Chapter 69 Nutrition, Malnutrition, and Starvation
For online-only figures, please go to www.expertconsult.com
OBSERVATIONS BY A TEST SUBJECT, MINNESOTA STARVATION STUDY, AFTER 24 WEEKS OF SEMI–STARVATION (1570 KCAL/DAY, 24% WEIGHT LOSS) (IN THE BIOLOGY OF HUMAN STARVATION, VOL. II58)
Importance of Nutrition in Stressful Environments
Nutrition has a profound underlying importance to human physiologic homeostasis and functioning on a day-to-day basis in everyday life; it becomes even more important when humans work or recreate in particularly challenging or “extreme” environments.10 The central role of nutrition is often underappreciated in wilderness expedition planning and may even be thought by some to be of minor importance. Many enthusiasts do not consider food as critical as gear and equipment, medical supplies, physical fitness, and other logistical considerations. In temperate environments, where food and water are plentiful and re-supply is feasible, the importance of nutrition may seem to diminish compared with other aspects of wilderness medicine. However, when a stressful physical environment is superimposed on the physically demanding tasks associated with wilderness activities, the role of nutrition rapidly becomes of prime importance for maintenance of performance and for prevention of disease and injury, as evidenced from the description of Napoleon’s disastrous 1812 winter retreat from Moscow:
BARON D. J. LARREY, INSPECTOR GENERAL, NAPOLEON’s MILITARY MEDICAL STAFF (IN HYPOTHERMIA AND WARFARE, NAPOLEON’s RETREAT FROM MOSCOW, 181290)
Environmental Stress and Nutrient Requirements
The physical and physiologic conditions of an individual (e.g., body weight, strength, coordination, fluid and electrolyte balance, and core temperature) play a significant role in determining nutritional requirements to maintain homeostasis. These conditions also directly influence survival time, especially when humans are deprived of food or water. In the short run, the most important nutrient is water.9 If an adequate supply of water is not available, all discourse on the physiology of starvation and malnutrition is pointless, because death from dehydration will occur prior to depletion of energy stores. Humans can survive food deprivation for extended periods of time—weeks or even months—depending on their level of body fat. A nonobese adult may live as long as 60 to 70 days while fasting in clinical setting.52 At the end of this time, almost all body fat and one-third of the lean body mass would be lost.51 James Scott, a victim of unintentional situational starvation, was marooned in a snow cave in the Himalayas with water but no food. He survived 43 days without food while losing one-third of his body weight, although he was near death at the time of rescue.104 Death from starvation in nonobese individuals is imminent if approximately 50% of body weight has been lost. This usually corresponds to a body mass index (BMI) of 12 kg/m2, although under some circumstances, a BMI of as low as 11 may be encountered prior to death.31
Time to death after complete water deprivation is measured in days—estimates run from 6 to 14 days, depending on the rate of body water loss, which is influenced by the temperature, humidity, and activity level.51 Water is critical because it, more so than any other nutrient, is responsible for maintaining homeostasis of the internal environment.9 It provides an aqueous medium to transport heat from cells to blood, to solvate and pass nutrients between blood and cells, to serve as a medium for intracellular reactions, and to transfer metabolic products for redistribution or excretion via urine. Both the quantity of reactants and the volume of fluid in which they are dissolved influence cellular chemical reaction rates; hence, imbalances in hydration status can alter cellular and tissue function, such as the body’s ability to regulate temperature. Muscle contraction depends on transformation of chemical energy (ATP) to mechanical energy. Nearly three-fourths of the energy used for muscle contraction is released as heat. Unless localized heat production from metabolism and muscle contraction is dissipated, the heat burden can be structurally damaging to enzymes or other proteins. Water absorbs heat produced at the cellular level and transfers it to the surface of the skin, where it can be dissipated to the external environment. The importance of water is discussed in greater detail in Chapter 70. The focus of this discussion is on energy restriction and assumes an adequate supply of water. For planning purposes, most wilderness expeditions require 3 to 5 L (about 3 to 5 qt) of potable water per person per day.
