Chapter Outline
Electricity and Electrical Safety 361
Classes of Electromedical Equipment for Electrical Safety 362
Methods to Reduce the Risk of Electrocution 363
Electrosurgery Units 363
Operating Room Fire and Fire Safety 363
Instigation of Surgical Fire 363
Chemical Chain 366
Reported Scenarios 366
Fire Prevention 366
Minimizing Combustible Materials 366
Lasers and Laser Safety 366
Laser 367
Laser Wavelength and the Eye’s Response 368
Laser-Generated Airborne Contaminants 368
Training and Credentialing 369
Radiation and Radiation Safety 369
Radiation Hazards 369
Radiation Prevention 369
Fluoroscopy 369
Radiation-Associated Procedures 369
MRI 370
Electricity and Electrical Safety
Electric current in contact with the human body has direct pathophysiological effects. When electrical current “escapes” from its protective insulation and its intended work pathway, it has potential to cause shock, interference with equipment, or even exploed when combined with flammable/combustible materials present on the body. The most feared of these is electric shock because the ensuing energy delivery to tissues can result in burns, cellular damage, cardiac arrhythmias, and death by electrocution.
In addition to resistant heating of tissues and burning, electrical stimuli can conduct in excitable tissues and result in electrochemical effects. Electrical conductivity will depend on the inherent resistance of individual tissues but can be categorized as follows: high (tendon, bone, fat), intermediate (dry skin), and low (nerve, muscle, blood, mucous membranes).
The severity of electrical injury is dependent on tissue resistance (R), current (I), potential difference (V), current density, current frequency, current pathway, and duration. The thermal energy delivered to the tissues depends on the power dissipated (P), derived from the formula: V × I = I 2 R or V= I × R, more familiarly known as Ohm’s law (also see Chapter 28 ). The body may be considered electrically to be a good conductor (electrolytes) ensconced in insulation (skin, fat). According to Ohm’s law, current flow is inversely proportional to impedance. The main impedance is the resistance of the skin, which is variable depending on the skin type (mucous membranes, sole of foot, etc.) and moisture level (dry skin, sweaty skin, wet skin). In addition, the larger the current or the smaller the area over which it is applied, the higher the current density. However, the applied voltage is related to the whole body electrical impedance because higher voltages (>250 volts) result in a drop in the total body impedance irrespective of contact area and pathway of the current.
The electrical current exerts effects on excitable tissues. These effects are dependent on current, time, and current frequency. The commonly used frequencies (line frequency) in the operating room are 50 Hz (UK, Europe) and 60 Hz (United States) and, ironically, are capable of the greatest risk of excitation and damage to human tissues. Not surprisingly, given its inherent electrical conduction pathways, the excitable tissue of the heart carries the greatest susceptibility to electrical injury. Cardiac arrhythmias and permanent damage may result. Energy conducted through the heart may result in muscle damage to the heart (vertical pathway) or disturbances in the heart’s electrical pathways causing ventricular fibrillation/sudden death (horizontal pathway). Animal studies suggest that a minimum of 100 milliamperes of current applied at 60 Hz may cause ventricular fibrillation. Given this, a very small potential from a stray voltage in the mains can transmit current to the heart causing ventricular fibrillation and electrocution, also known as microshock.
The type of current, alternating (AC) or direct (DC), produces varying effects. Most electromedical devices are powered by AC current for efficiency of power transmission. Alternating current (e.g., power line) consists of current (electron flow) that reverses direction at regular intervals. Direct current (e.g., battery) flows in a single direction. Alternating current carries greater inherent risk of harm than direct current at lower levels of current, and is most dangerous in the 10 to 200 Hz frequency. U.S. current (line frequency) is 60 Hz despite this danger because of its better ability to carry power over long distances than higher frequency current. The current travels from the power station to the substation where it is transformed to line voltage. Current is measured in amperes (A) and represents a flow of 6.24 × 10 ^18 electrons past a specific point in 1 second. Line electric current, typically via a medical equipment source, has the potential to injure the patient and cause electrocution, burns, electrochemical disturbances, and explosion.
The general effects of line supply electrocution (60 Hz) follows:
1 mA—tingling/pain
5 mA—pain
15 mA—tonic muscle contraction/pain
50 mA—respiratory tonic muscle contraction/respiratory arrest
70 to 100 mA—ventricular fibrillation/cardiac arrhythmias/local burns
1000 mA—extensive burning/charring
Direct current tends to produce single muscle spasm, throwing of the victim from the source, blunt mechanical trauma, and heart disturbances. Alternating current induces tetany (continuous muscle contraction), extensive gripping of the power source/conductor by inducing flexion muscle contraction (flexor muscles being stronger than extensor muscles), and local sweating, resulting in lower skin impedance (lowered resistance).
