Chapter 9 Nucleus Pulposus Replacement and Motion-Sparing Technologies












Anatomy
The intervertebral disc consists of three components: an inner gelatinous NP, an outer annulus fibrosus (AF), and vertebral endplates (EPs) located superiorly and inferiorly. The AF is a circular layer consisting of concentric lamellae that are resistant to tensile strength; its tensile strength resistance is caused by an abundance of type I collagen. In these lamellae, collagen fibers run obliquely between the lamellae of the annulus in alternating directions. The NP consists of proteoglycan and water gel held together loosely by an irregular network of fine type II collagen and elastin fibers. The major proteoglycan of the NP is aggrecan,1 which provides the osmotic properties needed to resist compression. The intervertebral disc is avascular, and consequently it is nourished through the vessels in the subchondral bone adjacent to the hyaline cartilage of the EP by passive diffusion.2 Cells of the nucleus and inner annulus are supplied by the blood vessels of the vertebral body and the distinct capillary network that penetrates the subchondral plate. The nutrients then diffuse from these capillaries across the cartilaginous EPs and through the dense NP to the target cells.3
NP and AF cells maintain the normal metabolism of the discs by controlling production of cytokines, enzymes, and growth factors. NP cells produce proteoglycan and collagen. A monomer of proteoglycan consists of a protein core linked to extended polysaccharide chains such as keratan sulphate and chondroitin sulphate. Each monomer is bound to hyaluronic acid by a link protein. Aggrecan represents about 70% of the NP and 25% of the AF. It has high osmotic pressure arising from the water retained within the NP and tends to inflate the collagen framework.4 This balance is responsible for the ability of the disc to retain fluid within the matrix, resulting in high viscoelasticity. The high concentration of aggrecan and its associated fluid enable the tissue to support compressive loads without collapsing; through this mechanism, loads are transferred equally to the AF and vertebral body during the flexion and extension of the spine.5
The equilibrium of water content also depends on the loads applied to the intervertebral disc; when loads are applied to the disc, the water of the NP is directed to the adjacent EPs; the converse occurs after unloading. This pumping mechanism enhances delivery of the nutrients to the nucleus.6
Disc degeneration in humans can occur as early as the third decade of life.7 However, disc degeneration does not always cause pain. In one study, degeneration appeared in at least one intervertebral disc in 35% of subjects between 20 and 39 years of age, and in all of the study subjects 60 to 80 years of age.8 Aging, obesity, smoking, vibration from transportation, excessive axial loads, and other factors may accelerate degeneration of intervertebral discs.9,10 The degree of disc degeneration appears to be correlated with the patient’s age. Although many studies have been carried out to show such a relationship, no distinct cause has been established.11,12> Although all of a person’s discs are the same age, discs in the lower lumbar segments are more vulnerable to degenerative changes than those in upper lumbar segments, suggesting a prevalent role of mechanical loading over aging.13
Cervical disc degeneration, typically associated with the development of calcified disc herniation, is a slower process than that which occurs in the lumbar spine, where frank disc herniation or disc degeneration occurs. Damage to the AF that occurs in progressive disc degeneration or after surgical discectomy causes a gradual loss in disc height, leading to changes in the biomechanical characteristics of the remaining disc.14 Such mechanical changes may ultimately place additional stress on the facet joints and lead to circumferential spinal segment degenerative changes.15–17
Several other factors have been reported to cause disc degeneration. Growth factors such as transforming growth factor, insulin-like growth factor, and basic fibroblast growth factor stimulate the NP or AF cells to produce more extracellular matrix and inhibit the production of matrix metalloproteinases.18 Since an increase in the level of the basic fibroblast growth factor and transforming growth factor promotes the repair of the degraded matrix,19–21 it is likely that a decrease in the concentration of these growth factors is associated with degeneration of the intervertebral disc. Decreased anabolism or increased catabolism of senescent cells also may promote degeneration.22
Basic Science
Artificial intervertebral disc characteristics should resemble those of native discs biomechanically to preserve both segmental motion and global stability. The vertebral column consists of 24 separate vertebrae and the sacrum, connected by a complex system of facet joints, intervertebral discs, ligaments, and muscles. Simple replacement of one section of the vertebral column may adversely affect the entire system. It is estimated that the spine undergoes approximately 100 million flexion cycles in a lifetime,23 not taking into account the slight motions that occur with breathing, estimated to be about 6 million a year.24 Thirty million cycles appear to be the optimal life span of an implant, and 10 million cycles are considered the minimum.25
Design goals for successful disc replacement prostheses include approximation of size and motion of a physiological disc to avoid distraction or overloading of the facet joints. Physiological motion is complex and unlikely to be replicated by a simple hinge joint with a fixed center of rotation. The intervertebral disc joint controls the majority of range of motion (ROM) in compression, flexion, and lateral bending; whereas the facet joints dictate axial rotation and extension. During spinal flexion almost all of the resistance comes from the anterior column. In the lumbar spine, normal discs allow 8 to 13 degrees of ROM at each level.26 The total ROM for lateral bending (right to left) at each level is also significant; approximately 10 degrees for upper lumbar discs and approximately half of that for lower lumbar discs. This lateral bending is resisted primarily by the disc. Because of the resistance from the facet joints, the ROM for extension and axial rotation are comparatively less than the ROM for other movements.