Advances in food processing, preservation, and nutrient fortification have resulted in modern camping foods and the military equivalent, field rations, that can support health and performance in a variety of temperate environments, even if they are not consumed to complete caloric adequacy.73 However, nutrition that was marginally adequate in a temperate environment may rapidly become inadequate in wilderness environments characterized by extreme temperatures, terrain, and physical demands.72,74 Rodahl and Issekutz100 observed, “While short-term nutritional deficiencies in men at room temperature appear to have little or no detrimental effect on the capacity to do short-term, heavy work, there is a marked reduction in physical work capacity when a nutritional deficiency or nutritional stress is superimposed on a cold stress.” Superimposed stressors, such as extreme heat, cold, altitude, sleep deprivation, physical exertion, and food restriction, influence nutrient requirements7 and can jeopardize performance.* The complex interrelationship between environment and nutrition, and its effect on human physiology and performance, is depicted in Figure 69-1. Stressors in the form of environmental extremes can have serious consequences on health and performance. Proper nutrition can help counter detrimental environmental influences on physical and mental performance.2–7
Energy and fluid deficits arising from the interaction of environment and nutrition can negatively impact both physical39 and mental77 performance. Volitional physical activity as well as mood can suffer under caloric deprivation, depending on the magnitude and duration of the restriction. Motivation may be more acutely influenced by undernutrition than is actual physical performance.77 Nutritional deficits may have a greater effect on what individuals are willing to do (i.e., on their perceived mood, symptoms, and self-motivation) than on what they can do (psychomotor performance).108
Nutritional Considerations in Planning for Wilderness Activities
Current national nutritional recommendations are revised periodically and can be found in the most recent dietary reference intakes published by the Institute of Medicine, National Academies.54 The dietary reference intakes (DRIs)88 are reference values for nutrient intakes that can be used to assess and plan diets for healthy people. Publications that list DRIs can be obtained through the National Academies website (http://www.nap.edu/catalog.php?record_id=11767) and the National Institutes of Health website (http://ods.od.nih.gov/Health_Information/Dietary_Reference_Intakes.aspx). The DRIs include four categories: estimated average requirement (EAR), recommended dietary allowance (RDA), adequate intake (AI), and tolerable upper limit (UL). EAR is the nutrient intake level estimated to meet the needs of 50% of the individuals in a population group. RDA is the nutrient intake level sufficient to meet the needs of 97% to 98% of individuals in a population group. AI is an estimate of adequate intake when an EAR cannot be established. AI and RDA are similar but not identical. The UL is the highest daily consumption level of a nutrient; when UL is exceeded, the nutrient poses a risk of adverse health effects. In general, for nutritional planning for wilderness expeditions, the basic daily diet should meet or exceed the DRI recommendations, especially if the traveler plans to subsist on them for prolonged periods of time (greater than 10 days). In the short run (less than 10 days), adequate energy provision is likely to be the predominant dietary concern. In the short run, certain nutrients may assume more critical or primary roles in environmental extremes than they might normally fulfill in everyday life.
Cold, heat, and altitude stressors and their influences on macronutrient vitamin and mineral requirements have been a focus of considerable military and civilian research.8 Research conducted primarily in the era since World War II (WWII) and the Korean conflict has established that vitamin and mineral requirements are not significantly increased by cold exposure, although caloric requirements for thermogenesis and work may be elevated to varying degrees, depending on the cold challenge, clothing ensemble, and level of physical exertion.74 Work in cold environments can be adequately supported by various combinations of fat, carbohydrate, and protein, although certain combinations of macronutrients may be more beneficial than others in helping a person withstand cold exposure.4 As an example, Mitchell and colleagues81 demonstrated that cold tolerance (the length of time body core temperature could be defended during a controlled cold challenge) was favored by previous diets high in fat as opposed to diets higher in carbohydrate or protein. Subsequent research has indicated that the macronutrient source is less important in the cold than consuming enough total calories to support activity and thermogenesis.4,45
Food and Adaptive Thermogenesis
Mild cold exposure, even without increased physical activity, elevates energy expenditure in mammals, including humans.59,70,127 This process is called adaptive thermogenesis. Adaptive thermogenesis, or regulated production of heat by the body, is influenced by environmental temperature and diet.70 Figure 69-2 depicts the major sources of metabolic heat production in mammals. Heat can be generated from synthetic, oxidative, and/or uncoupling processes. Mitochondria, the organelles that convert food to carbon dioxide, water, and ATP, are fundamental in mediating effects on energy dissipation in response to an energy demand stimulus.48 Adaptive thermogenesis is a complex cascade of cell signaling events and primarily regulated by two major hormonal effectors: β-adrenergic agents and thyroid hormone. Thyroid hormones are major endocrine controllers of energy expenditure.48 Thyroid hormones are critical in providing a vigorous response to cold exposure and to sustaining that response by providing glucose and fatty acids to fuel energy production and thermogenesis.109 Thyroid hormone acts synergistically with catecholamines of the sympathoadrenal system during cold adaptation, times of high energy output and, conversely, when energy demands should be reduced, such as during starvation.109 Physiologic stimuli, such as cold exposure, elicit these thermogenic hormones, which in turn interact with specific cellular tissue ligand-activated nuclear receptors called peroxisome proliferator activated receptors (PPARs) in brown and white adipose tissue, liver, heart, and skeletal muscle. PPARs act as fatty acid sensors to control many metabolic pathways essential for energy homeostasis.125 PPARs are mainly expressed in white and brown adipose tissue, where they control expression of several proteins involved in upregulation of lipid metabolism, subsequent energy generation and thermogenesis. Peroxisome proliferator-activated receptor gamma coactivator-1α (PGC-1α) is a tissue-specific transcriptional coactivator protein that interacts with the nuclear receptor PPAR-γ. This permits interaction of this protein with multiple transcription factors, thus serving as a coactivator that enhances the activity of many nuclear receptors and coordinates cellular transcriptional programs important for energy metabolism and energy homeostasis. PGC-1α can interact with, and regulate the activities of, cAMP response element binding protein and nuclear respiratory factors that are involved in energy metabolism and thermogenesis. Uncoupling protein-1 (UCP1) is an uncoupling protein found in the mitochondria of brown adipose tissue (BAT). It is used to generate heat by non-shivering thermogenesis. Adrenergic and thyroid hormones have an impact on energy dissipation and subsequent heat production by the mitochondria in brown fat and skeletal muscle via a complex signaling mechanism involving PGC-1α, PPARγ, and UCP1.96 PPARγ is a nuclear receptor, subject to transcriptional coactivation by PGC-1α. PGC-1α is a transcription coactivator that plays a central role in regulation of cellular energy metabolism. It is induced by cold exposure, linking this environmental stimulus to adaptive thermogenesis.66 Induction of increased mitochondrial activity through activation of PGC-1α results in an increase in oxidative-type muscle fibers that in turn leads to enhanced resistance to muscle fatigue and increased tolerance to cold.63 PGC-1α is expressed at high levels in tissues where mitochondria are abundant and oxidative metabolism is active, such as BAT, heart, and skeletal muscle.96 The schematic relationship of these cell signaling and response elements and their potential interaction with cold, caffeine, and catechins is depicted in Figure 69-3.