Classes of Electromedical Equipment for Electrical Safety
Class I: This type of equipment affords basic protection and includes household equipment. The electrical device has live and neutral leads, which do not come into contact with one another. Additionally, further protection is offered by insulating the metal casing via an earth wire in the plug to the line supply, thereby allowing the casing to be touched by the user. In the event of a fault in the apparatus where current leaks to the casing, the earth connection grounds the leaking current. Fuses are positioned in the live and neutral supply, and in the UK, a fuse positioned in the live lead of the mains plug also melts, disconnecting the circuit in the event of a fault. However, the user loses protection and becomes susceptible to electric shock if the earth lead is faulty.
Class II: This equipment, termed double-insulated equipment , has “double insulation” because both the apparatus and casing are insulated. There is no need to earth this type of equipment, and the power cable only has live and neutral conductors with a single fuse.
Class III: This type of equipment operates with a power source that does not exceed 24 volts AC or 50 volts DC. The voltage is called safety extra low voltage or SELV. There is still a risk for microshock. Thus this equipment may contain its own internal power source or connect to the mains supply via SELV transformer or an adaptor.
The following equipment categorization is based on the degree of protection conferred according to the maximum permissible leakage current.
Type B: This categorization defines devices in which the earth leakage current is limited to 0.05 mA in class I equipment and 0.1 mA in class II equipment. Type B classification can be applied to classes I, II, and III equipment or one with an internal power source. These devices may be connected to the patient internally or externally, but are not intended for connection to the heart. They may contain defibrillator protection.
Type BF: These devices are similar to Type B equipment, except that the part applied to the patient is isolated from the other parts of the equipment using a floating (isolated) circuit. The allowed current leakage under single fault conditions must not exceed 1.1 times the rated line voltage applied between the earth and the patient. The maximum AC leakage current under normal conditions is 0.1 mA and 0.5 under a single fault. While Type BF is safer than Type B, direct connection to the heart is still not permitted. These devices may have defibrillator protection.
Type CF: This type of equipment may be class I or II and confers the greatest level of protection against electric shock. Type CF equipment is considered safe for direct connection to the heart as is used for ECG leads and pressure transducers. Allowed current leakage is 0.05 mA per electrode for class I and 0.01 mA for class II equipment. Leakage current resulting from these devices will not cause electrocution. Such equipment may have defibrillator protection.
Isolated or floating circuit —A small isolating transformer is contained within the individual item of medical equipment’s internal circuitry. The line circuit is thus earthed, whereas the patient circuit is isolated from the earth and is thus thought of as floating. This safety measure prevents current from flowing between the electrical source and earth.
COELCB (current-operated earth leakage circuit breakers)—These methods of improving patient safety can be connected to an individual item of medical equipment or to the whole operating room. COELCB are also referred to as an “earth trip” or RCCB (residual current circuit breaker). These consist of a transformer with a core that has equivalent numbers of windings of live and neutral wire. A third winding to the coil relays the circuit breaker. The magnetic fields from the two coils cancel one another under normal conditions. However, in the case of a fault, the current generated from the two coils will result in a magnetic field and will cause a current in the third winding “tripping” the circuit breaker. The COELCB are designed to be very sensitive and as little as 30 mA can trip the circuit. These devices are generally less expensive than isolating transformers and similarly decrease the potential for serious shock to the patient.
Electrosurgery Units
Electrosurgery units (ESU), or surgical diathermy, are frequently used in surgery for cutting and coagulation to assist with dissection and to halt bleeding. Surgical diathermy uses current passing through a small amount of tissue to generate the heat needed to carry out these functions. The active electrode consists of high current density with a current passing through a very small area of tissue at the point of contact, as compared with the patient plate, which has low current density with a large conductive area to rapidly conduct away heat to prevent burning. This current density gradient allows for the desired surgical effect. Tissue sensitivity to main frequency electric current decreases at higher frequencies (500,000 to 1,000,000 Hz), allowing the necessary current to pass through the human body and generate the appropriate heat for surgical diathermy without excitation of contractile cells (lowered tissue penetration).
Surgical diathermy frequency (0.4 to 1.5 MHz) is regulated by international standards to certain narrow frequency bands to prevent interference with monitoring devices. A sine waveform for cutting and a modulated waveform for coagulation are used, though a combined waveform (blended mode) is also common. While the risk of electrocution is lowered by the differential impedance provided relative to the current frequency (low impedance to high frequency current of diathermy; high impedance to low frequency current to prevent electrocution), potential burn risk is still a concern and correct lead orientation is critically important to prevent accidental connection of the patient plate to the active terminal.
