div class=”ChapterContextInformation”>
2. Pharmacology of Anesthetic Agents in Children
Keywords
PharmacokineticsChildrenPharmacodynamic changesChildrenPharmacologyNeonatesDrug clearanceChildrenPediatric drug dosesPediatric anesthetic drugsAs children grow, absorption, distribution and clearance change because anatomical and physiological processes mature. Many drugs are poorly studied in children. Data is often extrapolated from adults as there are financial and ethical problems with clinical pediatric studies. These problems often mean newer drugs are not approved for use in children. Fortunately, the pharmacokinetics of many drugs commonly used as part of anesthesia have been studied, though less so their pharmacodynamics. This chapter focuses on the pharmacological differences between children and adults.
2.1 Factors Affecting Dosage in Children
Size and age are the most important determinants of drug dose in children. Size is most commonly dealt with by weight-based dosing but age affects organ function and body composition, which require more complex adjustments to dosage.
2.1.1 Size
Children can be less than a kilogram or more than 100 kg. There are three alternatives to allow for this. The first is weight-based dosing (mg/kg, up to a maximum equal to the adult dose). This is simple, accurate enough for most drugs and commonly used in anesthetic practice. Size and metabolism are not linearly related however, and the other two methods of dosing try to allow for this. Body surface area is one method. This requires complex calculations and is used for drugs with low therapeutic margins such as chemotherapy agents. The other alternative is to scale the dose using a non-linear, allometric power technique. Allometric scaling describes the nonlinear relationship between size and organ function. It also requires complex calculations and is not used clinically.
2.1.2 Age
Pharmacokinetic and pharmacodynamic differences between children and adults are maximal in the first 2 years of life, making neonates and young infants at high risk of side effects.
2.1.3 Pharmacokinetic Changes
Pharmacokinetic differences in neonates and infants that affect their response to drugs
Absorption |
Slow gastric emptying until 6–8 months and reduced gastric acidity in infancy Thin neonatal skin, increasing absorption of EMLA and chlorhexidine antiseptic |
Volume of distribution increased |
Increased total body water (mostly as increased ECF) |
Decreased fat and muscle as a proportion of body weight in neonate; increased and more sustained peak concentration of drugs that redistribute into fat and muscle |
Decreased albumin (and affinity), decreased alpha-1 acid glycoprotein |
Clearance |
Decreased metabolism in neonate, especially if preterm. Varies with different P450 isoenzymes. Most conjugation enzymes also decreased |
Renal function immature during first 6 months, adult level by 1–2 years |
Changes in body composition affect the physiological spaces into which drugs distribute. The high proportion of total body water (TBW) and extracellular fluid (ECF) in neonates (75% and 50% of body weight respectively) are the major factors, along with changes to fat, muscle, plasma protein levels and regional blood flow differences.
Keypoint
Neonates are ‘wet’ and ‘skinny’ at birth, increasing the apparent volume of distribution for many drugs.

Maturation of clearance, expressed as a percentage of adult capacity. Glucuronide conjugation, responsible for paracetamol and morphine metabolism, matures slower than the cytochrome P450 isoenzymes responsible for l-bupivacaine metabolism. Cytochrome P450 isoenzymes also contribute to the metabolism of propofol during infancy, whereas propofol undergoes glucuronide conjugation in older children. Blood and tissue esterases which metabolise remifentanil and atracurium are fully active in term, and probably preterm, infants. Adapted from Anderson, Eur J Anaesthesiol 2012;29: 261–70

Schematic representation of weight-based clearance of many drugs during childhood. Clearance is generally lower in neonates compared with adults due to reduced metabolism, then increases in toddlers and decreases gradually during childhood to the adult level. Although the shape of this curve is helpful in the clinical setting, it is an artefact caused by the weight-based calculation of clearance