Although considerable research has been accomplished recently on basic cell signaling mechanisms influencing gene activity in response to thermogenic stimuli, less applied research has been conducted on nutrients and whole-body cold tolerance. Older studies of food and cold tolerance have largely used core temperature responses to actual cold exposure along with a dietary intervention; more recent cold tolerance research has incorporated aspects of basic cell signaling research described above in response to other food-derived components. Most notably, resveratrol, a plant polyphenol found in abundance in sources such as grapes and Japanese knotweed, has been shown to induce mitochondrial activity via activation of PGC-1α, which in turn increases oxidative-type muscle fibers, enhanced resistance to fatigue, and increased tolerance to the cold in a rat model.63 Assuming the rat model research has applicability to human cold tolerance, these effects are all potentially beneficial attributes for facilitating strenuous cold weather activities. Human resveratrol cold tolerance studies are lacking. It is unlikely that the food products containing resveratrol could be consumed in quantities adequate to exert a similar effect to that seen in the supplemented rat model. However, numerous concentrated resveratrol supplements and even food bars containing as much resveratrol as 50 glasses of wine are commercially available, making a comparable dose level of resveratrol perhaps feasible for human ingestion.
Other Potential Thermogenic Nutrients
Recent research on the thermogenic properties of green tea seem to provide support for tea as a historically favored hot beverage for cold weather and high-altitude expeditions. In research directed at the possible role of green tea in stimulating thermogenesis in conjunction with weight loss diets to combat obesity, it has been found that green tea stimulates thermogenesis in a manner that cannot be completely attributed to its relatively low caffeine content.35,36 Green tea contains catechins that inhibit the enzyme catechol O-methyltransferase (COMT), which degrades catecholic compounds such as norepinephrine. Diminishing COMT’s net effect would be to permit higher and more sustained levels of norepinephrine, which in turn would permit a more sustained lipolytic response to support increased energy expenditure. Besides the catechin inhibition of COMT, green tea, as well as coffee, contains caffeine, which enhances thermogenesis by inhibiting the enzyme phosphodiesterase. Inhibition of phosphodiesterase prevents degradation of cyclic AMP, permitting lipolysis to continue and provide additional fatty acids for eventual oxidation.53 In addition to inhibition of key enzyme responders in the body’s “braking system” of the stimulatory effect of norepinephrine-activated lipolysis, catechins also inhibit nuclear factor kappa B (NFκB), a transcription factor that normally regulates PPARs. Upregulation of key enzymes involved in fatty acid oxidation by PPARs increases energy expenditure along with enhanced stimulation of fatty acid release afforded by elevated cAMP. Finally, catechins have a direct effect on expression of several uncoupling proteins that also can influence thermogenesis via uncoupling or decreasing the efficiency of oxidative phosphorlyation.61,70 The role of green tea and coffee in enhancing energy expenditure (thermogenesis) is shown schematically in Figure 69-3.
Other thermogenic “nutrients” may also have application in cold tolerance. Capsaicin is the major pungent compound found in certain pepper species and a common spice in many food products. It has been studied with mixed results for its thermogenic properties and influence on increasing fat oxidation.111 Capsaicin is believed to increase thermogenesis by enhancing catecholamine secretion from the adrenal medulla through activation of the central nervous system, resulting in β-adrenergic stimulation.53 It possibly upregulates certain uncoupling proteins in response to the catecholamine release.76 Polymorphisms in the receptor and promoter regions of genes of individuals may explain the variability in efficacy of capsaicin stimulation between individuals53 and some of the differences in capsaicin study results reported in the literature.46,50,111
Brown adipose tissue has been extensively studied in infants and animals under a variety of conditions. It helps maintain body temperature. It was formerly thought that this energy-rich and heat-generating tissue regresses with age.64,65 The recent discovery that humans contain more BAT than previously thought33,124 and that cold exposure activates BAT thermogenic activity in humans123 has stimulated BAT research in adult humans. In human cold exposure studies, BAT activity was inversely correlated with changes in distal skin temperature, suggesting a role for BAT in maintaining core body temperature.123 The unique uncoupling properties of BAT that make it such an efficient heat-producing organ may also be shared by other tissues, such as skeletal muscle. Wijers and colleagues127 have found that mitochondrial uncoupling in skeletal muscle during cold exposure may be one mechanism facilitating cold adaptive thermogenesis in humans. Dietary or pharmacologic approaches to stimulating BAT activity may be a promising way to turn on heat production during cold exposure. Future research on the metabolic and dietary control of adaptive thermogenesis may have particular relevance to diverse metabolic outcomes, such as obesity and weight loss research, as well as human cold tolerance.
