Nutritional Support
Werther Brunow De Carvalho
Artur F. Delgado
Heitor Pons Leite
KEY POINTS




NUTRITIONAL ASSESSMENT
It is known that in critically ill patients, anthropometric assessment is difficult to interpret because the measurements may be confounded by fluid retention and swelling. However, the consensus is that this assessment is very important for the nutritional evaluation and monitoring of patients in the PICU (1,2,3,4).
HISTORY AND PHYSICAL EXAMINATION
A good history and physical examination are the first steps toward establishing the requirements for nutritional support. The need for specialized nutritional support is often clinically obvious but detailed histories of the illness, of food intake, and of weight loss are important to establish a nutritional therapeutic plan. Nutritional repletion is indicated by weight gain, loss of edema, increased muscle strength, increased work capacity, improvement in appetite, and sense of well being (3,4,5,6).
Poor nutritional status has been associated with decreased respiratory function, impaired wound healing, and immune and gastrointestinal dysfunctions. Despite its high prevalence, malnutrition in hospitalized patients is poorly recognized by pediatricians. The classical presentation with signs of kwashiorkor is rare in children and adolescents with chronic diseases but the physical examination may reveal signs of malnutrition such as hair loss or change in texture, skin atrophy, pallor, etc. (7,8,9,10).
ANTHROPOMETRIC EXAMINATION
Anthropometric measurements are useful to assess nutritional alterations prior to hospital admission and to document the effects of long-term therapeutic interventions. However, they may not accurately reflect acute nutritional deterioration under conditions of metabolic stress. Despite the limitations in acutely ill children who are admitted to the PICU, anthropometric measurements are vital to allow for an objective assessment, enabling the detection of undernutrition and aiding in the planning and monitoring of nutritional support throughout the hospital stay. Anthropometric markers (measurements) are useful to document the severity of undernutrition upon admission to hospital. The anthropometric data for a population reflect its health, growth, and development status (3,6,8). As opposed to anthropometric markers, many biochemical markers do not have standardized values per age ranges.
There are well-established criteria for the nutritional assessment of a child without associated comorbidities, including those developed by the World Health Organization (WHO), the Center for Diseases Control and Prevention (CDC), and the National Center for Health Statistics (NCHS). All criteria assume a predictable sequence of events during nutritional deprivation beginning with weight loss and followed by linear stunting. Typical measurements use the weight-age ratio, the height-age or length-age ratio, the weight-height or length ratio and the BMI-age ratio, where:
BMI (Body Mass Index) = body weight (kg) ÷ height (m2)

The Waterlow criteria express the child’s anthropomorphic ratios as percentage of population medians determined from standard growth charts. Specifically, acute malnourishment (“wasting”) is determined by the ratio of the child’s actual weight as percentage of the expected weight for height, where the expected weight for height can be obtained by plotting the child’s height on the 50th percentile line of the growth curve to obtain the height-age. The 50th percentile for weight at the height age is
defined as the expected weight for height. Children whose actual weight is less than 90%, 80%, or 70% of their expected weight for height are considered to have mild, moderate, or severe acute undernutrition, respectively. Similarly, children whose height is less than 95%, 90%, or 85% of their expected height for age have mild, moderate, or severe stunting, respectively, reflecting chronic malnutrition.
defined as the expected weight for height. Children whose actual weight is less than 90%, 80%, or 70% of their expected weight for height are considered to have mild, moderate, or severe acute undernutrition, respectively. Similarly, children whose height is less than 95%, 90%, or 85% of their expected height for age have mild, moderate, or severe stunting, respectively, reflecting chronic malnutrition.
Clinical circumstances and comorbidities may confound height and weight measurements. Critically ill children are usually confined to bed, and accurate height or length values may not be available. Fluid and electrolyte imbalance, renal and liver malfunction with edema and/or ascites, or tumor mass may impair the accuracy of weight measurement. This difficulty in measuring weight and height can hinder the diagnosis and management of the nutritional status using the usual ratios.
Critically ill patients may undergo an intense protein catabolic process and have changes in body composition due to loss of muscle mass and rearrangement of total body water. Therefore, measurements that define the body compartments in more detail become more important, even as prognostic indices in the assessment of this patient. In the anthropometric assessment, circumference measurements and skinfolds are simple methods to estimate the body composition, for they reflect both the fat and fat-free components. The WHO recommends the mid-upper arm circumference (MUAC) to estimate the total skeletal muscular protein. A reduced fat-free mass (FFM)/height ratio is an important predictor of increased mortality (3,6). Once again, these measurements may be less reliable in the setting of edema.
