although bracing is used to manage these curves, almost all children in this group with curves greater than 30% require surgical intervention.41
Growing rods may be used for congenital, infantile, or juvenile scoliosis to maintain the correction obtained at initial surgery while allowing spinal growth to continue. Several procedures are required before a definitive fusion.42 All the systems (i.e., growing rods and VEPTR) have a moderate complication rate (i.e., rod breakage and hook displacement).VEPTR systems are being used to correct large-magnitude curves in this group of children when conservative treatment is inadequate.43
Idiopathic Scoliosis
Although adolescent idiopathic scoliosis is relatively common, severe morbidity is seen only in children with early-onset (infantile or juvenile) idiopathic scoliosis.44 Respiratory deterioration alone is seldom the reason for surgery in those who develop scoliosis after the age of 5 years.25 This is explained by the fact that the respiratory alveoli are mature by this age.45,46
Scoliosis evolves during growth spurts. The earlier the age of onset and the more immature the bone growth at the time the process begins, the more severe the outcome. The relentless progression of infantile-onset idiopathic scoliosis with rapidly deteriorating curves and lung function is often not amenable to surgery. Treatment involving spinal instrumentation and anterior epiphysiodesis does not prevent the reappearance of the deformity or the decrease in pulmonary function.47
Pulmonary impairment correlates directly with the magnitude of the thoracic curve. Severity of the scoliosis is the most accurate predictor of impaired lung function.48 The morphology of the thoracic curve, the number of vertebrae in the major curve, and the rigidity of the curve also are associated with deteriorating pulmonary function.49 Conventional wisdom has held that there is minimal impact on the vital capacity until the curve exceeds 60 degrees, with clinically relevant decreases in respiratory function occurring only after the thoracic scoliosis has progressed beyond 100 degrees.34 However, it has been shown that children with adolescent idiopathic scoliosis may have pulmonary impairment that is disproportionate to the severity of the scoliosis and that it occurs well before the curve reaches 100 degrees. Forced vital capacity (FVC) may decrease below the normal threshold (<80% of predicted) after the magnitude of the thoracic curve exceeds 70 degrees; FEV1 decreases to less than the normal threshold after the main thoracic curve exceeds 60 degrees.35 Twenty percent of children with a thoracic curve of 50 to 70 degrees have moderate or severe pulmonary impairment (i.e., less than 65% of predicted) (Fig. 30-4, A).50 Those with thoracic hypokyphosis are more likely to have moderate or severe pulmonary impairment; complex curves have a greater prevalence of moderate or severe pulmonary impairment, and the number of vertebrae in the thoracic curve is the most significant predictor of impaired respiratory function (see Fig. 30-4, B).51 These data indicate that children with a structural cephalad thoracic curve, a major thoracic curve spanning eight or more vertebral levels, or thoracic hypokyphosis are at increased risk for moderate to severe pulmonary impairment.
Neuromuscular Scoliosis
Children with neuromuscular scoliosis have the burden of deteriorating muscle function in addition to mechanical distortion. Crowding of the ribs on the concave side of the curve limits chest wall expansion, and the sitting posture restricts diaphragmatic excursion. This inevitably leads to more rapid deterioration in the curve and respiratory function. These children also have the potential for rapid and unpredictable deterioration of the curve.36 It is important to consider the natural history of the specific neuromuscular disease when trying to balance the risks of surgery against conservative management.