Two principal types of ESU systems exist: the ground-referenced type and the isolated-generator type. For ground-referenced systems, the electrical current passes through the patient and returns to ground. The grounding is intended to occur through the dispersive plate (pad) placed onto the patient; however, any grounding object can serve as the method of ground return. Given the potential for burns to occur at alternative grounding pathways/sites, many manufacturers no longer rely on ground-referenced ESUs. A safer system incorporates the isolated-generator system where the current return through the dispersive pad to the negative side of an isolation transformer located within the generator. Since the return electrode is not connected or referenced to ground, the current does not return to ground nor does it seek an alternative pathway through other grounded objects (e.g., such as when a conductive material such as metal is in contact with the patient’s skin).
In bipolar diathermy, the current flows between the two forceps tips and a patient plate is not needed. The heat generated at both electrodes is equivalent as is the current density and a good coagulation effect is noted. However, the cutting ability of the bipolar diathermy is less effective.
Use of radio frequency diathermy should be avoided in patients with pacemakers. Potential sequelae include cardiac burns and pacemaker malfunction. In these patients, a bipolar cautery, used in locations remote from the heart, provides a safer alternative.
Operating Room Fire and Fire Safety
Obtaining an accurate estimate of the total number of surgical fires in the United States can be trying due to the lack of central reporting mandates and redundancy in reporting agency systems. Fortunately, operating room fires are a relatively infrequent occurrence despite the nearly 50 to 60 million operations that are carried out in the United States each year. In 2007, the ECRI (Emergency Care Research Institute) estimated 550 to 650 incidents of surgical fire in the United States. Of these, the vast majority was due to electrosurgical equipment usage and lasers. The remainder involves other heat-producing sources such as defibrillators, sparking high-speed equipment, and fiber-optic light sources, among others. An oxygen-rich environment and alcohol-based preps contribute to the instigation of a surgical fire. While the majority of fires do not result in injury, nearly 10 to 15 cases are devastating, resulting in serious burn injury to the patient and resultant disfigurement.
The traditional model for fire initiation involves three components, termed the “fire triangle,” and includes a heat source, a combustible material, and an oxidizer. When a fourth component of the chemical chain is added to create the “fire tetrahedron,” an exothermic reaction develops whereby a burning metal reacts with water.
In the operating theater, surgeons, nurses, and anesthesiologists synchronize their efforts to facilitate the successful execution of a safe operation. To accomplish this goal, an intricate interplay of machinery, surgical devices, and chemical components may be involved. In general, surgeons will control potential heat sources, surgical nurses police combustible materials, and anesthesiologists will regulate the oxidizer(s). Therefore a team-based approach with patient safety in mind is critical to prevent fire ignition in the operating room.
Instigation of Surgical Fire
Heat Source
There are numerous sources of heat in the operating room. Surgeons serve as the main source of heat in the fire triangle, usually due to the incorporation of surgical devices or specialized equipment needed for the execution of the particular surgery.
ESU (Electrosurgery Units)
Electrosurgery units, or diathermy, can serve as a source of ignition and fires, and explosion may result; particular attention should be paid in the setting of bowel gas or cleansing solutions. ESUs beget potential hazards in the operating room including fire, burn, shock, and smoke plume. (Burn and shock are discussed earlier in the “Electricity” section.)
Laparoscopic Surgery
In a similar manner to ESU, laparoscopic surgery is associated with several special electrical hazards. The primary ones are insulation failure, direct coupling, and capacitative coupling. Current leakage to the patient may occur from the laparoscopic instrument, resulting in either laparoscopic diathermy malfunction or unintended tissue damage. Stray current may result from insulation break in the diathermy applicator, direct coupling between the active electrode and other organs or metal instruments out of the field of view, or capacitative coupling through intact insulation. Capacitative coupling between a laparoscopic cannula and electrically isolated adjacent tissues can occur, which may be aggravated by smaller cannulas that yield greater capacitance and larger stray current. Insulated instruments and bipolar coagulation help guard against these hazards.
Surgical Plume
Each year, an estimated 500,000 operating room personnel, including surgeons, nurses, anesthesiologists, and surgical technologists, are exposed to laser/electrosurgical smoke. Surgical plumes have contents similar to other smoke plumes, including carbon monoxide, polyaromatic hydrocarbons, and a variety of trace toxic gases. These gases can produce upper respiratory irritation and have in – vitro mutagenic potential. Although no documented transmission of infectious disease through surgical smoke has been reported, the potential for generating infectious viral fragments, particularly following treatment of venereal warts, may exist. The Occupational Safety and Health Administration (OSHA) recommends local smoke evacuation systems, which may improve the quality of the operating field.
