KEY POINTS
Respiratory, neurologic, metabolic, thoracic, and cardiac emergencies constitute life-threatening complications in patients with malignancies. These oncologic emergencies often result from the cancer itself and/or from treatment of the cancer.
Increased activation of the coagulation system, administration of thrombogenic chemotherapy regimens, and placement of intravascular venous catheters place cancer patients at higher risk for pulmonary embolism and hemodynamic instability.
Neurologic emergencies in cancer patients include status epilepticus, malignant spinal cord compression, and intracranial hemorrhage.
Radiation therapy and corticosteroids are the mainstays of treatment of malignant spinal cord compression.
Malignancy-associated hypercalcemia (MAH) can be divided into humoral, osteolytic, and calcitriol-associated hypercalcemia. Bisphosphonates are the most efficient and recommended treatment for MAH.
Tumor lysis syndrome is associated with hyperuricemia, hyperphosphatemia, hypocalcemia, and hyperkalemia, which if left untreated can lead to arrhythmias and death. Treatment includes aggressive hydration, specific treatment of individual metabolic derangements, allopurinol or rasburicase, and hemodialysis for severe hyperphosphatemia and symptomatic hypocalcemia.
Leukapheresis is usually initiated for the treatment of leukostasis associated with acute myelogenous leukemia if the WBC count >50,000/mm3 and in acute lymphoblastic leukemia if the WBC count is >250,000/mm3.
Ninety percent of malignant causes of superior vena cava syndrome (SCVS) are due to lung cancer and lymphoma. Patients presenting with cerebral edema and airway compromise due to SVCS should be treated urgently and considered for SVC stenting.
Treatment for cardiac tamponade requires emergent drainage by either pericardiocentesis or pericardial window.
INTRODUCTION
Significant advances in cancer care and preventive strategies have decreased the incidence of the classic oncologic emergencies (eg, superior vena cava syndrome, tumor lysis syndrome, and malignant spinal cord compression) that previously necessitated admission to the intensive care unit (ICU). Currently, cancer patients in the ICU are more frequently admitted for respiratory and cardiac failure, life-threatening sepsis, metabolic complications, and hemorrhagic and thrombotic disorders. Similar to the classic oncologic emergencies, these syndromes often result from the cancer itself and/or from treatment of the cancer. This chapter will discuss the epidemiology, pathophysiology, clinical presentation, diagnosis, and management of the common and classic oncologic emergencies that ICU clinicians will encounter in their practice. These include respiratory, neurologic, metabolic, thoracic, and cardiac emergencies.
RESPIRATORY EMERGENCIES
Pulmonary embolism (PE) leads to 300,000 deaths a year and is the second most common cause of death in cancer patients.1-3 Increased activation of the coagulation system, administration of thrombogenic chemotherapy regimens, and placement of intravascular venous catheters place oncological patients at higher risk for thromboembolic disease.3 Early recognition and treatment of PE is essential due to its high mortality when left untreated.
Patients with PE should be admitted to the ICU when there is significant respiratory compromise, presence of hemodynamic instability, right-sided heart failure, or high risk of cardiovascular collapse. Right-sided heart failure in the setting of massive PE is associated with a mortality rate ranging from 25% to 58%.1,4 The acute elevation in pulmonary artery pressures after massive PE causes severe strain to the right ventricle (RV), myocardial dysfunction, and ventricular failure.5 Moreover, increased right-sided pressures cause the septum to shift into the left ventricle (LV), decreasing end-diastolic volume, worsening cardiac output, and resulting in cardiogenic shock.1,5
In the ICU setting, early diagnosis and treatment of PE are essential. Computed tomography angiography is the most commonly used test for diagnosis and can also be used for risk stratification of PE. Studies suggest that an RV/LV ratio greater than 1 is suggestive of RV dysfunction and can be associated with increased mortality.6 Echocardiography should also be a part of routine evaluation of ICU patients who are admitted with PE. Patients with echocardiographic signs of RV strain (Fig. 93-1) have in-hospital mortality rate of 10% to 53% even without clinical signs of right-sided heart failure.4,7,8 The elevation of cardiac markers such as troponins, B-type natriuretic peptide (BNP), and pro-BNP has also been associated with increased mortality in patients with PE.9,10
Treatment of PE should be based on risk stratification (Fig. 93-2).10,11 Many modalities of treatment exist for PE, and anticoagulation with unfractionated heparin, low-molecular-weight heparin, or fondaparinux remains the standard initial therapy. A recent study showed that rivaroxaban, a new oral factor Xa inhibitor, was as effective as standard anticoagulation for patients with symptomatic PE; however, since few patients in this study were admitted to the ICU, the utility of this agent in this setting remains to be determined.12 Other treatment modalities such as thrombolysis, surgical embolectomy, and catheter devices for clot removal are used occasionally as rescue therapy, and their exact indications continue to be under evaluation.