Children with Duchenne muscular dystrophy (DMD) suffer from progressive muscular weakness and increasing disability until death occurs, usually by the beginning of the third decade.52 These children tend to become wheelchair bound by 8 to 10 years of age because of increasing motor muscle weakness. Scoliosis then progresses with an acute deterioration during the growth spurt between the ages of 13 and 15 years, such that it becomes difficult or impossible to sit unaided. After the lumbar curve exceeds 35 degrees, further progression becomes inevitable.53 A normal cough requires an inspiratory effort of more than 60% of total lung capacity and effective glottic closure to produce an effective peak flow (more than 160 L/min in adults). Forced expiratory flows are typically reduced proportionally to the decrease in lung volume. As muscle weakness progresses, patients hypoventilate, initially at night. If nocturnal ventilatory support is not provided at this stage, diurnal hypercapnia will result.54
There have been two significant changes in the overall management of children with DMD: the use of steroids and the earlier use of nocturnal noninvasive positive-pressure ventilation (NPPV). Steroid treatment in the early phase of the disease appears to slow disease progression for a few years; treatment with prednisone can stabilize strength and function for 6 months to 2 years.55,56 This may delay the presentation of children for corrective surgery. Earlier adoption of nocturnal NPPV for nocturnal hypoventilation improves survival and quality of life. Clinically unsuspected nocturnal hypoventilation occurs in about 15% of patients with DMD and can be predicted by moderate impairment according to pulmonary function tests (FVC <70% and FEV1 <65% of predicted) and scoliosis. Those with nocturnal hypoventilation have increased gas trapping, decline of muscle strength, and worse perception of health status despite NPPV.57
A 2007 multidisciplinary consensus statement on the “Respiratory and Related Management of Patients with Duchenne Muscular Dystrophy undergoing Anesthesia or Sedation” provided recommendations to standardize the approach to these patients58 and others with flaccid neuromuscular diseases undergoing anesthesia; the most important of these are as follows: An FVC less than 50% of predicted indicates an increase in postoperative respiratory complications, and an FVC less than 30% suggests a further increase in that risk. Respiratory function tests should be part of the preoperative evaluation whenever possible and should include FVC, maximal inspiratory pressure, maximal expiratory pressure, peak cough flow, Spo2 on room air, and Paco2 if the Spo2 value is less than 95%. Consider preoperative training and postoperative use of NPPV if FVC is less than 50% of predicted, and strongly consider NPPV if FVC is less than 30%. Consider preoperative training and postoperative use of manual and mechanically assisted cough in those with impaired cough. In older children, this can be predicted by a peak cough flow less than 270 L/min or maximal expiratory pressure less than 60 cm H2O. Strongly consider planning to extubate the trachea directly to NPPV when the FVC is less than 30%.
Dilated cardiomyopathy occurs in up to 90% of DMD individuals older than 18 years of age. The severity of the physical disability in boys with DMD in their late teens often masks the clinical symptoms of cardiac failure. Cardiomyopathy has been considered responsible for death of up to 20% of individuals with DMD, but this proportion may increase in the future for individuals in whom NPPV prevents respiratory-related mortality.56 (See also Chapter 22.)
Risk Minimization and Improving Outcome from Surgical Intervention
Respiratory Function and Complications in the Early Postoperative Period
Decreases in lung volumes and flow rates similar to thoracic and upper abdominal surgery occur after scoliosis surgery. The FVC and FEV1 decrease with a nadir at 3 days and are about 60% of preoperative values 7 to 10 days after surgery (Fig. 30-5). It is not until 1 to 2 months after surgery that pulmonary function test results again approach baseline values. The magnitude of this decrease is not affected by the type of surgery performed or whether the scoliosis has an idiopathic or neuromuscular cause.59
Children with neuromuscular disease are more likely to require prolonged mechanical ventilation after spinal surgery because of more severe preoperative respiratory impairment.60 The marked decrease in vital capacity and peak flows is undoubtedly related to the risk of postoperative complications, but determining when it is no longer safe to anesthetize those with a restrictive lung defect remains an imperfect science.
Equipment is available to assist the postoperative management of children with impaired respiratory function. The routine use of NPPV and cough augmentation therapy should be planned if the preoperative FVC is less than 30%. Cough augmentation can be provided manually by hyperinflation and forced expiration, alone or together, and by mechanical insufflation-exsufflation (MIE) therapy.61 The effectiveness of MIE may be limited in children with a weak or enlarged tongue if it blocks exsufflation flow.