Defibrillators
Operating room fires have been reported in defibrillator usage. The source of heat during a defibrillation attempt is typically a spark or electric arc. When there is a poor electrode-chest wall interface, an electric arc may occur during the countershock. A poor interface can also result from paddles that are too large for the chest wall or paddles that extend beyond the conducting electrode cream or conducting gel pads. Electric arcing can also occur when paddles are placed too close to an electrocardiographic electrode. Excessive electrode cream bridges from one paddle to another can also increase the risk for fire, as does a saline-soaked pad placed between the paddle and the patient.
Pressure Regulator
When gas is released from a high pressure system to a low pressure system, recompression of the gas can cause an increase of local temperature. When oxygen is in the vicinity, fire or explosion can occur. Aluminum regulators used with high pressure oxygen systems can rarely cause catastrophic combustion in normal usage. Therefore, routine checks of the regulator and updates on product safety from the manufacturers are recommended.
Electrical Faults
In the 1990s, several fires were reported when power plugs were being pushed in or pulled out of the outlet. These were likely due to a “single fault condition”—where a single means for protection against hazard is defective (e.g., a short circuit between live and applied parts). Today, anesthesia machines and electric surgical equipment can still short circuit and cause operating room fires.
Light Sources
Argon beam coagulators, intense surgical light sources, and endoscopic light systems from a fiber optic system can all serve as sources for heat. Light systems used in the surgical field often need to provide high intensity light through a small diameter device. As the required power densities for the light source are high, they are at risk for overheating. In an environment with a high concentration of oxygen (i.e., during anesthesia induction and emergence), the high power light can serve as a heat source for operating room fires and explosions. To prevent fire, surgical staffs should try to inactivate the power supply whenever possible.
Static Electricity and Sparks
Although operating room static electricity is relatively low in quantity, enough sparking energy can be created to ignite flammable vapors. To prevent unexpected sparks, the floors of operating rooms should be covered with an approved conductive (antistatic) material. Floor conductivity should be tested regularly and records of testing should be maintained by the hospital engineering department. In addition, electrical equipment should be approved by the hospital engineering department before use in the operating room. All mobile electrical equipment should make electrical contact with the operating room floor. Regular checks are needed to ensure proper operation. Likewise, conductive clothing should be worn, and conductive footwear should be required.
Combustible Materials
Combustible material serves as the “fuel” in the fire triangle model. These are any materials that can be burned; they can be in gas, liquid, or solid state.
Endotracheal (ET) Tube
The specific material composition of an ET tube (PVC, red rubber, metallic) determines the risk and characteristics of a tracheal fire. ET tubes are usually penetrated by laser or surgical equipment before ignition. A characteristic of ET tube fire is that the fire usually starts in the inner rim of the penetrated area then spreads along the direction of oxidizing gas.
Polyvinylchloride Tube (PVC Tube)
PVC tubes are products of petroleum-based extractions. They are combustible in an oxidizer-rich environment, usually with an ignition by a laser. Once ignited, PVC tubes can produce a torchlike fire. Increasing positive end-expiratory pressure (PEEP) is thought to decrease the risk of PVC tube fire during laser surgery. Blood, saliva, and mucus covered PVC tubes have a higher risk for fire. Furthermore, rubber-based ET tube may have an advantage over PVC tubes during extubation. Other components of the fire triangle should be avoided when possible (i.e., nitrous oxide or high concentration of oxygen gas). Saline-filled cuff ET tubes may further reduce the risk for fire in the airways. Metallic tapes such as aluminum-foil tape and copper-foil tape have been developed to protect combustible endotracheal tubes from the CO 2 laser. Comparatively, the Laser-Guard-wrapped PVC tracheal tube and the Tyco Healthcare/Nellcor Laser-Flex tracheal tube are less reflective of incident CO 2 or KTP laser surgery laser radiation than the copper- or aluminum foil-wrapped red rubber tracheal tubes. Laser-Guard helps protect the shafts of combustible PVC endotracheal tubes from direct, high power, continuous CO 2 laser radiation.
Red Rubber (RR) Endotracheal Tubes
Sterile disposable PVC ET tubes are now routinely used in surgery. Previously, red rubber (Rusch-Germany) tracheal tubes were used. These tubes were sterilized for reuse, which carried a small a risk of infection. Similar to PVC tubing, RR tubes also pose a risk for operating room fires if penetrated by laser energy in an oxidizer-rich environment. Use of a rubber ET tube may have an advantage over plastic PVC ET tube, with a potentially more rapid extubation in the case of an ET tube fire.
Laser-Resistant Tubes
An appropriate laser-resistant tracheal tube should be used for the procedure (e.g., carbon dioxide [CO 2 ], neodymium-doped yttrium aluminium garnet [Nd:YAG], Ar, erbium-doped yttrium aluminium garnet [Er:YAG], potassium titanyl phosphate [KTP]). Laser-resistant tubes provide improved safety; PVC and RR tubes can readily ignite in an oxygen-rich environment with continuous laser energy supported by nitrous oxide combustion.