NUTRITIONAL STATES
Undernutrition
Undernutrition is common at hospital admission and tends to worsen during hospitalization. In Europe and North America, 40%-50% of hospitalized patients are at risk of malnutrition (3,4,5). Several studies performed in Brazil and in other countries demonstrated that malnutrition can affect 50% of children and adolescents during hospitalization, although the classical kwashiorkor presentation is rare in critically ill infants, children, and adolescents with chronic diseases such as those in ICUs (9).

Critically ill, undernourished children are less able to handle substrate, liquid, and solute overload. The most common absorptive disturbances involve carbohydrates (particularly
lactose) and fats. Reductions in cardiac output, glomerular filtration rate, renal blood flow, or renal solute excretion capacity may arise from undernutrition. Intracellular potassium and sodium pump activity is lowered. In view of these limitations, caution is urged in initial phases of treatment as excessive volume intake of water and nutrients may cause complications or death (7). Well-nourished children who are likely to return to normal feeding within 4-5 days do not generally require artificial nutritional support, whereas undernourished patients with hypermetabolism or hypercatabolism should receive treatment as swiftly as possible (8).

TABLE 97.1 SIMPLE VERSUS STRESS STARVATION | ||||||||||||||||||||||||||||||||||||
---|---|---|---|---|---|---|---|---|---|---|---|---|---|---|---|---|---|---|---|---|---|---|---|---|---|---|---|---|---|---|---|---|---|---|---|---|
|
Starvation
Fasting (a reduction or absence of the intake of nutrition) becomes starvation when suffering or the process of perishing occurs. The physiologic changes that occur with severe malnutrition and starvation may be a result of inadequate intake of energy and basic nutrients (simple starvation) or it can be a result of increased energy expenditure (EE) unmatched by adequate intake (stress starvation). Stress starvation can develop more quickly than simple starvation (7,9,13) (Table 97.1).
For didactic purposes, the starvation response may be divided into three phases, although the biochemical events overlap and depend on baseline nutritional status, comorbidities, and the extent of the nutrient restriction (complete or partial). During phase I, glucose remains the primary source of energy and is produced by the breakdown of absorbed carbohydrate and glycogen stored in muscle and liver. Phase I begins after the consumed meal has been digested and the body has entered the postabsorptive phase. Glycogen stores are usually exhausted within 24 hours in young children.
During phase II, fats become the primary source of energy after glycogen stores have been depleted. Lipolysis mobilizes fat stores to release free fatty acids, which are oxidized to ketone bodies (aceto-acetate and β-hydroxybutyrate). These ketone bodies serve as an alternative source of energy for the brain in the face of limited glucose supply. Laboratory analysis reveals mild ketoacidosis at this stage. Proteolysis liberates amino acids from muscle to support gluconeogenesis and mitigate the risk of hypoglycemia. Hormonal changes including falling insulin levels and rising glucagon (and other counterregulatory hormone) levels contribute to maintenance of serum glucose levels.
If starvation continues, the fat stores become exhausted and the body enters phase III of starvation. During this phase proteins become the major source of energy. This phase is characterized by further breakdown of muscle (including cardiac muscle) in order to support gluconeogenesis, a process known as “protein wasting.”
Adaption to starvation involves reducing EE by suppressing metabolic rate, body temperature, and delaying growth/
reproduction (10,13). During stress starvation, the normal adaptative responses of simple starvation, which conserve body protein, are overridden by the neuroendocrine and cytokine
effects of injury. Metabolic rate rises rather than falls, ketosis is minimal, protein catabolism accelerates to meet the demands for tissue repair and for gluconeogenesis, and there is hyperglycemia and glucose intolerance. Salt and water retention is exacerbated, and this may result in a kwashiorkor-like state with hypoalbuminaemia and edema.

effects of injury. Metabolic rate rises rather than falls, ketosis is minimal, protein catabolism accelerates to meet the demands for tissue repair and for gluconeogenesis, and there is hyperglycemia and glucose intolerance. Salt and water retention is exacerbated, and this may result in a kwashiorkor-like state with hypoalbuminaemia and edema.