Fat, Carbohydrate, and Protein Content of the Diet May Be Tailored to Different Environments
Although the most important nutritional concern in challenging environments, aside from water, is total energy intake, when wilderness activities shift from a sea-level, cold-weather environment to a moderate- or high-altitude, cold-weather environment, the macronutrient balance in the diet should be reconsidered. Although fat is an efficient and well-tolerated energy source during relatively low-power-output, cold-weather activities at sea level, it is not as well tolerated at high altitude.5 Substituting carbohydrate for fat and, to a certain degree, for protein can theoretically provide metabolic advantages to the individual’s critical oxygen economy when working at altitude.3 This is because carbohydrate is a more efficiently metabolized fuel at altitude than is fat, because it is already partially oxidized (i.e., it contains a higher ratio of oxygen atoms to carbon atoms) and therefore requires less oxygen to combust its carbon skeleton to CO2, H2O, and energy. Metabolizing carbohydrate for energy requires approximately 8% to 10% less inspired oxygen than is required to obtain a similar amount of calories from fat. A high-carbohydrate diet can reduce the symptoms of acute mountain sickness, enhance short-term high-intensity work as well as long-term submaximal efforts, and lower the effective “felt” elevation by as much as 300 to 600 m (984 to 1969 feet) by requiring less oxygen for metabolism.
Initial altitude exposure frequently results in anorexia and subsequently reduces energy and carbohydrate intake.25 Anorexia (and thus food intake) usually improves with time and acclimatization (3 to 7 days at altitude), but, depending on the altitude, may never match that at sea level. Weight loss and performance decrements are quite common under these conditions. Carbohydrate supplementation of the diet at elevations exceeding 2200 m (7218 feet), particularly with carbohydrate-containing beverages, is usually an effective method to increase carbohydrate and total energy intakes.3,25,37 Some,32 but not all,117 studies of carbohydrate supplementation at altitude have demonstrated a decrease in the adverse symptoms resulting from acute altitude exposure. Enhancement of short-term, high-intensity performance,26 as well as long-term, submaximal performance,3,14 by carbohydrate supplementation has also been noted in some studies involving altitude exposure. The beneficial effects of carbohydrate at altitude most likely depend on the type of exercise performed (intensity and duration) and the degree of prior muscle glycogen depletion experienced by the test subject, because of varying degrees of anorexia.
Muscle glycogen is related to the caloric adequacy of an individual’s prior diet; carbohydrate intake usually parallels the overall dietary intake of the antecedent diet.3 It is a good plan to consume a mixed diet with snacks high in carbohydrate. The most effective form of carbohydrate supplementation in environmental extremes is usually liquid beverages; people will drink even when they are reluctant to eat.3,14,25,37 Increasing fluid intake along with carbohydrate intake is also beneficial at altitude, where increased fluid losses occur as the result of diuresis and of respiration in the dry (low-relative-humidity) atmosphere.9
The “Right” Macronutrient Mix for Work at Altitude
There are two schools of thought regarding the most advantageous mixture of dietary macronutrients for work at altitude. Some believe that food preferences change markedly as elevation increases during the climb, and that carbohydrate becomes more palatable to the anorexic appetite. Others believe that once appetite recovers from the initial period of altitude acclimatization, the relative proportions of carbohydrate and fat in the diet are not as important as eating to energy demands to prevent loss of lean body mass. Early work published by Teasdale119 and later advocated by Pugh97 and Consolazio32 favored carbohydrate for work at altitude largely because of its structural oxygen content; carbohydrate is more highly oxidized than is fat or protein and therefore theoretically should require less atmospheric oxygen (which is, in effect, reduced at the reduced barometric pressure at altitude) for its metabolism to CO2 and ATP. This line of reasoning also agrees with what we know about the need for glycogen replenishment. Glycogen stores would be important energy providers for intense physical climbing work at altitude if one was working at a high percentage of . However, as Teasdale119 aptly pointed out in his writings on “The Diet Problem for Mountaineers in the Himalayas,” the actual amount of work in foot-pounds done at altitude is usually self-limited and may be relatively low compared with that at sea level; the real problem is not the amount of energy that is expended but rather oxygen availability. Teasdale119 recommended that climbers should seek a diet “demanding as little oxygen as possible” and his recommendation for accomplishing this was the ingestion of carbohydrate at frequent intervals. He also was one of the first to point out the oft-encountered “vicious” cycle of human physical deterioration at altitude: loss of appetite–partial starvation–metabolism of climbers’ fat stores for energy–ketoacidosis–further anorexia and loss of appetite–loss of weight–deteriorated physical performance. This cycle of events, along with dehydration, is depicted schematically in Figure 69-1. Many of these situational/physiologic turning points leading to physical performance decrements can happen in other extreme environments, but the climber at high altitude seems to experience these adverse factors sooner and to a more significant degree than do workers in other environments. Washburn126 emphasizes that dietary carbohydrate becomes particularly more critical as altitude increases above 10,000 feet, and Consolazio32 identified carbohydrate as an important factor in lessening the initial severity of altitude illness. Although early observations by Pugh97 and subsequent research by other investigators seem to indicate that carbohydrate is better “tolerated” or preferred at altitude, other more recent studies in normobaric101 and hypobaric hypoxic conditions99 have failed to show a carbohydrate “preference” at altitude.12 Reynolds and co-workers99 expected carbohydrate consumption to increase with increasing altitude in a study of climbers on Everest, but surprisingly found that retort pouch packages of high fat sausages seemed to be preferred under cold, high-altitude conditions. This preference could be due to a true preference for fat or simply the ease of utilization of the heat in a pouched food product. However, just because high fat foods gain some acceptance in the cold and perhaps even at altitude does not necessarily mean that carbohydrate loses its importance for glycogen repletion and maintenance of blood glucose levels for periods of high-power energy output. The most important consideration regarding fueling heavy physical work in the cold or in combination of cold and altitude is as Washburn126 puts it, “plenty of good food is of vital importance.” “Good food” that is appetizing served warm is likely to be consumed and therefore subsequently beneficial. Perhaps the real advantage of carbohydrate at altitude is more evident early in the ascent and, as climbers become more acclimated to the altitude, the exact macronutrient composition of the diet may be of lesser importance than individual climber food preferences.