The tracheal cuff of the laser tube should be filled with saline and colored with an indicator dye such as methylene blue. Before laser activation, the surgeon should indicate to the anesthesiologist that laser activation is about to occur so that delivered oxygen content can be reduced to the minimum acceptable, nitrous oxide use can be terminated, and ample time can transpire (a few minutes) for reduction of the oxidizer-rich atmosphere. Adding PEEP during CO 2 laser operations on the airway in which laser-resistant tracheal tubes with PVC components are used may decrease the incidence of fire.
Prep Agents
Many prepping agents and certain ointments are flammable. In particular, alcohol-based prepping agents are extremely flammable and vapors from these agents can ignite from a laser or ESU. One should avoid dripping or pooling of residual alcohol-based preps, especially at the umbilicus and suprasternal notch of the patient because vapors trapped under drapes or residual wet draping may ignite with heat or sparks. When prepping with alcohol-based prep, proper technique should be employed to ensure proper drying time and limited exposure of any volatile solution in the operating room. Also, surgical petroleum-based ointment, especially if applied in thin layers, can absorb considerable heat and vaporize. These vapors, after mixing with oxygen, serve as a potential ignitable source. To the contrary, water-based lubricants (K-Y jelly) do not burn and can be used to coat hair as a fire retardant. Similarly, water-based prepping agents (Betadine, Pharmaseal) should be used when possible.
Patients
A patient’s body hair, eyelashes, mustache, and eyebrows are all combustible. Water-based lubricant can reduce the fire hazards.
Intestinal Gas
Methane, hydrogen sulfide, and sulfur gas in the GI tract have all been reported sources for combustible gas within the patient’s body. Care should be taken to reduce the intestinal gases as much as possible by proper preoperative bowel preparation. Oxygen may also diffuse into the GI tract and become a combustible firehazard.
Surgical Products
Surgical drapes, gowns, masks, shoe covers, mattresses, pillows, and blankets can serve as fuels for operating room fires. An oxygen-rich environment may override the fire-resistant properties of surgical products. Sponges, gauze, and ace bandages can also be fuels for operating room fires. However, moistening sponges and gauze with saline reduces the chance for fire. Disposable drapes may be troublesome when caught on fire because they are water repellant. One should consider that fire-resistant drapes are not fireproof.
Gauze, especially with blood, can be very combustible under an oxygen-rich environment.
Equipment/Supplies
Other equipment that may become fuel in the operating room include anesthesia components, flexible endoscopes, coverings of fiberoptic cables, gloves, blood pressure and tourniquet cuffs, stethoscope tubing, smoke evacuator hoses, instrument cabinets, disposable packaging material (plastics), and warming blankets (Bair Huggers).
Oxidizing Media
Anesthetic Gases
Older anesthetic gases, such as cyclopropane, ethylene, acetylene, and nonhalogenated ethers (i.e., diethyl ether), are flammable; as are some halogenated volatile agents, such as ethyl chloride and fluoxene. While flammable gases are employed in some cases, most of the halogenated gases currently in use in the United States are not considered flammable. Carbon dioxide, halothane, enflurane, isoflurane, sevoflurane, and desflurane are not flammable, nor is atmospheric pressure nitrous oxide. Mixing these gases with oxygen can alter the mixture’s flammability, making fire possible in a clinical setting. Therefore, a routine check of leakage of gas tanks is recommended. Flammable anesthetics should be kept in a National Fire Protection Association-approved central location that is fire-resistant and vented to the outside.
Anesthetic-Proof Equipment
Anesthetic-proof (AP) equipment standards are based on the ignition energy required to ignite the most flammable mixture of air and ether (based on 5 to 25 cm of gas escape from the respiratory system). The temperature should not exceed 200° C.
Anesthetic-Proof Equipment Category G
APG standards are more stringent and based on ignition energy (energy level less than 1 mJ) for 5 cm of gas escape from a respiratory system of the most flammable mixture of air and ether. Temperature should not exceed 90° C.
Oxygen Supply Fire and Nitrous Oxide
Since the introduction of oxygen supplies, the fire incidence in the operating room due to anesthesia gas has increased. Nitrous oxide is a more reactive oxidant and supports combustion more intensely than oxygen. Nitrous oxide should therefore not be used during laser endoscopic surgery.
Faulty assembly of the oxygen regulator can cause serious fire. When oxygen is released from a high pressure to a lower pressure system, the temperature can increase dramatically. With 100% oxygen, this temperature spike can cause fire within the tubing, the regulator seats and seals, or even the metal tank itself. Teflon tape or other hydrocarbon contaminants may potentially cause sparking while under high pressure release from the oxygen tank, inducing a fire. Oxygen-content-lowering devices used in the operating room can reduce the incidence or prevent this type of fire.