Thrombolytic agents have been used since the late 1960s for the treatment of PE. The American College of Chest Physicians recommends the use of thrombolytics for all patients with PE accompanied by hemodynamic instability.11 Early use of thrombolytics decreases pulmonary artery pressures and leads to rapid recovery of ventricular function.3,13,14 In a meta-analysis comparing systemic anticoagulation with heparin versus thrombolytics in hemodynamically unstable patients, patients treated with thrombolytics had lower recurrence of thromboembolic events and decreased mortality.15 Thus, in the presence of hemodynamic compromise, thrombolytics are the first-line treatment if there is no contraindication to their use.10,11,13 On the contrary, the role of thrombolytics in patients with submassive PE (hemodynamically stable patients with early signs of RV dysfunction and elevated pulmonary artery pressures) is not clear. One study showed that patients with submassive PE who were treated with thrombolytics and anticoagulation had a less complicated hospital course and no higher risk of bleeding, when compared to those treated with heparin and placebo.16 However, there was no difference in overall mortality between the two groups.16 Because of these findings, consensus guidelines have a grade 2B recommendation for thrombolytic use in submassive PE.11 A prospective, multicenter, randomized double-blind RCT, called the Pulmonary Embolism THrOmbolysis (PEITHO) trial noted reduced hemodynamic decompensation, albeit at a price of increased major hemorrhage and stroke. When considering thrombolysis, and even anticoagulation, it is important to discuss with the patient and their surrogate decision makers the risks of these treatments. Although bleeding complications are rare, they may carry substantial morbidity and even mortality in some patients.
The use of catheter-directed thrombectomy (with or without local thrombolytics) has increased significantly in specialized centers, despite the absence of well-designed trials demonstrating improvements in outcomes. Thrombectomy is performed by clot fragmentation, aspiration (directly performed by the tip of the catheter), or rheolytic therapy (Venturi effect by speed jet injection of saline which also lyses the clot).17 Suitable candidates are patients in whom thrombolysis or surgery is contraindicated, and as a rescue therapy for those who do not respond to initial thrombolytic therapy.17 Local injection of thrombolytics via catheter insertion has increased the efficacy of clot fragmentation with no significantly higher risk of bleeding18; however, there are no studies demonstrating outcomes benefits. Accordingly, this strategy cannot be recommended as standard therapy. In a recent meta-analysis, the rate of survival and success of treatment was similar for systemic thrombolysis and catheter-related therapy; major complications were <3%.19 Caution is important during catheter-directed therapy as these catheters were initially developed for peripheral and coronary arteries and can cause vascular rupture, especially if targeting peripheral vessels.20 Other complications, even though infrequent, include arrhythmias, embolization, and bleeding from the site of insertion.19,20
Surgical embolectomy with cardiopulmonary bypass is another treatment option for massive PE. The procedure is indicated in patients who have contraindications for thrombolysis, as a rescue therapy for thrombolytics, or when there is evidence of intraventricular clots.1 Early studies showed a mortality rate of 28% after surgical embolectomy; however, with current techniques in selected patients, the mortality has been reduced to 6% to 18% in specialized centers.1,21,22 Gulba et al compared the efficacy of surgical embolectomy with medical treatment of PE. These investigators found a slightly better outcome with surgical embolectomy than with medical management (33% vs 23% mortality).23 However, the study lacked statistical power as it included only 37 patients, and had no adequately matched control groups.23 For all patients being considered for surgical embolectomy, the overall prognosis and functional status should be adequate.