Refeeding Syndrome
Refeeding syndrome (nutritional recovery syndrome) is a potentially lethal condition that can occur usually within 1-3 days after reinstitution of nutrition following 5-10 days of fasting. Patients at risk include those with severe undernutrition and anorexia nervosa. The major manifestations of refeeding syndrome include the following:
Hypophosphatemia
Hypokalemia
Hypomagnesemia
Fluid overload
Thiamine deficiency
The pathophysiology of refeeding syndrome begins with a contraction of intracellular spaces and depletion of major intracellular ions (phosphorus, potassium, and magnesium) during starvation, yet normal serum levels are maintained (14). Refeeding of carbohydrates increases glucose levels, stimulates insulin release and induces protein, glycogen, and fat synthesis. Elevated insulin levels shift phosphorus, potassium, magnesium, and thiamine to the intracellular space. Hence serum levels of these ions may fall rapidly.
Cardiovascular manifestations of refeeding syndrome include heart failure and dysrhythmias. Preexisting cardiac atrophy from starvation exacerbated by thiamine and phosphate deficiency make the patient vulnerable to heart failure and fluid overload. Because most severely undernourished patients are bradycardic, the restoration of a “normal” heart rate may be a sign of impending heart failure.
Pulmonary complications of refeeding syndrome involve respiratory muscle weakness. A weak cough reflex leads to retained airway secretions, atelectasis, and pneumonia. Hypophosphatemia may lead to ventilatory failure.
Neurologic complications may entail seizures, delirium, and frank encephalopathy from thiamine deficiency (Wernicke’s encephalopathy).
Prevention is the key to management of refeeding syndrome. Electrolytes abnormalities should be anticipated and corrected when discovered in the severely undernourished child. Nutritional supplementation (especially caloric intake from carbohydrates) should be advanced gradually. Excess fluid and sodium administration are avoided. Observation for cardiac arrhythmias is particularly important, as this is the major cause of fatalities. Vitamin supplementation (especially thiamine) should also be implemented with refeeding and continued for at least 10 days.
Hypercatabolism
A hypercatabolic state occurs in response to many clinical conditions (sepsis, trauma, burns, etc.) and is characterized by an increase in circulating catabolic hormones and inflammatory cytokines as well as insulin resistance. Hyperglycemia, glucose intolerance, and insulin resistance are common features of critically ill patients, especially in patients with sepsis or septic shock.
Nutrition in children with severe infection, trauma, or following major surgery is hampered by the hormonal and metabolic changes associated with the systemic inflammatory response (8,15,16). The systemic inflammatory response activates the sympathetic nervous system and the hypothalamic-pituitary-adrenal axis. These responses are characterized by changes in glucose and lipid metabolism, along with increased protein turnover and breakdown, which results in increased EE, a negative nitrogen balance, and muscle protein loss. Deamination of branched-chain amino acids supplies amino acids for the protein synthesis required for wound healing and the immunologic response (production of immunoglobulins and acute-phase reactants) (15,16). Peripheral resistance to insulin action ensures continued glucose production with hyperglycemia, reduced glucose oxidation, and storage as hepatic glycogen. Increased lipolysis provides free fatty acids for energy and glycerol for gluconeogenesis (16,17,18). Levels of catecholamines, cortisol, glucagon, growth hormone, aldosterone, and antidiuretic hormone are increased, and insulin is generally elevated but not sufficiently to impede hyperglycemia. Insulin secretion is initially suppressed by the α-adrenergic mechanism and later increased together with glucagon levels. Peripheral resistance to growth hormone action occurs, with a reduction in insulinlike growth factor (IGF)-1 secretion, while hyperglycemia and lipolytic effects remain. Increased counterregulating hormone concentrations induce insulin- and growth hormone-resistant states (a characteristic sign of stress), which results in protein catabolism and the utilization of carbohydrate and fat stores to meet the increased basal metabolic rate. All of these mechanisms constitute the interaction between the nervous, endocrine, and immunologic systems in an effort to mediate stress (19,20,21).
An additional pathophysiologic process is the synthesis and release of inflammatory and metabolic response mediators by monocytes (primarily Kupffer cells and alveolar macrophages). These mediators include cytokines, products of arachidonic acid metabolism, and platelet activation factors. (Interleukin 6 is an important cytokine that causes an inflammatory response and increases nutritional risk.) Acting locally or distally, they promote changes in cell function and metabolism. Such mediators also act directly on hypothalamic activity, influencing the release of classic stress hormones (9,17). Continued hypermetabolism results from complicating factors, such as hypotension or infection, leading to accelerated malnutrition and lowering of immunologic function (15,17,22).