Chemical Chain
When a fourth component of the chemical chain is added to create the “fire tetrahedron,” an exothermic reaction develops. For example, desiccated Baralyme (CO 2 absorbent) or soda lime can react exothermically with sevoflurane to produce carbon monoxide and flammable organic compounds, such as methanol and formaldehyde. The heat produced accelerates the reaction speed, and at very high temperatures, combustion of the flammable metabolites may occur. Temperatures can reach above 300° C and canister melting and water in the circuit may be observed.
Fire Prevention
Some important safety measures include keeping the Bovie tip in its insulated holster when not in use. Turn down the power of high intensity light sources whenever not in use. Use only protected endotracheal tubes when operating near the trachea. Use air or air and oxygen mixtures in anesthetic gases. Avoid tenting of surgical drapes to form oxygen-enriched areas (e.g., near a nasal cannula). Decrease oxygen content to lowest acceptable levels. Use water-soluble (nonflammable) substances to cover hair and other flammable parts of the body. Use fire-retardant drapes.
Minimizing Combustible Materials
Supraglottic jet ventilation (SJV) is a technique in which adequate oxygenation and ventilation is achieved without using a combustible endotracheal tube during laser surgery. However, there has been a case report of accidental fire caused by other combustible material in the surgical field.
Chemical Chain
As an alternative to Baralyme, Amsorb (carbon dioxide absorbent) can be used. Amsorb remains hydrated and changes color when dehydrated; it does not contain the strong bases necessary to produce carbon monoxide in clinically significant amounts or react exothermically with sevoflurane. Routine changing of carbon dioxide absorbent may also reduce the risk of an injurious exothermic reaction.
Fire Safety Plan
A fire safety plan should be adopted and implemented. If a fire is recognized, all unnecessary operating room personnel should leave and notify appropriate authorities, electrocautery should be shut off, and the electrode removed from the area. A fire extinguisher can be used to put out fires in the immediate setting, and, if possible, all oxygen and nitrous oxide should be shut off and the patient extubated as quickly as possible. Awareness of surgical suite fire is the key to prevention.
A surgical suite primer has been published; appropriate training in fire hazard prevention and proper facility operation and maintenance is critical to ensuring patient and operating room personnel safety.
- ▪
Use separate collection containers for glass, empty ether cans, aerosol cans, disposables, etc., that are not to be incinerated.
- ▪
Instruct personnel to report defective equipment.
- ▪
Post warning signs where necessary and enforce proper work practices.
- ▪
Discuss safe work practices and health hazards with each new worker as part of orientation. This training should be reviewed periodically.
Guidelines for operating room fire safety and hazard prevention are available and should be used accordingly.
Lasers and Laser Safety
The increasing popularity of laser use in the operating room demands understanding of its mechanisms, energy delivery, and potential hazards to operating room staff. Laser is an acronym for “Light Amplification by Stimulated Emission of Radiation.” Laser energy generates a one wavelength, one color (monochromatic) pure beam of light.
Laser
Laser is a modern technology that converts electric energy into concentrated light energy. Light energy is concentrated by storing it in an enclosed reflective container before release. A laser uses collimated, coherent, monochromatic electromagnetic radiation to achieve the intended surgical goal. Lasers have the inherent ability to cut, coagulate, and/or vaporize tissues, rendering surgery near bloodless.
The power of the energy delivery attributed to a laser is usually described in “watts,” or energy (in joules) per unit time of exposure (in seconds). Laser power density is defined as the laser’s power per area (in square centimeters). The wavelength of the laser is specified by the medium used for lasing, which may be a solid, liquid, or gas. Stable atoms in the “ground state” of the lasing medium becomes excited to a higher energy state by the photon pumping source and then spontaneously emit a photon of energy as it returns to the ground state, usually in the form of light or radiofrequency energy. Einstein first described the process of adding an additional photon of energy at the correct wavelength to the excited atom to promote “stimulated emission” of two photons of energy, the basis of modern laser technology. As many photons escape back into the lasing medium, a chain reaction of amplification ensues and an intense source of light energy can be generated.
Components of Lasers
Lasers used in clinical settings usually include four components in the apparatus: a delivery system to direct the output of the laser, a power supply with laser control and calibration functions, mechanical housing with interlocks, and associated liquids, solids, or gases as the media for laser operation.