Energy: How Critical Is It?
When planning for a prolonged wilderness outing, the following question should be asked: “If we run short on food, will we suffer severe consequences in our progress along our route and experience difficulty carrying our heavy packs?” For optimal performance, it is total energy intake, especially carbohydrate intake, that is the key for sustaining high-level work capacity for extended periods. However, performance across a broad spectrum of backcountry tasks, including load-bearing work, is not always severely degraded by short periods of suboptimal energy and carbohydrate intake. A review of the effect of energy restriction on military work performance indicated that soldiers can maintain relatively normal work capacities for short time periods (<10 days) of food restriction.39 The Minnesota starvation studies conducted during World War II demonstrated that energy deficits resulting in a loss of less than 10% of body weight did not greatly impair physical performance; however, underconsumption of calories for longer periods producing continued loss of body weight created significant deficits in physical performance,118 as evidenced by the observations of one test subject who participated in the 1570-kcal/day, 24-week semi-starvation study, resulting in 24% body weight loss: “Then came February twelfth, the starting date of semi-starvation … only two meals a day from now on. For the first two weeks the new life was fun. I was losing weight, of course, but I still had lots of energy. Then came the day when I lost my ‘will to activity’—I no longer cared to do anything that required energy.”
The degree of reduction of work capacity depends on the degree of caloric restriction, the carbohydrate content of the food available, and the power output the work demanded. Studies of food restriction in military scenarios have revealed that restricted energy and dietary carbohydrate content over a 30-day period supported light to moderate activity level without evidence of greatly impaired physical performance capabilities.15 On the other hand, longer periods (8 weeks) of caloric restriction coupled with higher levels of energy expenditure (U.S. Army Ranger training) have been associated with significantly reduced physical performance capacity.86
Because studies have varied with regard to experimental conditions and performance measures, it is difficult to draw conclusions about the relationship between energy deficit and performance. Some indicators of performance, such as grip strength, appear to be well preserved until nutritional status is severely compromised. Other measures, such as maximal lift test, maximal jump height, isometric leg extension, and maximal oxygen uptake, appear to be more sensitive predictors of impaired performance.56 In general, in nonobese individuals, strength seems to be rather well maintained at a body weight loss of up to 5%. This may be good news for wilderness travelers who are concurrently on a gradual weight loss program. Shedding fat actually “lightens the load” without diminishing strength. However, when dieting or restricting food results in loss of lean body mass, performance decrements result. Aerobic capacity and strength are reduced when loss of body weight exceeds 10%. Friedl39 reviewed the influence of reduction in body weight resulting from reduced food intake on muscle strength and aerobic capacity and concluded that changes in maximum oxygen consumption () in response to modest caloric restriction generally influence performance to a lesser degree than do reductions in muscle strength in response to weight loss. The primary concern of weight loss due to inadequate energy consumption occurring during extended wilderness activities appears to be loss of muscle strength. Significant loss of muscle strength can be expected to occur following 5% to 10% body weight loss.39 Significant declines in aerobic capacity can also occur following weight losses of this magnitude, but the decline in aerobic capacity appears to have relatively little effect on individual performance at moderate (<50%
) sustainable workload levels.39 In practical terms, this may mean that a gradual trek to the summit may not be precluded by a prior food restriction accompanied by significant loss of body weight, but that a short-term, all-out push for the summit to avoid impending bad weather would most likely be compromised.
Factors other than strength and aerobic capacity should also be considered when evaluating the effects of energy restriction on performance. Although weight losses of 6% or less over periods of 10 to 45 days generally do not produce significant degradations in cognitive performance,108 mood may be adversely affected by caloric restriction.30,79 However, long-term reduced consumption resulting in 50% loss of energy requirements may significantly degrade cognitive performance.77 Reduced food intake, when coupled with other stressors such as high rates of energy expenditure and sleep deprivation, can also impair immune function.54,62,68
Carbohydrates: Critical for High Work Output Performance
Both the length of time provided for dietary adaptation to carbohydrate restriction and the amount of carbohydrates in the diet can influence the level of aerobic endurance performance.2 Aerobic endurance performance can be reduced by 40% after only 4 days on a total-calorie-adequate but low-carbohydrate diet (i.e., with carbohydrates providing 10% of the kilocalories).42 When a diet that was similarly adequate in total calories, but with carbohydrates providing only 5% of the kilocalories, was fed for 2 weeks, performance was also reduced, but only by approximately 15%, presumably because of metabolic adaptations to the shift in energy sources that occur with time.95 Carbohydrate is important to performance, but the impact of any reduction in dietary carbohydrate intake depends on (1) the period of time over which it is reduced, (2) the absolute level of carbohydrate in the diet, and (3) the power output (P = F × D /T) needed to accomplish the task at hand. The latter point regarding power output requirements is an important consideration in the provision of food for wilderness activities. Since much wilderness activity is usually recreational and not competitive, self-paced work and the lowering of power requirements by increasing the time over which the work is accomplished is a feasible approach that can result in less reliance on carbohydrates and more reliance on a “slower burning” fuel, such as fat.