Less extensive surgery with the newer pedicles screw systems decreases the need for pelvic fixation to correct pelvic obliquity. The procedures require less extensive surgery and shorter operating times, which may benefit children with impaired respiratory function.62–65
Respiratory complications after surgery for idiopathic scoliosis are relatively uncommon. In contrast, respiratory complications after surgery in children with nonidiopathic scoliosis is reported to be fivefold greater.66 The risk increases as the degree of curvature increases and the respiratory function decreases.67 Anterior spinal procedures are associated with a greater incidence of complications than posterior spinal fusion, such that some consider this to be the main risk factor for postoperative respiratory complications.67 Modern pedicle screw systems may decrease the need for anterior procedures, thereby decreasing the complication rate.68
Atelectasis, infiltrates, hemothoraces, pneumothoraces, pleural effusions, and prolonged intubation have the greatest incidence, whereas pneumonia, pulmonary edema, and upper airway obstruction occur less frequently. These problems are more common when the scoliosis is associated with mental retardation and developmental delay. The greatest complication rate occurs for those with cerebral palsy and flaccid neuromuscular scoliosis.66–69 Although respiratory complications increase as the severity of scoliosis and the degree of respiratory impairment increase, reported complication rates vary considerably. Studies of children with neuromuscular scoliosis suggest an overall respiratory complication rate of 15% to 30%69–73 and minimal mortality. In one of these studies, which identified three groups by respiratory impairment (FVC <30%, FVC = 30% to 50%, FVC >50%), an overall complication rate of 31% occurred independent of the degree of respiratory impairment,69 perhaps reflecting improvement with modern management techniques (Table 30-2).
Children with cerebral palsy have additional problems associated with their lack of muscular control (e.g., swallowing incoordination, excessive salivation, gastroesophageal reflux) and sometimes have developmental delay that contribute to a postoperative complication rate of 30%.70,71,74 Nonambulatory patients and those with curves greater than 60 degrees are at increased risk for major complications, with nonambulatory patients almost four times more likely to have a major complication.74 Gastrointestinal dysmotility in cerebral palsy patients can be exacerbated after scoliosis surgery and cause persistent vomiting and bloating.75 Pancreatitis may occur in up to 30% of cerebral palsy patients after surgery, with a greater incidence among those with documented gastroesophageal reflux and reactive airway disease.76
Long-Term Changes
Idiopathic Scoliosis
Improvements in pulmonary function are not impressive after correction of idiopathic scoliosis. Early studies suggested that spinal fusion stabilized the respiratory dysfunction that existed preoperatively, but failed to offer any improvement.77 Improvements may be possible in certain subgroups of patients with some surgical techniques, but it takes months to years for pulmonary function to improve. Children with a preoperative curve less than 90 degrees undergoing a posterior procedure only had a greater than 10% increase in vital capacity, maximum voluntary ventilation, and maximum respiratory mid-flow rate after 2 years; this improvement did not occur in those who underwent anterior surgery.78 Harrington rod instrumentation in children with idiopathic scoliosis resulted in only a small improvement in vital capacity.79
The newer instrumentation systems, such as the Cotrel-Dubousset instrumentation that allows segmental realignment and approximation, result in further improvements in pulmonary volumes.80 Pulmonary function returns to preoperative values within 3 months after the posterior approach using the newer instrumentation systems, with additional improvements occurring and being sustained for 2 years.81 Pedicle screws provide greater curve correction in adolescent idiopathic scoliosis, with a trend toward improved pulmonary function after 2 years compared with other instrumentation techniques.23 A 10-year follow-up analysis demonstrated an absolute increase in the FVC (3.25 versus 3.66 L) and FEV1 (2.77 versus 3.10 L) but no changes in percent of predicted values in children who underwent a posterior fusion and instrumentation only. In the same analysis, those with chest wall disruption experienced no change in FVC and FEV1 over 10 years, but the assessment demonstrated a significant decrease in percent of predicted FVC (85% versus 79%,) and FEV1 values (80% versus 76%).82