Laser Types
While there are numerous types of lasers, only a dozen or fewer are found in everyday surgical use. The clinical effect of the light energy on human tissues is dependent on the laser’s intensity and the frequency of light used. At lower intensities, the laser stimulates cells. However, at higher intensities, cellular activity attenuates, tissue temperature rises, and protein denaturation and photocoagulation occur. To control the thermal energy delivered to tissue, laser pulsing can be carried out to allow for heat dissipation between bursts. “Q switching” refers to the process of storing laser light aliquots for release in bursts of higher energy and shorter duration. With this process, mechanical damage may predominate over thermal damage because of induced vibration. Numerous lasers are available for medical use, the most familiar being CO 2 (carbon dioxide) and Nd-Yag (neodymium: yttrium-aluminum garnet). The carbon dioxide laser (10.6 μm) causes rapid vaporization of intracellular water (absorbs water within 1 mm depth) with energy in the far infrared spectrum; it is mainly used as a vaporizer and bloodless cutter. The Nd-Yag laser uses a solid lasing medium and penetrates at a 3 to 5 mm depth. It produces energy in the near infrared spectrum and maximally penetrates hemoglobin, melanin, and water.
Laser Hazard
Operating room laser usage always carries a risk of accidental injury to OR personnel and patients. Therefore appropriate OR signage ( Figure 29–1 ) should be displayed. A laser source, when not being used, can be accidentally activated (e.g., by stepping on a laser’s foot-controlled operating switch). In the setting of potentially flammable surgical drapes and clothing, an accidentally triggered laser can introduce a hazard in the operating room if in the line of clothing, directed toward reflective metal sources, or camouflaged under ignitable surgical drapes. To reduce the prospect of such disastrous occurrences, a surgical laser source should routinely be placed on standby mode when not being actively engaged.

The most common cause of laser-induced tissue damage in humans is thermal. Heat from the laser beam causes biochemical injury by denaturing proteins in the affected body area. The nondivergence of the beam and long range of laser light leaves little protection even when remote from the laser source. A particularly vulnerable portion of the body to the laser’s thermal damage is the ocular region ( Figure 29–2 ). The specific location of eye injury for a given laser depends upon its output wavelength. Laser light in the visible and near infrared spectrum—400 to 1400 nm—may induce damage to the retina, while wavelengths outside this region (e.g., ultraviolet and far infrared spectrum) are absorbed by the anterior segment of the eye, damaging the cornea and/or the lens. Laser light transmitted to the eye can result in blind spots or even total blindness. The extent of the damage depends on the total energy the body part has suffered under the laser. Therefore protective eyewear in the operating room in the form of goggles, glasses, and shields is crucial to ensure protection against ocular injury, and must be worn at all times during laser surgery. Laser safety eyewear (LSE) is designed to reduce the amount of incident light of specific wavelength(s) to safe levels, while transmitting sufficient light for good vision. Each kind of laser requires a specific type of protective eyewear, and factors that must be considered when selecting LSE include laser wavelength and peak irradiance, optical density, visual transmittance, field of view, effects on color vision, absence of irreversible bleaching of the filter, comfort, and impact resistance.

Laser Classification
Class 1 —A class 1 laser system is considered to be incapable of producing damaging radiation levels during normal operation. There are no control measures or other forms of surveillance for class 1 lasers. Some class 1 lasers do emit very weak, nonhazardous beams, but these class 1 laser systems usually have “embedded” higher power lasers, which are only accessible if the interlock safety systems are deliberately opened.
Class 1M —A class 1M laser system is considered to be incapable of producing hazardous exposure conditions during normal operation unless the beam is viewed with an optical instrument, such as an eye loupe or a telescope. Class 1M lasers are also free of any control measures and other forms of surveillance.
Class 2 —A class 2 laser system emits in the visible portion of the spectrum (400 to 700 nm) with power up to 1 mW. The means of eye protection is normally done by the aversion responses, which include closure of the eyelid, eye movement, pupillary constriction, or movement of the head to avoid the exposure. The aversion response to a bright visible laser source is assumed to limit the exposure of the retina to 0.25 seconds or less (blink reflex time). Class 2M is potentially hazardous if viewed with certain optical aids.
Class 3R —A class 3R laser system is potentially hazardous under direct or specular reflection viewing conditions if the eye is appropriately focused and stable, but the probability of an actual injury is small. This laser is unlikely to pose either a fire hazard or diffuse reflection hazard.
Note: an old classification of class 3A should be considered equivalent to class 3R.
Class 3B —A class 3B laser system may be hazardous under direct and specular viewing conditions; class 3B laser is normally not a diffuse reflection or fire hazard thanks to its required laser power limitation: 500 mW for continuous laser sources and 30 mJ for pulse laser sources.
Class 4 —A class 4 laser system is a hazard to the eye and skin from the direct beam. It includes any laser systems that emit a higher power than class 3B laser systems. A class 4 laser system poses a diffuse reflection or fire hazard, and may also produce laser-generated airborne contaminants and hazardous plasma radiation.
Nearly all surgical treatment lasers are class 4 because they are designed to deliver laser radiation for the purpose of altering biological tissue. The following section provides information regarding the use of lasers.