The use of vena cava filters is reserved for patients who have contraindications to anticoagulant therapy such as active bleeding or immediately following major surgery or major trauma.10
Massive hemoptysis, defined as expectoration of 300 to 600 mL of blood in 24 hours, accounts for less than 5% of hemoptysis cases and carries a mortality greater than 50%.24 Common causes of hemoptysis are (1) infections (mainly aspergillomas and tuberculosis); (2) pulmonary (bronchiectasis, diffuse alveolar hemorrhage); (3) malignancy (primary and metastatic); (4) vascular (aneurysms and arteriovenous malformations); (5) vasculitis (Granulomatosis with polyangiitis [formerly known as Wegener’s granulomatosis] and Goodpasture syndrome); and (6) trauma or iatrogenic (bronchoscopy, biopsy, and catheter-induced pulmonary artery rupture).25,26 Cancer patients account for 30% of massive hemoptysis cases, and about 10% of patients with lung cancer develop hemoptysis during the course of their disease.27 In the past, the mortality of malignancy-related hemoptysis was up to 90% in case reports compared to other causes (28%-50%).25,27 However, recent studies have shown that the efficacy of treatment for hemoptysis in the oncological population is comparable to all other patients and mortality has been reduced significantly.27,28
Ninety percent of pulmonary hemorrhages originate from the bronchial arteries.29 Bronchial arteries derive from the aorta at the level of T5 and T6, and the anatomy of these branches can vary from patient to patient. Thus, anatomical classifications of the bronchial artery anatomy have been described to facilitate diagnosis and embolization by interventional radiologists.24,30 In 5% of cases, bleeding can come from nonbronchial systemic arteries.24,30 In these cases, the bleeding vessels identified are transpleural collateral vessels from the subclavian, axillary, and internal mammary arteries, which form after chronic inflammation.30 Bleeding from the pulmonary artery (PA) occurs in less than 5% of cases.31 The most common cause for PA bleed is Rasmussen aneurysm, a pseudoaneurysm that forms secondary to chronic inflammation.24
In the presence of massive hemoptysis, early protection of the airway, resuscitation, and reversal of coagulopathy are essential. After stabilization of the patient, the cause and localization of the site of bleed should be established. Chest x-ray is an adequate initial tool for diagnosis due to its easy availability. Although the chest x-ray can help localize the bleed and show other parenchymal abnormalities in 30% to 60% of cases, approximately 20% to 30% of chest x-rays are negative in patients with hemoptysis.30,32 In addition, the diagnostic yield of chest x-rays to localize the anatomic source of bleeding also decreases in the setting of bilateral lung compromise due to aspiration.32
Contrast computed tomography (CT) is superior to chest x-ray, as it can localize the site of bleed in about 70% to 100% of cases.30,32 Initial CT evaluation, especially multidetector CT, can give radiological clues if the bleed originates from bronchial, nonbronchial systemic arteries or the PA.31 Extrapulmonary causes that will require emergent surgery, such as false aortic aneurysms and aortobronchial fistulas, can also be detected on initial CT scans.31,33
In massive hemoptysis, bronchoscopy has a diagnostic yield of 61% to 93%, but its role is still debated by some experts.28,29,34 Use of rigid bronchoscopy has been widely supported as it facilitates airway patency, ventilation, and allows better clot evacuation and visualization of the airways.33 Despite these advantages, only <6% of pulmonologists in the United States have adequate training in the use of rigid bronchoscopy.33 Flexible bronchoscopy, because of its availability and possibility of bedside use, makes it an attractive diagnostic tool. Flexible bronchoscopy allows better access to all segments; however, if the bleeding is massive, visualization may not be possible.27,28 Even though some believe that performing bronchoscopy only delays ultimate treatment, recent studies have shown successful stenting and ballooning of airways in patients with hemoptysis.35,36 Use of bronchoscopic instillation of epinephrine, cold saline, vasoactive solutions, and fibrin has been described in literature but the efficacy of these strategies in massive hemoptysis is limited and unreliable.24,33
More than three decades ago, the initial management of massive hemoptysis was observant and mortality was >90%. Surgical intervention was later integrated as an important part of the management of hemoptysis.33 Surgery is the definitive treatment for patients with massive hemoptysis due to aspergillomas, early malignancy, arteriovenous malformations, and thoracic aneurysms.25,26 Mortality of these procedures is usually 7% to 18.2%; however, when the interventions are emergent, the mortality rate increases to 40%.25,26 Because massive hemoptysis requires emergent intervention, less invasive modalities of treatment, such as embolization, have been developed.