The dose in mg/kg of many drugs is larger in infants and young children
Keypoint
The speed of maturation of metabolism varies between children and increases inter-individual variability of drug effects in children.
Note
The doses of three anesthetic drugs do not change with age because they are metabolized by esterases which are fully active at birth: suxamethonium, remifentanil and atracurium.
2.1.4 Pharmacodynamic Changes
The neuromuscular junction is not fully developed in neonates, affecting muscle relaxant action. The CNS is not fully developed, affecting the MAC of volatile agents. Although end-organ maturation has an effect on the action of other drugs, it is the pharmacokinetic changes that are most important beyond infancy.
Keypoint
In general, drugs have longer duration of effect in neonates. Children aged 1–2 years need higher doses in mg/kg, and these doses are shorter in effect. The dose and effects of drugs in children beyond 2 years age gradually change to adult levels during childhood.
2.1.5 Pharmacogenomics
Genetic influences on drug metabolism is another factor affecting drug dosage. Genetic polymorphisms affect how a drug is used in an individual child, or what drug-drug interactions might occur. Phenotyping will become more available for children requiring treatment with drugs dependent on polymorphic enzymes for metabolism. The recent understanding of genetic influences on codeine metabolism has led to its removal from pediatric practice. Another example is the metabolism of tramadol.
2.2 Licensure of Drugs in Children
Commonly used drugs and minimum age recommended by manufacturer
Drug | License age |
---|---|
Propofol | Over 3 years |
Fentanyl | Over 2 years |
Remifentanil | Over 1 year |
Oxycodone | Adults |
Ropivacaine | Term neonate |
Atracurium | Over 1 month |
Ondansetron | Over 2 years |
Dolasetron, Tropisetron | Adults |
Sugammadex | Over 2 years |
2.3 Drug Errors
Reducing drug errors in children
Techniques to reduce drug errors in children |
---|
Have another person in theater check unusual doses, unusual drugs, or difficult calculations |
Have another person in theater check the preparation of infusions |
Label drugs carefully. Do not rely on color of a drug or memory |
Avoid diluting drugs if possible, or always use the same or a standard dilution for each drug |
For drugs that are not titrated to effect such as antibiotics, draw up only the dose to be given |
Cross check by comparing the dose with an adult dose—“If an adult dose is for 50 kg and the child is 10 kg, how does the dose I’m about to give compare?” |
Prescribe practical doses for postop use that are not complex for staff to calculate (such as 110 mg of paracetamol rather than 113 mg); or use increments of dose that match the strength of the drug preparation—paracetamol 24 mg/mL for example |
Write ‘micrograms’ in full to prevent one thousand times overdose from misreading abbreviation |
Flush the IV injection point after every dose of drug |
2.4 Local Anesthetic Creams
Local anesthetic creams are used to reduce the pain of venipuncture. However, children still often fear needles and do not believe the cream will work. EMLA ® is a eutectic mixture of lidocaine, prilocaine and excipients. It takes 45–60 min to work, although a longer duration is more effective. The larger the needle, the more likely it is to be felt. The cream continues to penetrate deeper and work better for at least the first few hours, though the skin can become ‘soggy’ if the cream is left on more than 3 or 4 h. It works for 1–2 h after removal, depending on duration of application. It vasoconstricts micro vessels which may make larger veins more obvious against a pale background. Prilocaine toxicity (methemoglobinemia ) is a concern in neonates. Absorption of EMLA through their thin skin is increased, and methemoglobin reductase activity is reduced. During the first 3 months, application to only one site for up to 1 h in a 24 h period is recommended.
Tetracaine (amethocaine ) gel (‘Ametop’ or ‘AnGEL’ cream) is faster in onset (30 min) and penetrates better than EMLA for IV insertion. It vasodilates microvessels and makes the skin red. Local skin reactions are rare, but more common than after EMLA. It should be left on no longer than 60 min and continues to work for 2 or 3 h after removal. Four percent of lidocaine cream (LMX-4) also takes 30 min to have a similar efficacy to EMLA.
2.5 IV Induction Agents
2.5.1 Propofol
Propofol is particularly useful in children because it suppresses airway reflexes and reduces emergence delirium.
2.5.1.1 Pharmacokinetics
Pharmacokinetic data for propofol at different ages
Age group | Vd (L/kg) | Clearance (mL/min/kg) |
---|---|---|
Neonates | 5.6 | 20 |
Child <3 years | 9.5 | 53 |
Child >3 years | 9.7 | 34 |
Adult | 4.7 | 28 |
2.5.1.2 Clinical Use
Summary of IV induction agent doses
Age group | ||
---|---|---|
Agent (dose mg/kg) | Neonates | Infants and children |
Propofol | 3 | 3–5 |
Thiopentone | 3 | 5–7 |
Ketamine | 1–2 | 1–3 |