Obesity
Childhood obesity is a public health crisis of epidemic proportions throughout the world, with an estimated prevalence of 10%-25% (23). Severe childhood obesity is defined as a body mass index (BMI) >35 kg/m2. Pseudotumor cerebri, slipped capital femoral epiphysis, steatohepatitis, cholelithiasis, and sleep apnea represent some of the major comorbidities associated with severe obesity (1,24). Obese adults with severe respiratory failure have higher mortality rates (24,25). Recent data also suggest that obesity may be associated with increased mortality in critically ill children (26). Special problems for morbidly obese patients in the PICU include the following:
Difficult airway
Risk of gastroesophageal reflux and acid aspiration
Obstructive sleep apnea and obstructive hypoventilation (requiring caution with use of opioids and sedative medications)
More rapid desaturation during rapid sequence intubation (decreased functional residual capacity and increased O2 consumption)
Drug dosing usually based on lean body weight or ideal body weight rather than total body weight
Insulin resistance is associated with obesity and may be secondary to the presence of adipocytokines. As a result, morbidly obese patients exhibit variable glucose homeostasis
including glucose intolerance, overt type II diabetes, or metabolic syndrome. Clinical clues to the presence of insulin resistance include abdominal obesity, acanthosis nigricans, hirsutism, muscle cramps, hypertension, and hypertriglyceridemia, among others.
including glucose intolerance, overt type II diabetes, or metabolic syndrome. Clinical clues to the presence of insulin resistance include abdominal obesity, acanthosis nigricans, hirsutism, muscle cramps, hypertension, and hypertriglyceridemia, among others.
Nutritional management of the morbidly obese patient takes into account comorbidities, special ICU problems, and insulin resistance. Excess carbohydrate administration may lead to increased CO2 production and delayed weaning from mechanical ventilation. Insulin resistance may produce hyperglycemia, which has been associated with poor ICU outcomes. Therefore some adult studies have suggested the use of hypocaloric—high protein diets in adults with obesity (27). The goal is to avoid the complications of CO2 retention, hyperglycemia, and fatty liver, while achieving positive nitrogen balance, which may promote wound healing. There are no randomized controlled trials on this approach in children.
NUTRITIONAL MANAGEMENT
Although some critically ill children present in a hypermetabolic state (major burns, multiple injuries, and prolonged admission), the majority have a lower EE than healthy children, particularly those receiving mechanical ventilation or sedation and muscle relaxation. The aims of nutritional therapy during critical illness are to provide fluids and nutrients in adequate quantities to maintain vital functions and homeostasis, to recover the activity of immune system, to reduce the risks of overfeeding, and to ensure an adequate supply of protein and energy sources necessary to minimize the protein catabolism and nitrogen loss. Implementation of evidence-based recommendations improved the provision of nutritional support and was associated with improved clinical outcomes (8,9,10,21). Conversely, several reports have shown that inadequate provision of nutrients is associated with increased physiologic instability in critically ill patients (8,22,28).
Component Requirements
An effective nutritional plan requires understanding of the individual components to be provided via the enteral or parenteral route.
Fluid Intake
Fluid requirements depend on a patient’s clinical picture. Daily assessment of weight, urinary osmolality, and fluid balance provides a good estimation of hydration state. Fever, increased ambient temperature, hypermetabolism, and liquid loss through diarrhea or digestive juices all imply further water loss and call for increased water intake. A significant weight loss from one day to the next tends to reflect abnormal liquid loss, and conversely, marked weight gain may be the result of excessive water intake or retention. Fluid losses through diarrhea or ileostomy drainage should be replaced daily. Fluid retention that stems from the changes in endothelial permeability that occurs with the systemic inflammatory response may require fluid restriction. Hypoxia and systemic hypotension may cause renal cortical or tubular necrosis, compromising renal function. In the presence of acute renal insufficiency, the volume required to supply protein-calorie needs should be administered in association with renal replacement therapy to remove excess fluid. Once the systemic inflammatory response has resolved, and if there is no further need to restrict volume, an increase of up to 50% over basal fluid requirements can be made to improve nutrient intake and promote anabolism (11,19,29,30,31).
Energy Intake
Adequate energy intake in critically ill children is essential to provide sufficient substrates for metabolic functions during the acute phase of the disease process, in addition to providing for growth of the child. However, excessive amounts of energy, particularly in the form of glucose, can be deleterious to the patient and can result in lipogenesis, increased respiratory quotient, increased carbon dioxide production, hepatic steatosis, and liver dysfunction. The caloric requirement for sedated infants in intensive care during acute metabolic stress is limited to that needed to reach basal metabolic rate plus a stress factor, which, depending on the clinical situation, is between 1.1 and 1.2 (18,19,30,32).