Bronchial artery embolization was first described in 1974.32 Arterial access is usually femoral, and an aortogram is performed for adequate mapping of all arteries.32 Angiographic signs suggestive of a source of bleeding include hypertrophied and enlarged arteries, aneurysms, shunting from the bronchial artery to the pulmonary vein or artery, and active extravasation.30,32 While most of the bleeds originate from the bronchial arteries, it is important to have adequate knowledge of the anatomy and review any possible collateral vessels as a possible source of bleed. Recurrence of bleed after embolization ranges from 2% to 25%.27,29,34 Van den Heuvel et al described a higher mortality in patients with recurrence and identified several risk factors: (1) residual mild bleed after first week of embolization; (2) blood transfusion before the procedure; and (3) aspergilloma as the underlying etiology.37 Early recurrence, considered to be within the first 6 months after intervention, is secondary to incomplete embolization, or incomplete search of other bleeding vessels.24,29 Late rebleed is secondary to recanalization of the previous bleeding vessel or progression of the underlying disease.24,29 Multiple studies have shown success rates of over 90% after embolization, with rebleeding occurring in only 10% to 20% cases.29,38,39 One of the main complications associated to bronchial artery embolization is spinal cord infarction. The anterior spinal arteries can feed from branches of the bronchial arteries in 5% of patients, placing these arteries at high risk of unintentional embolization when treating bronchial bleeds.24 Currently with superselective embolization, the risk has been reported to be lower than 2%.40
NEUROLOGIC EMERGENCIES
Status epilepticus (SE) can be a life-threatening emergency when unrecognized and left untreated. SE is defined as either a persistent seizure for >30 minutes or repeated seizures with no recovery of consciousness between each episode. Importantly, any seizure activity that continues for >5 minutes should be treated as SE.41,42 The incidence of SE in the United States is 18.3 to 41 episodes per 100,000 patients/year and about 7% of seizures progress to SE.41 In the cancer population, about 13% of patients experience seizures at some point during the course of their disease; 50% of these seizures are secondary to brain metastasis or primary brain tumors.43 Nevertheless, the prevalence of SE is no higher in cancer patients when compared to the general population.44
The causes for SE are the same in the general population and in patients with cancer. The most common causes are noncompliance to medications (29%), alcohol intoxication or withdrawal (26%), CNS infection (8%), stroke (6%), tumors (6%), trauma (6%), and anoxic encephalopathy (6%).44 In cancer patients, it is important to rule out malignancy-related causes of SE (Table 93-1). Poor prognostic factors are presence of anoxia, old age, multiple comorbidities, and brain tumors.41,42
Malignancy-Related Causes of Seizures
Tumor |
|
Medication |
|
Others |
|
Initial management of patients with SE should focus on stabilizing the patient’s airway and cardiovascular status. Laboratory workup should be performed to determine any possible metabolic or toxic causes as well as brain imaging to determine structural lesions. In patients with poor mental status or coma in whom nonconvulsive SE is suspected, an electroencephalogram (EEG) should be obtained. Lumbar puncture should be considered if an infectious cause is suspected. Supportive treatment for SE includes avoiding hyperthermia, hypoxia, hypotension, hyperglycemia, hyperventilation, and electrolyte imbalance.45,46 Treatment and stabilization of the patient should not be delayed by diagnostic tests and procedures since multiple studies have shown that delaying treatment of SE causes brain injury, and increases morbidity and mortality.45,47 Delaying treatment of SE can decrease the response to treatment from 80% to 30% and therefore increase the risk for refractory SE.45 Moreover, institutional protocols for treatment of SE have shown to improve outcomes.42,45
Initial management of SE should be with intermittent boluses of benzodiazepines.47,48 There are four double-blind randomized controlled trials comparing diazepam or midazolam with lorazepam.48-51 While initial studies did not show any preference for one benzodiazepine over the other, Alldredge et al reported that the use of lorazepam led to earlier termination of SE when compared to diazepam.49 Addition of hydantoins (phenytoin, fosphenytoin) to initial boluses of benzodiazepines is recommended due to the decreased efficacy of benzodiazepines after 30 minutes of sustained seizure activity42 although large studies have failed to show improved outcomes with this practice.51 Levetiracetam and valproic acid, while not approved by the Food and Drug Administration for SE, have also been used effectively.52,53 After the administration of any antiepileptic drug, clinical and EEG evaluation should be performed to determine if further seizure activity persists.