Reduced carbohydrate intake, more than the reduction of any other macronutrient (with the exception of water), has the potential to negatively influence muscle glycogen levels and endurance.2 There is abundant evidence in the sports medicine literature to permit extrapolation to similar wilderness activities and to conclude that certain types of performance, such as backpacking, cross-country skiing, and climbing, may be influenced by an acute shortage of carbohydrates in the diet, depending on the intensity of the workload (% ) in which the individual is engaged. Inadequate carbohydrates in the diet, coupled with successive days of intense prolonged exercise, results in a gradual reduction of glycogen stores, deterioration of performance, and increased perception of fatigue. Perceived or “felt” exertion for certain wilderness activities, such as load-bearing work, may reasonably be assumed to be a function of the dietary carbohydrate intake and its subsequent effect on muscle glycogen levels. To avoid fatigue and extend or enhance performance, carbohydrates may be ingested before, during, and after moderate to heavy intensity aerobic exercise.55 This requires daily consumption of approximately 500 to 600g of carbohydrate (about 60% of the kilocalories in a day’s food). The actual amount of carbohydrates required depends on the individual’s body size and energy needs. To put this into perspective, a review of typical dietary carbohydrate intakes of male soldiers fed a variety of rations during 18 field studies in temperate, hot, and cold environments revealed intakes ranging from 244 to 467 g/day.18 Similar data are not as well documented for nonmilitary wilderness activities, but it is probable that daily carbohydrate intakes would be similar and considerably less than the 500 to 600 g/day recommended for optimal physical performance, because total caloric intake during work in the outdoor environments is often less than that required to maintain energy balance.73 Most people do not selectively consume low-carbohydrate diets during wilderness activities, but total carbohydrate intake is often low because of its relationship to total energy intake and because of limited high-carbohydrate food choices. Some backpackers seek to maximize food and caloric density because of the weight of, and space available in, their packs. Calorie-dense food item choices that achieve this goal are often high in fat and relatively low in carbohydrates. Inadequate food consumption in military field exercises has been ascribed to poor ration palatability and variety, menu boredom, not enough time to eat or prepare meals, anxiety, and intentional dieting to lose weight.73 Similar factors may be operative in wilderness expeditions. Countermeasures to reduce the effect of these factors should be taken into consideration during ration planning. To sustain short-term performance, a shortfall of energy (calories) is not as significant a concern as the lack of carbohydrate.39
Field studies of carbohydrate intake and performance are difficult to conduct because of the many uncontrolled variables encountered outside the laboratory. Therefore, definitive field studies demonstrating a positive effect of dietary carbohydrate supplements on wilderness performance are lacking.6 However, when field conditions can be imitated in well-controlled laboratory settings, the results suggest that carbohydrate supplementation benefits performance. In a study84 conducted using 18 physically fit U.S. Army Special Operation Forces soldiers as test subjects designed to test the concept that soldier performance would benefit from carbohydrate supplementation under conditions simulating field operations, subjects were fed a diet controlled to simulate a “typical” daily dietary intake of carbohydrate and protein encountered during field operations (327 g carbohydrate per day, 201 g fat per day, and 118 g protein per day, furnishing 3657 kcal/day). The soldiers exercised daily (11 days total) under conditions designed to simulate field energy expenditure patterns (intermittent- as well as sustained-activity patterns of varying intensity levels). Each test subject received one of three beverage supplements during the program: placebo (0 g carbohydrate), carbohydrates once a day immediately after exercise (180 g carbohydrate), or carbohydrates divided into several doses taken after the morning exercise session and at intervals during the afternoon exercise session (180 g carbohydrate in divided doses). A drop in the respiratory exchange ratio in all treatments was observed, indicating that the soldiers were experiencing a carbohydrate shortage in response to the field simulation and were switching to fat energy sources to sustain energy expenditure. However, the provision of supplemental carbohydrates permitted a higher level of physical performance or aerobic power to be attained. Run times to exhaustion were increased approximately 6% with the single carbohydrate feeding and 17% with the divided-dose administration. The ingestion pattern of the carbohydrate supplement as well as the carbohydrate itself appeared to influence performance, indicating that a supply of easily consumed carbohydrates (supplement or food) ingested before, during, and after field activities is an effective method to sustain or boost physical performance.
Fat: A Special Place in Wilderness Exploration?