Chest cage disruption (i.e., thoracoplasty or anterior thoracotomy) is associated with reduced pulmonary function at 3 months and a 10% to 20% decrease in total lung capacity and FVC. These values do not return to baseline until 1 to 2 years after surgery. Improvements in lung function with this approach are seldom seen.44,45 Video-assisted thoracoscopic surgery (VATS) for anterior release and instrumentation appears to result in less pulmonary morbidity and a smaller decrease in pulmonary function at 3 months. One year after surgery, values for children treated thoracoscopically had returned to baseline, but this did not occur for those undergoing open thoracotomy (Fig. 30-6).83,84 Two- and 5-year follow-up evaluations of those undergoing VATS showed no significant changes with regard to the correction of the major Cobb angle (56% ± 11% and 52% ± 14%, respectively) or average total lung capacity as a percent of the predicted value (95% ± 14% and 91% ± 10%) over the study period.85
Changing surgical techniques may challenge these findings in the future because some surgeons think that anterior fusions with modern systems offer short-term benefits of reduced blood loss and transfusion and long-term benefits due to shorter fusions, better maintenance of thoracic kyphosis, and improved spontaneous lumbar curve correction. A 2-year postoperative study concluded that VATS for thoracic curves and open procedures for thoracolumbar curves resulted in minimal to no permanent pulmonary impairment 6 months after the procedure compared with posterior spinal fusion, despite a short-term decrease observed after VATS.86
Neuromuscular Scoliosis
Improvements in the scoliosis angle and the degree of pelvic obliquity are achieved after spinal instrumentation in children with neuromuscular disease. Significant improvement can usually be shown in the ability to sit unaided, particularly if children are unable to do so beforehand.87–91 Some investigators have reported an increase in the quality of life perceived by the child or caregiver.89,91
There is little evidence for any improvement in respiratory function in this group of children. There may be a period of delay or even stabilization of the inevitable deterioration of respiratory function.52,88,92 Other investigators have shown no difference in respiratory function after 5 years compared with patients managed conservatively90,93 or an early loss in vital capacity after surgery with a progressive decrease of 25% over 4 years, with 66% of patients requiring mechanical respiratory assistance by that time.89 A Cochrane review was unable to find any data evaluating the effectiveness of scoliosis surgery in patients with DMD and even suggested, “Patients should also be informed about the uncertainty of benefits on long-term survival and respiratory function after scoliosis surgery.”94
Some retrospective analyses, however, deserve consideration. A review of the long-term survival of children with DMD after spinal surgery and nocturnal ventilation demonstrated that those having spinal surgery and ventilation had a median survival of 30 years, whereas those receiving nocturnal ventilation only survived to 22.2 years. This result occurred despite a decrease in mean vital capacity from 1.4 to 1.13 L in the first postoperative year.95 Posterior spinal fusion for scoliosis in DMD was associated with a significant slowing in the rate of decrease in respiratory function; the rate of 4% per year before surgery decreased to 1.75% per year (over 8 years) after surgery.96 In a study of 14 children with DMD and an FVC of less than 30%, the mean rate of decrease in percent of FVC after surgery was 3.6% per year. Most children and parents thought scoliosis surgery improved their function, sitting balance, and quality of life and gave it high satisfaction scores.97
Less outcome information is available for children with cerebral palsy. Surgery is perceived as having a positive impact on patients’ quality of life, overall function, and ease of care by parents and other caregivers,98 despite the high complication rates described earlier. A 3-year follow-up after a pedicle screw construct for scoliosis, which reduced the mean Cobb angle to 31%, demonstrated improved functional ability in 42% of children. Most children had improved sitting balance and nursing care requirements. A 32% complication rate occurred; most were pulmonary in origin but ultimately reversible. There were two perioperative deaths and one transient neurologic deficit due to screw impingement among 56 patients.68
Less morbidity has been claimed for the same-day (one-stage) surgery compared with the two-staged approach in children with neuromuscular disease requiring anterior and posterior spinal surgery.99,100 However, it seems reasonable to avoid anterior thoracotomy in neuromuscular patients in view of the poor respiratory function after chest cage disruption.81,83 Currently, pedicle screw systems in children with neuromuscular scoliosis produce outcomes similar to those of earlier systems but with quicker operating times and less blood loss.63