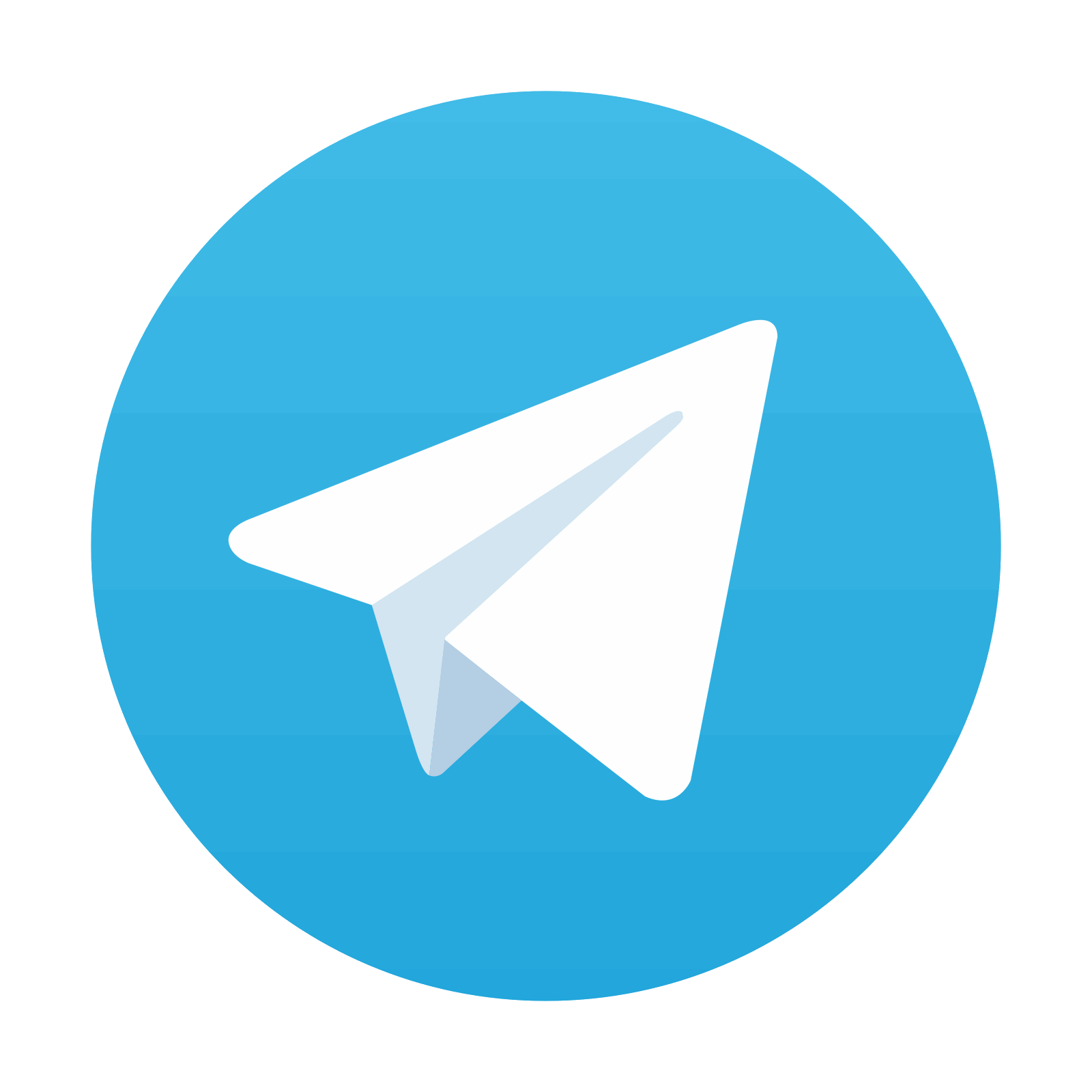
Stay updated, free articles. Join our Telegram channel

Full access? Get Clinical Tree
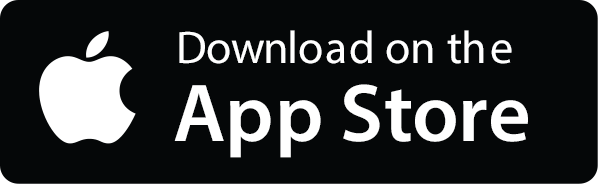
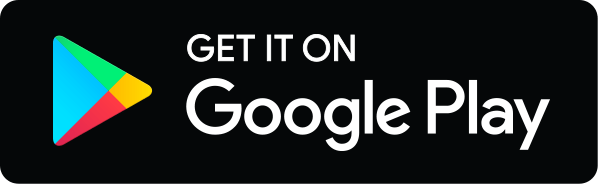