Historically, wilderness explorers and expeditions have relied heavily on foods higher in fat content than current sports nutrition and healthy dietary guidelines recommend. One early 20th-century explorer, Vilhjalmur Stefansson, became such a strong advocate of meat-based high-fat diets that he volunteered himself for an unusual experiment in 1928.67,113 Stefansson consumed an all-meat diet for a year with no detectable adverse medical consequences. Stefansson’s championing of high-fat diets for work in cold regions led to a World War II–era U.S. Army and Canadian test of a high-protein and high-fat pemmican diet (low in carbohydrate) for work in the cold, with less-than-encouraging results.57 Predictable fatigue occurred when the high-fat, low-carbohydrate diet was abruptly thrust on the soldiers. At the time, this study was offered as an argument against including pemmican in the cold-weather military diet. Notwithstanding the military’s lack of enthusiasm for high-fat food, recent arctic explorers such as Will Steger have relied on pemmican for nutritional support during cold-weather polar expeditions.114
Although we now know that a period of time is required for metabolic adaptation to a high-fat diet,92,93 the dispute concerning the suitability of high-fat diets continues. A review of numerous studies and the composition of the diets and experimental conditions under which they were conducted indicates that two factors are critical to the satisfactory utilization of high-fat diets: prior adaptation to fat in the diet and the power or exertion level at which the subjects were required to work.91 Humans permitted to adapt gradually to increasing levels of fat in the diet for approximately 3 weeks can function much better than if the dietary change is abrupt. High-power outputs require the capacity for repeated bursts of anaerobic metabolism, which is best fueled by carbohydrates (i.e., via glucose and glycolysis). Efficient fat metabolism depends on the ready availability of oxygen. The ability to take up oxygen necessary for fat oxidation becomes limiting at high power outputs. Military studies of fat content of the diet confirms that a subcaloric, relatively high-fat diet (1976 kcal/day; 46% fat) can maintain a moderate physical performance (including load-bearing work) for periods of up to 30 days.15,68 Practical physical and situational constraints such as caloric density, weight-per-volume considerations, prior experience, work level intensity, and local availability of food seem to affect the usefulness of relatively high-fat provisions used in support of wilderness expeditions.3,38
Fat, although not an ideal energy source for high power output competitive sporting events, can serve admirably as a concentrated energy source for outdoor activities requiring low power but sustained work performance over longer periods of time. This is because low power but sustained work such a carrying a loaded backpack along a trail at a comfortable pace, can usually be accomplished aerobically at a moderate % effort that can be energetically powered largely by fat oxidation without dipping deeply into glycogen reserves. Although fat has received a somewhat “tarnished” health image over the past 40 years via an imperfectly understood relationship with atherosclerosis and coronary heart disease, we have gradually come to understand that there is a significant interaction between the type of fat (fatty acid composition) and the individual’s habitual level of physical exertion. Simply put (although a bit oversimplified), fat intake becomes particularly problematic when highly saturated fat (e.g., C-16:0 palmitic acid) is consumed habitually without accompanying daily high rates of energy expenditure. Anthropologic support for this concept can be found by examining cardiovascular disease in early Arctic populations that subsisted on a largely meat diet, where fat was a highly prized commodity in comparison. No one would consider classically made pemmican a “health” food, because of its high saturated fat content. Although pemmican is historically considered to be an ideal “trail” food for cold-weather expeditions because of its caloric density and resistance to spoilage, no one advocates a long-term habitual diet based on pemmican. Pemmican consumption is largely self-limited—acceptable for its purpose in the backcountry for defined lengths of time but not acceptable as a staple in daily everyday life. There is little scientific evidence that short periods (usually less than 1 month) of a high-fat diet has an irreversible effect on cardiovascular health, although a single high-fat meal can acutely influence endothelial response to the meal ingestion, causing a narrowing of the arteries supplying blood to the extremities.85 We project from observations such as this and epidemiologic studies linking long-term high dietary fat intakes to increased incidence of heart disease that repeated insults of this nature may ultimately lead to increased deposition of lipid in the intima of arteries.22,110 All indications, however, lead to the conclusion that we really do not know the time course required for high-fat diets to take a toll on our arteries, particularly if the periods of high fat consumption are intermittently imposed and further complicated by the level of caloric expenditure during the periods of high fat consumption, as well as the type of fat consumed. The amount and type of fat in wilderness provisions remains largely a personal choice balanced against logistic constraints and the requirement for caloric density.
Washburn126 stated that diets consisting of high levels of fat can be tolerated well by individuals doing heavy exercise in extreme, cold environments. He also wrote that diets for work in cold weather at low altitude can rely heavily on fats for energy. Because the usual diet of most humans now is generally higher in carbohydrates than fats, it is advisable that a gradual transition to a higher proportion of calories in the diet from fat be accomplished over a period of a few weeks prior to embarking on an expedition. Enzymic adaptation to a higher-fat diet can help prepare the individual to optimally metabolize and utilize increased fat in the diet. Drury and associates34 studied the metabolic effects of a diet high in fat and protein under cold weather field conditions and found that there is distinct adaptation to diets of this nature. Initially, blood glucose levels drop sharply because of the low amount of carbohydrate in the diet, but level out in the low normal range after 2 to 3 days. Ketone excretion rises over the same time period in response to the high levels of fat being oxidized for energy, peaking about the same time (3 days) as when glucose decrease levels out, and returns to normal levels after about 9 days. Two weeks should be considered the minimum amount of time to adapt to a high fat–low carbohydrate diet prior to utilizing it as the main daily food routine during expeditions.92
Early explorers utilized pemmican and other high fat–high protein foods as staples for their expeditions, partially from observations of the dietary patterns of native people and partially from logistic considerations of weight/volume and perishabilty. Evidence that pemmican is not just an “antiquated dietary anachronism” has been demonstrated by the modern day use of pemmican by Arctic adventurers Several food supply houses still manufacture and supply various versions of pemmican to outdoor enthusiasts. Those desiring to prepare their own pemmican can do so quite easily using common kitchen food processor and readily available supermarket products. Numerous Internet guides to pemmican making can be easily accessed, such as http://www.traditionaltx.us/images/PEMMICAN.pdf. Pemmican is energy dense, well preserved without refrigeration, and can be a versatile energy source, especially on long days on the trail when there is not a large amount of time or daylight for leisurely food preparation. It lends itself well as a cold snack on the trail. When added to a pot of hot water and mixed with a nutritious grain such as quinoa or brown rice, it becomes a hot filling meal (generically referred to by Arctic explorers as “hoosh”) that can provide protein, fat, and some carbohydrate at the end of a hard day. Table 69-1 lists some nutritious carbohydrate grains that compliment a meal of pemmican “hoosh.”