Spinal Cord Injury during Surgery
Etiology
Spinal cord injury can occur by four main mechanisms: direct contusion of the cord during surgical exposure; contusion by hooks, wires, or pedicle screws; distraction by rods or halo traction; and reduction in spinal cord blood flow.101 Epidural hematoma should be included in the differential diagnosis of deficits occurring postoperatively. The areas of the spinal cord most vulnerable to ischemic injury are the motor pathways, which are supplied by a single anterior spinal artery. This is fed in a segmental manner by the radicular arteries that arise from the vertebral, cervical, intercostals, lumbar, and iliolumbar arteries. The largest radicular artery is the artery of Adamkiewicz, which arises between T8 and L4. A watershed area between T4 and T9 is prone to ischemia because the blood supply in this region of the cord is poorest.102 Paraplegia is the most feared neurologic complication, but partial spinal cord injury resulting in areas of localized weakness and numbness as well as bladder and bowel disturbances also have been reported.
The increasing use of pedicle screws in spinal surgery raises the possibility of increased risk to individual nerve roots. A systematic review of pedicle screw complications that involved a total of 4570 pedicle screws in 1666 patients reported an overall 4% malposition rate that increased to 16% in studies that systematically examined their patients postoperatively.103 Eleven patients required revision surgery for the malpositioned screws, and there was one temporary neurologic complication (i.e., epidural hematoma). No vascular injuries were reported, although six cases of aortic abutment were described.
Risk of Spinal Cord Injury and Spinal Cord Monitoring
Combined surveys undertaken by the Scoliosis Research Society and the European Society for Deformities of the Spine investigating idiopathic scoliosis suggested an incidence of neurologic impairment of 0.72% in 1975.104 At the end of the last millennium, that incidence had decreased to less than half (0.3%), and all were partial cord lesions.101 Patients with curves greater than 100 degrees, congenital scoliosis, kyphosis, and postirradiation deformity appear to be at greatest risk for complications. The use of pedicle screws may have increased the immediate neurologic complication rate. Nine neural complications were reported among 1301 patients, for an incidence of 0.69% in 2007. Three thecal penetrations occurred, two as a result of pedicle screws, all without sequelae. There were two nerve root injuries and four spinal cord injuries, all of which resolved within 3 months.105
A retrospective review of 19,360 cases of pediatric scoliosis showed significantly different overall complication rates among idiopathic (6.3%), congenital (10.6%), and neuromuscular (17.9%) scoliosis. Neurologic deficits had a different distribution, with the greatest rate among congenital cases (2%), and lower rates with neuromuscular (1.1%) and idiopathic scoliosis (0.8%).106 Mortality rates of 0.3% were observed for neuromuscular and congenital scoliosis, with an idiopathic scoliosis rate of 0.02%. Rates of new neurologic deficits were greater with anterior screw–only constructs (2%) or wire constructs (1.7%) than with pedicle screw constructs (0.7%).
Spinal cord function is monitored to ensure that the complication rate is as small as possible. The Scoliosis Research Society issued a position statement concluding that neurophysiologic monitoring can assist in the early detection of complications and can possibly prevent postoperative morbidity in patients undergoing operations on the spine. For any monitoring technique to be effective, it needs to have a sensitivity and specificity that allows true changes to be recognized with a very low occurrence of false-negative and false-positive results. The test or technique must also produce its results in a time frame that allows the problem to be reversed or prevented. Recognition of the limitation of individual techniques has seen the development of increasingly sophisticated monitoring systems to identify and minimize this risk. Older tests, such as the wake-up test and ankle clonus test, have largely been superceded by monitoring of SSEPs, MEPs, and triggered electromyographic techniques (EMGs). The importance of using a multimodal approach is increasingly recognized and is well addressed in the literature.107–110 The capabilities and limitations of the various techniques are summarized in E-Table 30-1.