The basal metabolic rate in newborns and infants is ˜50-55 kcal/kg/day and falls steadily until adolescence to 25 kcal/kg/day. This calculation represents only an estimate of energy needs, considering that the rules tend to overestimate EE and can vary by up 30% over any given 24-hour period (1,8
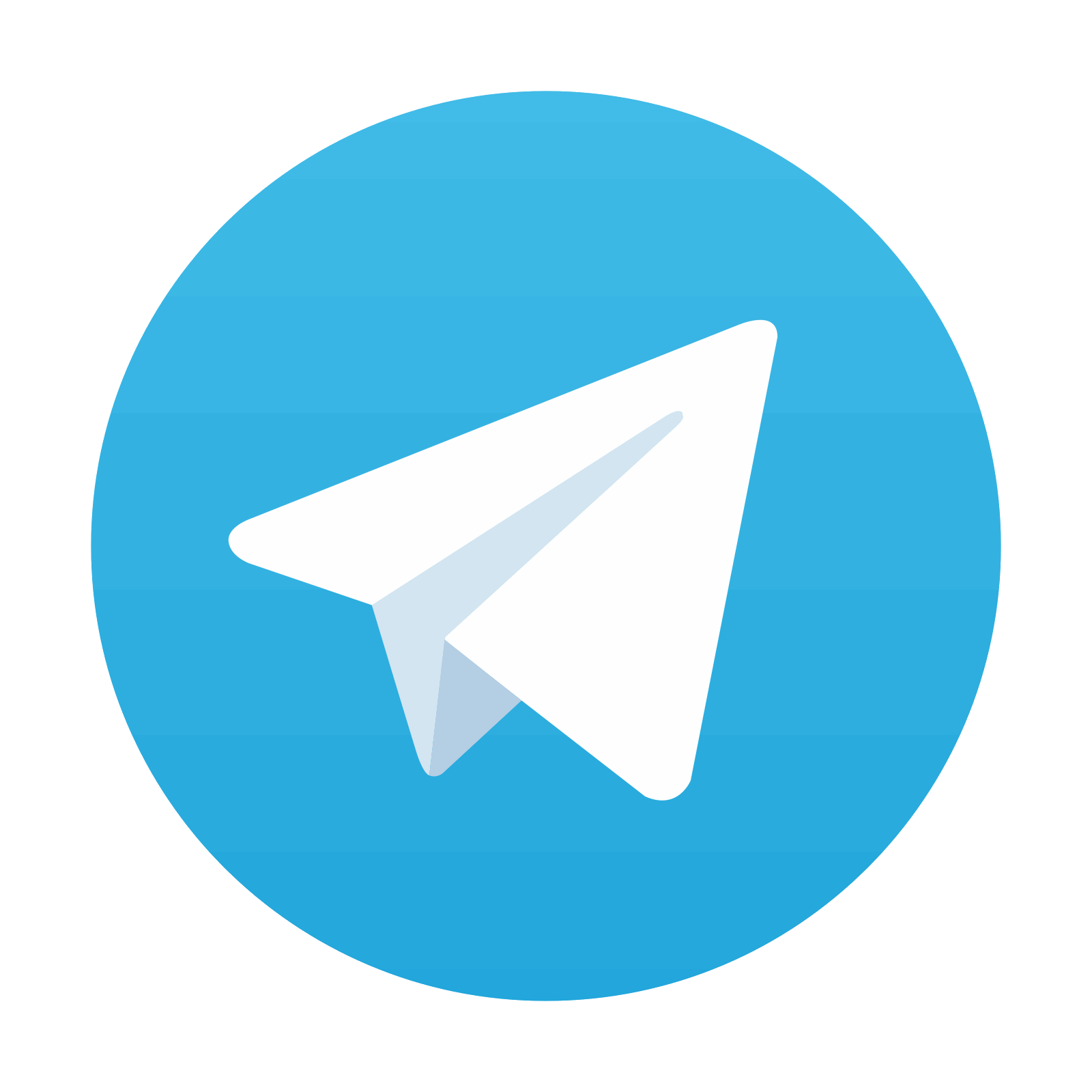
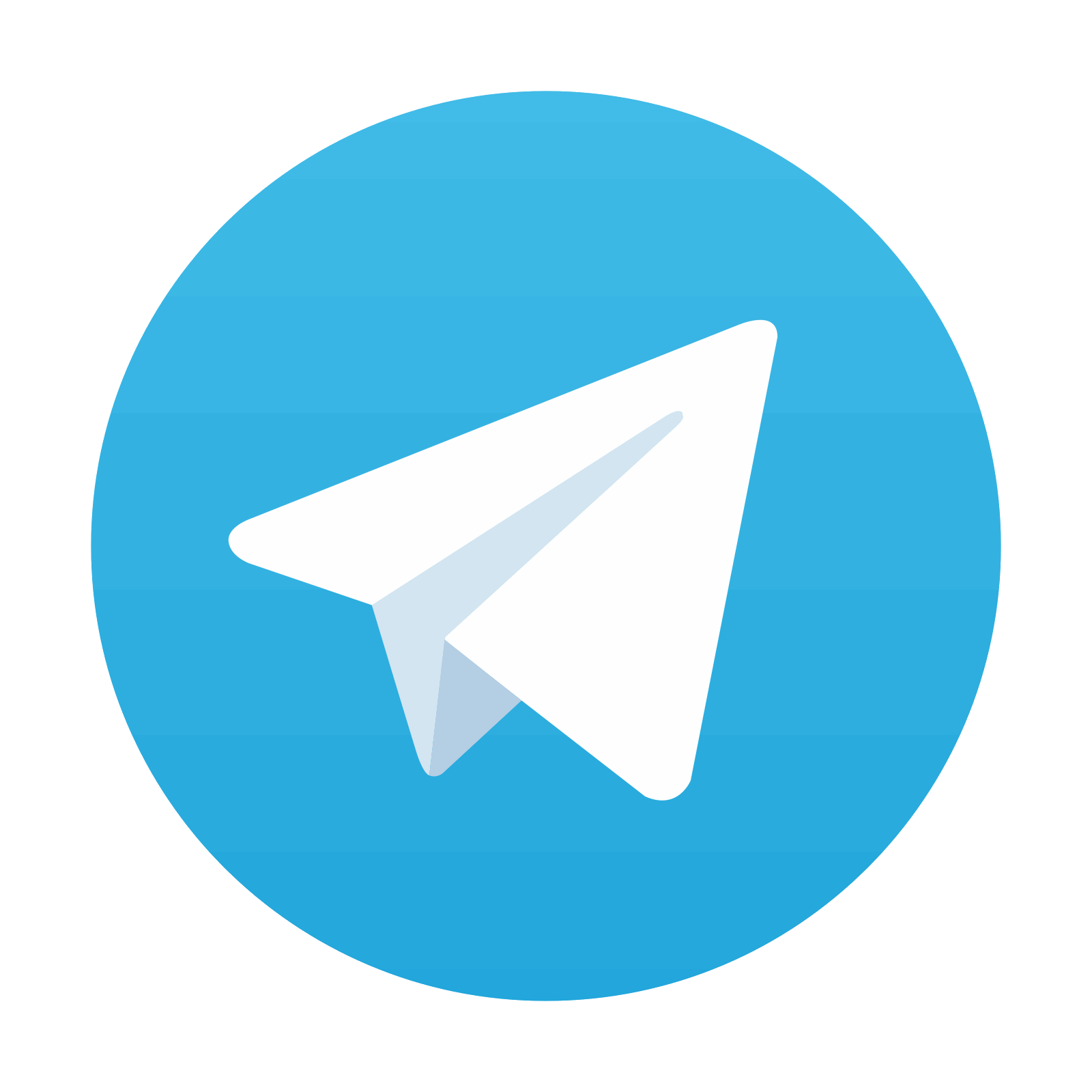
Stay updated, free articles. Join our Telegram channel

Full access? Get Clinical Tree
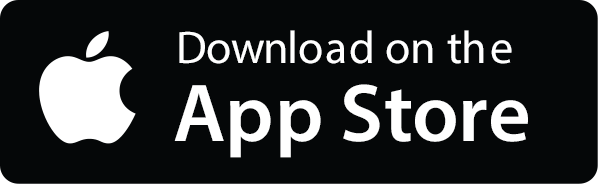
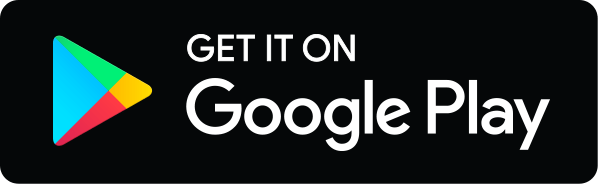