Refractory status epilepticus (RSE) is defined as seizures that persist after adding a second line of medication therapy.45,46 In RSE, only 7.3% of patients respond to administration of a second drug, and 2% to a third drug.51 Even though there are reports of survival after prolonged periods of SE, the prognosis is poor.41,42,44 Refractory SE requires further workup including reevaluation of administered doses of antiepileptic drugs (AED), discontinuation of medications that could decrease the therapeutic levels of AED, and further imaging such as magnetic resonance imaging (MRI), position emission tomography (PET), and single-photon emission computed tomography (SPECT) to rule out unidentified structural pathologies.41 After further workup has been performed, medication-induced coma is the next step in the treatment of RSE. In these cases, continuous EEG monitoring is the most reliable method for the evaluation of responsiveness to treatment.
Benzodiazepine infusion is used for the initial treatment of RSE. However, their efficacy becomes reduced with prolonged use.42 Surveys performed in Europe and North America report that barbiturates are the first drug of choice for RSE among neurologists and neurointensivists.47 Secondary effects of barbiturates include cardiovascular depression, aplastic anemia, and liver dysfunction.41 Propofol has also been used successfully for the treatment of RSE. There are no studies suggesting any benefits of propofol over benzodiazepines; however, barbiturates have shown higher success rates and lower breakthrough seizures when compared to benzodiazepines and propofol.54 Ketamine, lidocaine, and inhaled anesthetics have also been used for RSE.41 If there is no response after a single medication infusion, a combination should be considered, being cautious of possible potentiating side effects. Favorable outcomes have been reported in patients who received simultaneous midazolam and propofol infusions.55 Multiple studies have proven the efficiency of newer drugs such as lacosamide and sec-butyl-propylacetamide (a derivative of valproic acid) in SE.56,57 Hypothermia, described in case reports and studied in animal models, appears to also have an encouraging role in the treatment of SE.58,59 However, further studies are required before any of these newer treatment options become standard of care.
Titration of infusions should be considered after 24 to 48 hours of no seizure activity on EEG.42,45 Slow titration, while continuing other AEDs, should be performed with careful observation for epileptiform activity on EEG or clinical evaluation. If all of these measures are ineffective, surgical intervention, electroconvulsive therapy, and transcranial magnetic stimulation should be considered.41,45,46
Spinal metastatic disease occurs in 40% of patients with osseous metastasis and 5% to 10% of these patients develop malignant spinal cord compression (MSCC).60,61 Lung, breast, and prostate cancer account for 20% of cases; non-Hodgkin lymphoma, multiple myeloma, and renal cancer account for another 5% to 10%, and the others are attributed to sarcomas, colorectal and unknown primary tumors.62-65 The most common mechanism of spinal involvement by tumor is hematogenous spread and tumor embolization; only 15% of cases are due to direct invasion of the spinal canal by a growing paravertebral tumor.60,62 After involvement of the spine, the tumor can cause MSCC by two different mechanisms: (1) the tumor grows, invades the epidural space, and then compresses the medulla; (2) the tumor causes vertebral fracture and bone fragments compress the spinal cord.60,64 Compression of the spinal cord causes edema, decreased vascular flow, and ischemia that can be irreversible.