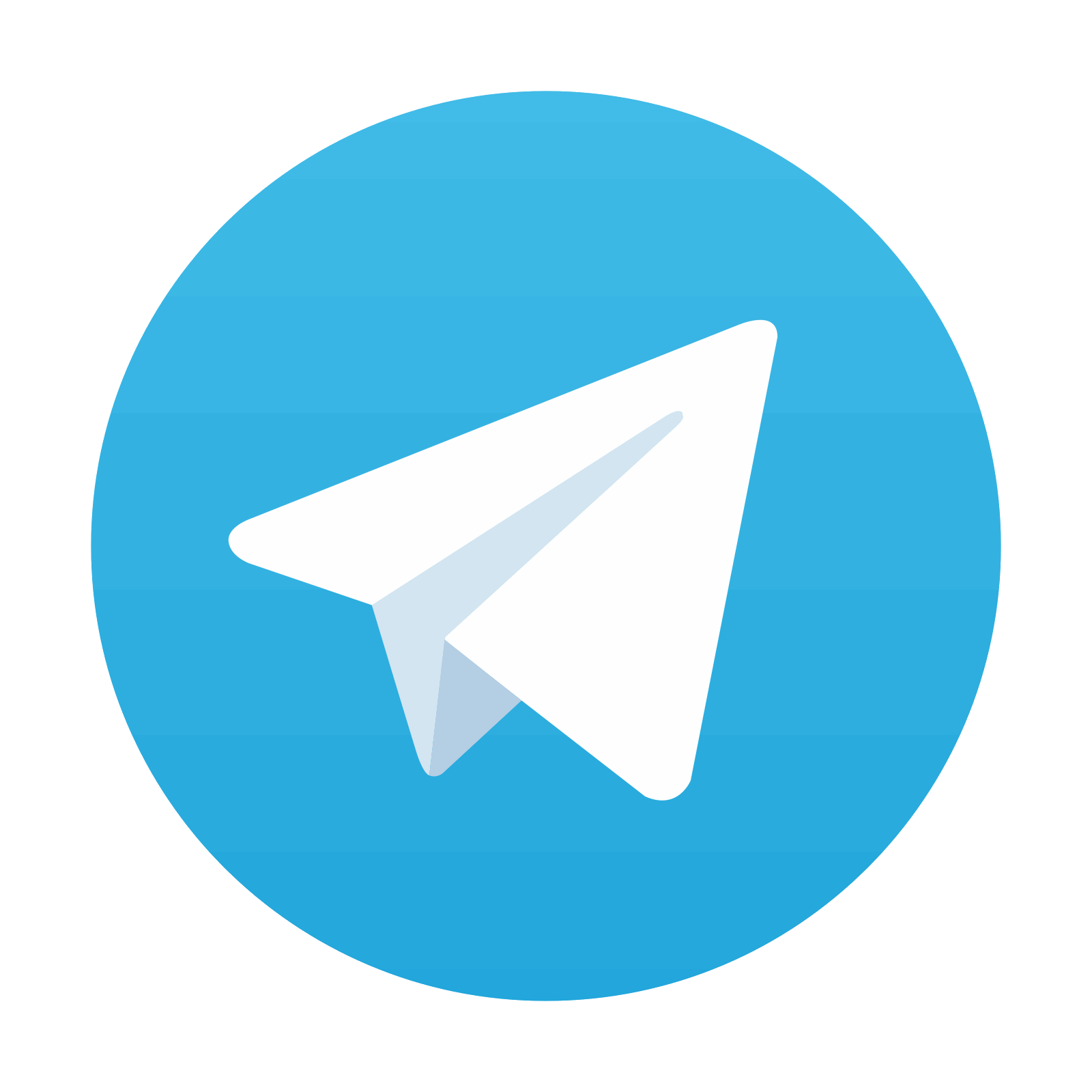
Stay updated, free articles. Join our Telegram channel

Full access? Get Clinical Tree
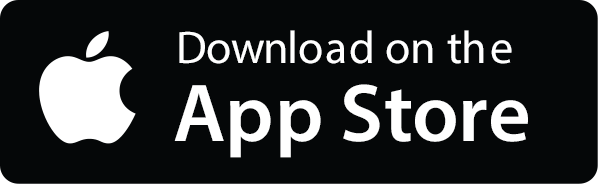
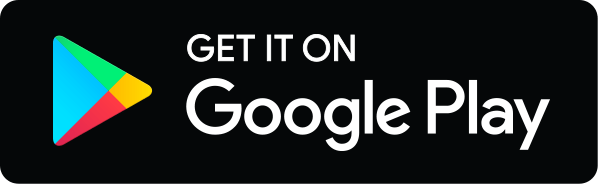