A propofol bolus may cause a prolonged fall in blood pressure in neonates. Based on Welzing et al., Pediatr Anesth 2010;21: 605–11
2.5.1.3 Propofol Infusions in Children
Initial target propofol concentration in adolescents during maintenance with propofol given with analgesic agents
Intraoperative analgesic | Target concentration propofol (μg/mL) |
---|---|
Propofol alone | 4–6 |
Remifentanil or regional block | 3–4 |
Nitrous oxide | 4–5 |
2.5.1.4 Target Controlled Infusions (TCI)
There are two TCI models licensed in some countries for children—the Paedfusor and Kataria . Both have minimum age and weight settings and target plasma rather than effect site concentration. Age however is ignored as a variable by both models, although the Paedfusor does adjust assumed volumes when the when age is more than 12 years. In general, TCI pumps give children a bolus dose about 50% higher and a maintenance rate 25% higher compared to adult TCI models.
Problems and practical points of propofol TCI in children
Propofol TCI in children | Comments |
---|---|
Problems | Pump algorithms use averaged pharmacokinetic variables. Titration of the dose is still needed to allow for interindividual differences |
Target concentration in children is probably the same as in adults, but it is not known why this is the case when MAC for volatiles varies with age | |
Induction slower than manual propofol bolus which may prolong induction process in unhappy or uncooperative child | |
Practical points | If gas induction, start TCI target 1–2 μg/mL then increase as sevoflurane washes out. Closely observe depth of anesthesia and watch for hypotension |
Always add an analgesic component to reduce propofol dose: An effective regional block; remifentanil infusion; alfentanil infusion if short anesthesia; even just nitrous | |
If propofol used alone, need target about 6 μg/mL or more to prevent involuntary movement. Huge dose and PACU recovery is prolonged | |
Wake-up concentration reported as 1.3–1.8 μg/mL |
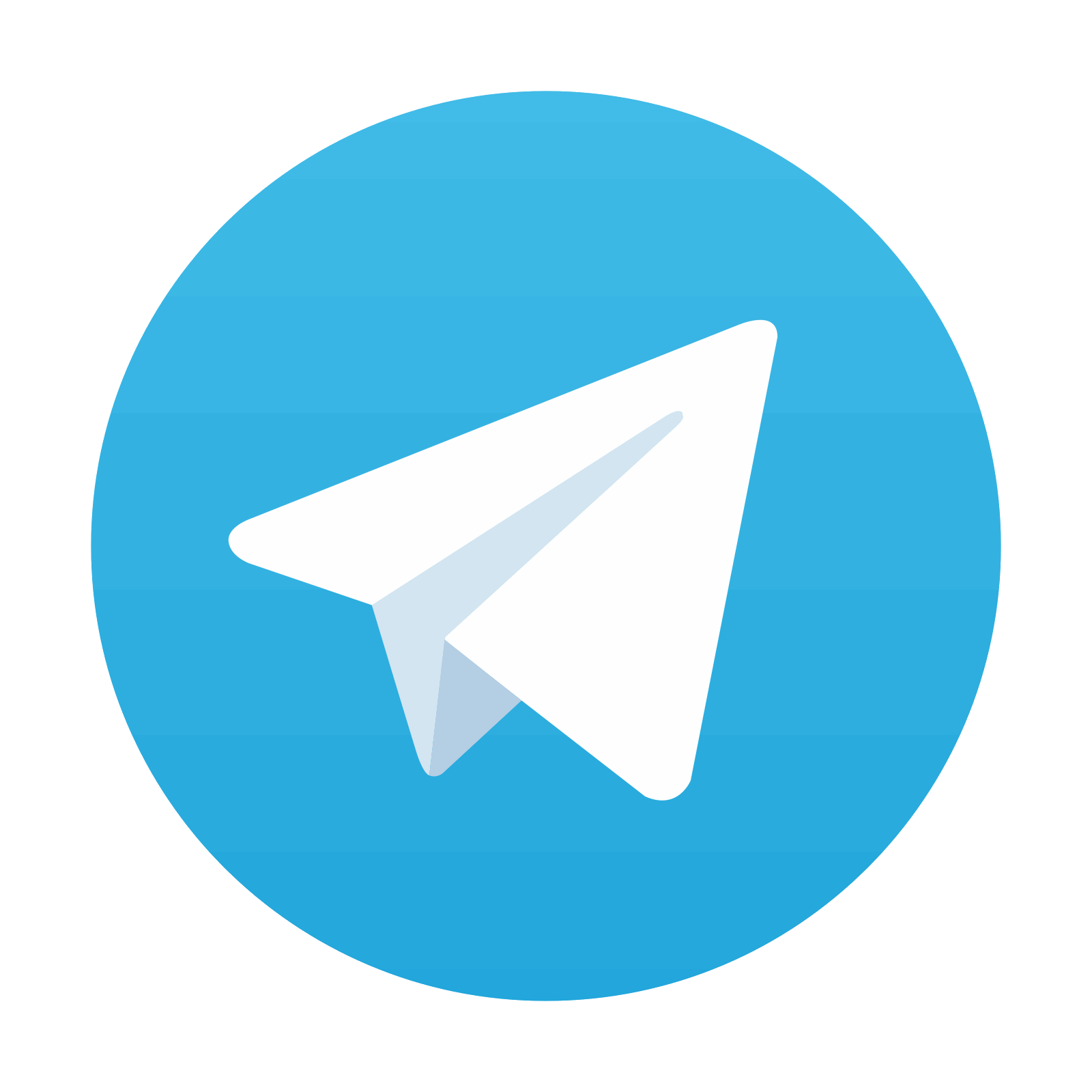
Stay updated, free articles. Join our Telegram channel

Full access? Get Clinical Tree
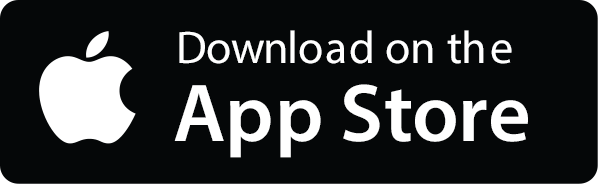
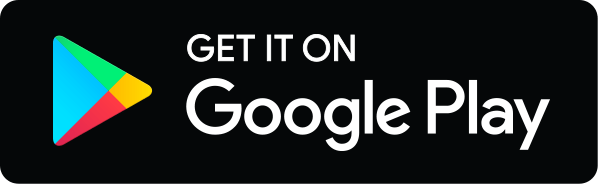