Early recognition of MSCC is vital as several studies have demonstrated that restoration of neurological function and prognosis are directly related to the degree of initial neurologic damage.62,66 Pain is the first symptom in 83% to 90% of cases.64 The pain can be localized, which suggests direct invasion of the tumor into the epidural space; radicular, which may be caused by impingement of nerve roots; and pain that worsens with movement due to instability of the spine after vertebral body collapse.64 Rodichok et al demonstrated that over 60% of patients with cancer complaining of back pain had compression of the epidural space by tumor even if no neurological deficits were present.67 Motor deficits are also common during MSCC; 35% to 75% of patients complain of a certain degree of weakness on presentation, and about 50% to 68% of patients diagnosed with MSCC cannot walk before initiation of treatment.60,68 Hamamoto et al observed that motor deficits were present in 54% of patients with middle thoracic spine involvement, 30% with lower lumbar spine, and 15% with cervical spine involvement.66 Sensory deficits can also be present in about 50% to 70% of cases, but are usually not recognized by the patients.68 Autonomic dysfunction, presenting with bowel and bladder incontinence, occurs late in the progression of disease and is a poor prognostic factor for recovery.60,64,68
The gold standard for diagnosis of MSCC is MRI. Prior to MRI, spine radiographs, CT scans, and myelograms were performed until studies proved that MRI was the most cost-effective method for diagnosis of MSCC.69 All patients suspected with MSCC should have a whole spine MRI as 17% to 30% of patients have multiple spinal lesions. These lesions should be characterized for risk stratification for SCC.63 Patients with evidence of laminar involvement or metastasis in the midthoracic spine can be high risk for MSCC and should be considered for early intervention.66 Angiography is useful in hypervascular tumors such as sarcoma, melanoma, thyroid, and renal cancer when embolization of these tumors is considered prior to surgery.62
Treatment of MSCC is an emergency and requires immediate and quick evaluation by a multidisciplinary team that includes oncologists, radiation oncologists, and neurosurgeons. Survival after diagnosis of MSCC is usually 3 to 6 months.61,63 Treatment is therefore palliative and should aim to decrease pain and preserve or restore neurologic function.62,64 Scoring systems have been created to evaluate the patient’s prognosis and serve as a guide for treatment.70-73 These scoring systems include functional status, type of tumor, number of bone metastases, degree of neurologic dysfunction, number of visceral metastases, and response to radiotherapy.73 Harrington score classifies spinal metastasis according to the degree of neurologic dysfunction, and is used as a guide to select candidates for either surgery or radiotherapy.74