Methods of Monitoring Spinal Cord Function
Wake-up Test
The wake-up test measures gross motor function of the upper and lower extremities. It has been used widely since it was first described.111 The test consists of decreasing the depth of anesthesia almost to the point of wakefulness and asking the patient to respond to verbal commands. Failure to move the feet and toes while being able to squeeze a hand suggests a problem with the spinal cord. The test requires limiting or reversing muscle relaxation and reducing the depth of anesthesia sufficiently to enable the patient to follow commands. When the test was initially described, 3 of 124 patients had a positive result (i.e., no movement) and were saved from paraplegia.111 A major concern is that the test is conducted after maximal spinal correction, which may occur after any neurologic insult has occurred; however, removal or modification of the spinal instrumentation within 3 hours of the onset of the neurologic deficit has been reported to prevent the risk of permanent neurologic sequelae.112 The wake-up test is unlikely to detect isolated nerve root injury or sensory changes. It is limited to neurologically normal patients with an appropriate developmental age who can follow instructions.
With the clinical application of SSEP and MEP monitoring (Fig. 30-7) well established and in the absence of intraoperative changes, there is no justification to perform the wake-up test.113 Nonetheless, some surgeons still regard the wake-up test to be the gold standard, and it may be used to confirm changes demonstrated by SSEP or MEP monitoring.114 Risks include lack of nerve root and sensory information, accidental extubation, dislodgement of the instrumentation, intraoperative recall with subsequent psychological trauma, air embolism, and cardiac ischemia. If a wake-up test is planned, it is prudent to fill the wound with saline to reduce the risk of an air embolism.
Ankle Clonus Test
The ankle clonus test uses the clonus that occurs just before consciousness is regained during wakening from anesthesia. Rhythmic muscle contractions are thought to result from spinal reflexes returning while the higher neurologic centers remain inhibited by anesthesia, and the oscillations demonstrate an intact spinal cord. Inability to demonstrate clonus suggests spinal cord injury.115 Like the wake-up test, it is a post hoc test rather than real-time monitoring. However, in a review of more than 1000 patients undergoing spinal procedures in which six postoperative neurologic deficits occurred, this test identified all the deficits but produced three false-positive findings, giving a sensitivity of 100% and a specificity of 99.7%. In comparison, the wake-up test produced false-negative results for four of the five patients who developed deficits.115
Somatosensory Evoked Potentials
SSEPs involve stimulating a peripheral nerve and measuring the response to that stimulation using scalp electrodes (i.e., cortical SSEPs).116,117 Alternatively, the response can be measured subcortically near the spinal cord by electrodes placed in the epidural space, interspinous ligament, or spinous processes of the vertebrae.118 An intranasally placed pharyngeal electrode can act as a surrogate for these. The advantage of the subcortical evoked potential is that the responses are more stable, reproducible, and resistant to the effects of anesthesia.
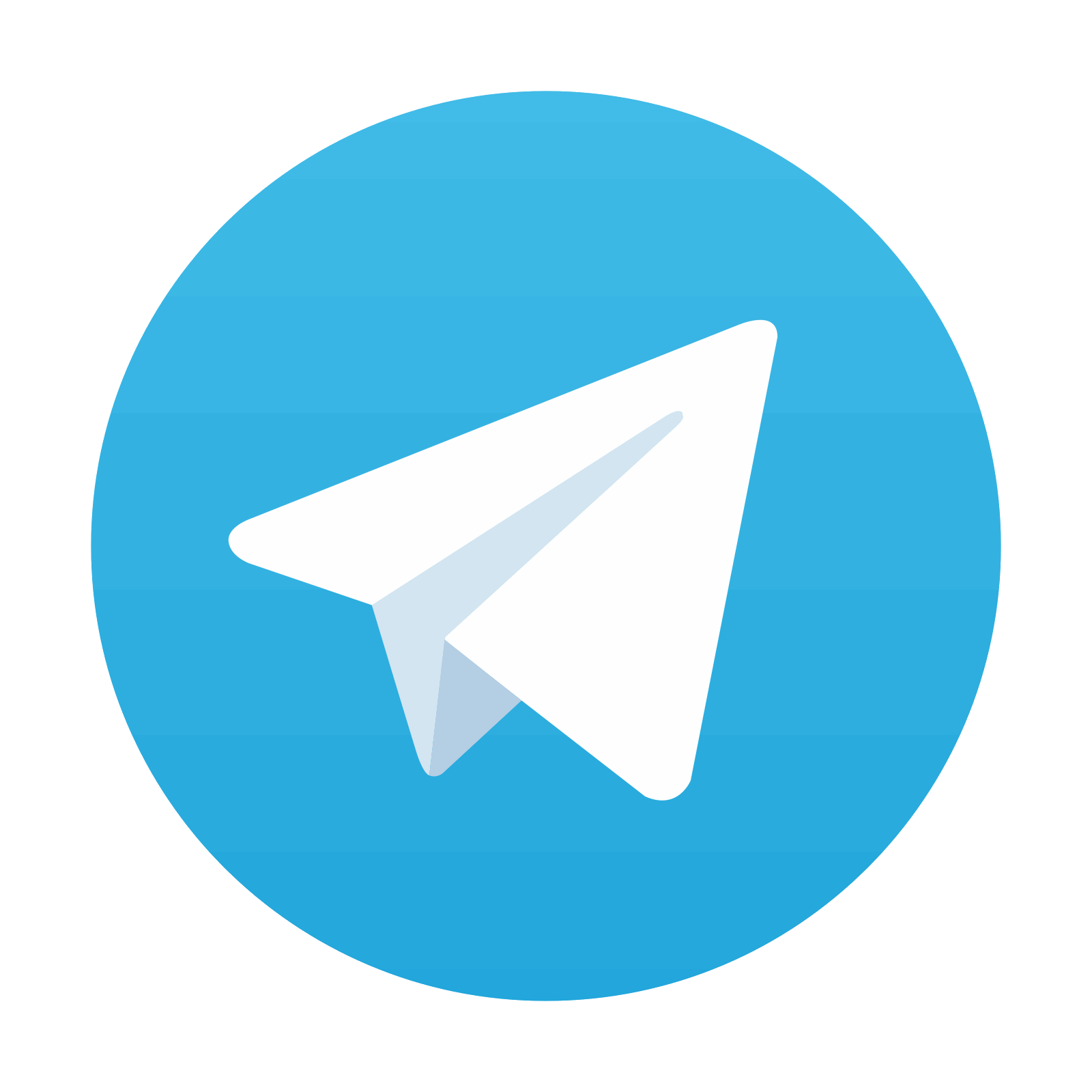
Stay updated, free articles. Join our Telegram channel

Full access? Get Clinical Tree
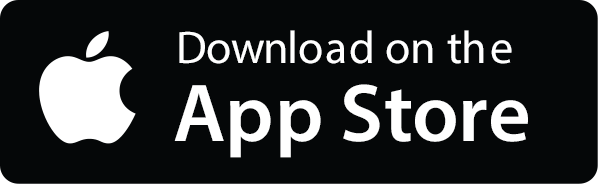
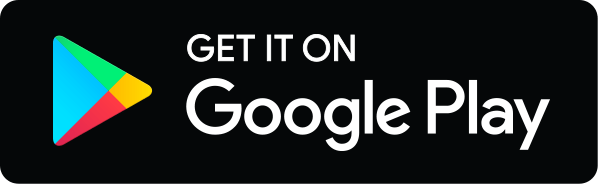