Radiation therapy has been the main treatment for MSCC since the early 1950s.60,73 Indications for radiation therapy include absence of spinal instability, patients unable to tolerate surgery due to poor functional status, life expectancy less than 6 months, diffuse spinal disease, neurological deficit ongoing for over 24 to 48 hours, and sensitivity of the primary tumor to radiotherapy.68 Treatment with radiotherapy has been effective in reducing pain, tumor size, and preserving neurologic function.60 Optimal dosing and frequency of radiotherapy have not been well established since no studies have shown improved outcome when comparing different protocols.75
Outcomes of patients undergoing surgical decompression have improved significantly since the 1980s. Initial surgical interventions consisted of only laminectomy; however, with new approaches, neurosurgeons are able to decompress the spinal cord, perform cytoreductive resections to avoid recurrence, and reconstruct and stabilize the spine.61,64 Indications for surgery include progression of tumor despite radiation, neurologic deterioration during radiation, significant cord compression, medically intractable pain, radioresistant tumors, and evidence of spinal instability.68 In 2005, Patchell et al published the first randomized clinical trial comparing the treatment of MSCC with radiotherapy alone versus radiotherapy combined with surgical intervention (generally within 24 hours).76 These investigators found that patients who had undergone surgical intervention and radiotherapy were more likely to regain neurologic function (gait and urinary continence) had better pain control, and improved survival than those receiving radiation alone.76 A meta-analysis by Klimo et al replicated these results.61 Despite these encouraging results, it is important to take into account the patient’s overall status and prognosis before undergoing any surgical procedure.64
Corticosteroids should be administered as soon as the diagnosis of MSCC is made. These agents not only decrease edema, but may also have a direct effect on tumor destruction such as in cases of lymphoma.64
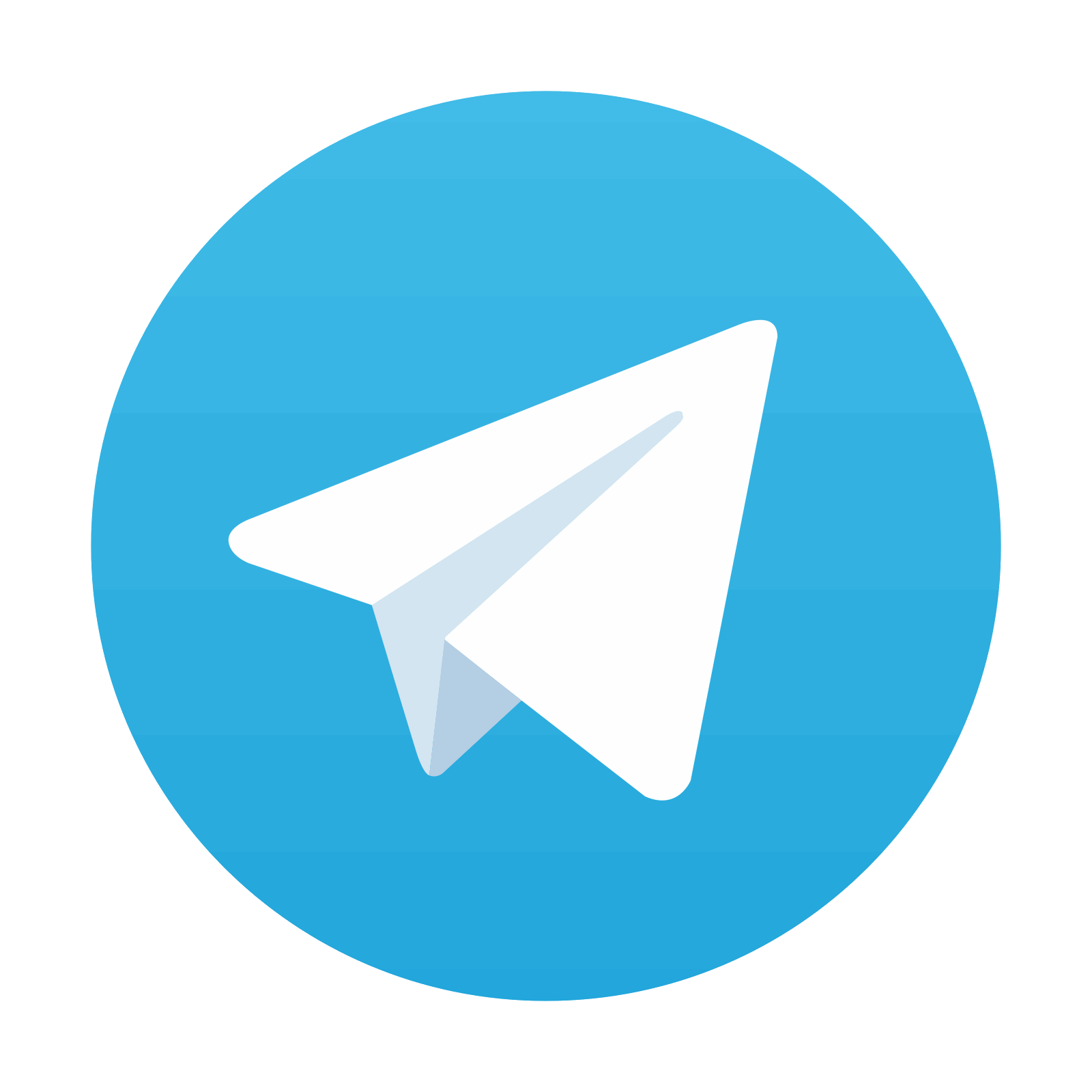
Stay updated, free articles. Join our Telegram channel

Full access? Get Clinical Tree
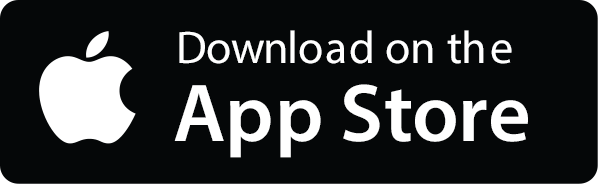
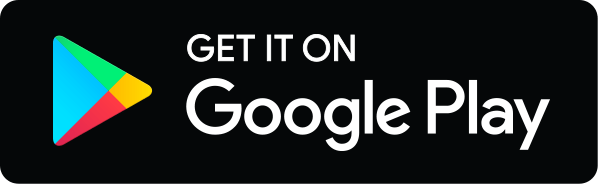