Because of the requirement for stability and mobility, lumbar spinal movement is complex. Translation and rotation are possible in three orthogonal planes.27 The disc is the main constraint to motion, but it also allows a certain amount of movement.28 In upper lumbar motion segments, lateral bending is the predominant motion. In the lower lumbar spine and lumbosacral motion segment, flexion and extension are the prevalent motions.29 In addition, 2 to 4 mm of anterior translation in the sagittal plane is normal for the lumbar spine vertebrae.27 Finally, the center of rotation for each lumbar motion segment changes with flexion, lateral bending, and rotation.30 In addition, the movements of axial rotation and lateral bending are coupled in the lumbar spine.31 Physiological lordosis allows the facet joints in the lumbar spine to bear axial loads (up to 16% in some studies).32
The cervical spine is the most mobile segment of the spine. Total cervical spinal movement ranges between 130 and 145 degrees of flexion and extension, 90 degrees of lateral flexion, and 160 to 180 degrees of axial rotation bilaterally. Sagittal flexion-extension motion is mainly sustained by the C0-C2 segment, whereas the other cervical spinal segments are only responsible for a few degrees of segmental ROM (around 10 to 20 degrees per level).33,34 In addition, the segmental and global cervical spinal ROM decreases with increasing age; in fact, significant loss of movement in all planes is found in healthy asymptomatic individuals between 50 and 54 years of age compared with those between 35 and 39 years of age.35 ROM is also inversely affected by increase in body weight and decrease in the level of physical activity.36 Moreover, a healthy cervical spine moves more than one afflicted with degenerative disc disease or radicular compression.37,38 Consequently, immobilization of a single spinal segment seems to have little effect on the global ROM of the cervical spine, especially in patients in whom the spine was stiff before surgery. Further studies are required to better understand cervical spine kinetics and to attempt to reproduce physiological ROM with cervical spine arthroplasty (CSA).39,40
When considering replacement of the NP, implants are intended to replace the extruded or surgically removed intervertebral disc or the degenerated disc before definitive collapse. The principal biomechanical function of the NP prosthesis is to maintain or restore the physiological height of the intervertebral disc space, the mobility and load-bearing function of the spine, and the normal mechanical behavior of the AF. It has been demonstrated that removal of the NP is associated with an increase in spinal mobility ranging between 38% and 100%.41 Moreover, removal of the nucleus leads to outward bulging of the outer region of the annulus and bulging of its inner region toward the center of the disc with axial loading.42 These factors cause circumferential tears in the AF and may further decrease annular ability to resist shearing forces. Nuclear replacement procedures are intended to restore the biomechanical function of the annulus by placing annular fibers under tension. To achieve this result, the nucleus replacement device must maintain and recreate the functional characteristics of the NP. Furthermore, the same device should be able to bear a considerable load before it fails; implant components should have a stiffness similar to that of a native disc to avoid stress shielding, atrophy, and bone resorption that may lead to subsidence and extrusion of an implant.43 It is of utmost importance that the modulus of the component material is comparable to the vertebral EPs because modular mismatches can lead to EP subsidence, especially if the stresses applied to the implant are greater than the strength of the bone. Conversely, if the stresses are lower, the changes in stress distribution may result in the remodeling of the vertebral body.44
Indications/Contraindications
The indication for NP replacement is lumbar discogenic back pain unresponsive to active conservative treatment for a minimum of 6 months.43 Klara and Ray45 described the indications for the prosthetic disc nucleus (PDN) device as back pain, with or without leg pain, related to degenerative disc disease that is refractory to nonoperative treatment. Patients with a disc space height of less than 5 mm were excluded because of the difficulty of implanting the device in such a narrowed disc space. It appears that such implants could be helpful in managing the pain from very early stages of symptomatic disc disruption or as an adjunct to discectomy to maintain disc space height and motion at the surgical level. Additional selection criteria include: posterior disc height of more than 5 mm (because of the implantation device height currently available); a symptomatic pathology restricted to one level between L2 and S1; an age range between 18 and 65 years; and, finally, the absence of any associated severe vertebral pathology. Some exclusion criteria are: previous spinal surgery; spinal problems at more than one level; globular disc; central disc herniation; pronounced Schmorl’s nodules at the involved level; EP lesions; significant spinal, foraminal, or lateral recess stenosis; symptomatic degenerated facet joints; degenerative spondylolisthesis greater than grade I; lytic spondylolisthesis; severe osteoporosis; bone tumors or congenital bony abnormalities; active infection; and the presence of malignant tumors.46
CSA is currently indicated in the treatment of radiculopathy and myelopathy at one or two levels and, although unproven, is used by some of the authors in the treatment of three or more symptomatic disc levels or levels adjacent to an arthrodesis. Arthroplasty use in the management of axial pain presumed secondary to disc degeneration is unknown but would not be expected to be better than fusion. More data are required to specifically expand the clinical indications. Contraindications are instability, significant facet arthrosis, osteomyelitis, and infections. Patients with spondylosis and preexisting ankylosis may not retain motion and may benefit significantly from joint arthroplasty. In the current prospective studies, lumbar arthroplasty is performed to manage chronic severe disabling discogenic low back pain isolated to one or two levels that has been unsuccessfully treated without surgery for a minimum of 6 months. In addition, the spine should be stable and free from significant facet arthrosis. Patients should be able to tolerate an anterior approach. Contraindications include significant psychosocial symptoms, obesity greater than two standard deviations above the bodies mass index, osteoporosis, and infection. Nuclear replacement has a more limited role. It is thought to be indicated early in the degenerative cascade before significant loss of height and EP changes.47
Current contraindications that can be considered common to both the cervical or lumbar spine surgeries include: arthritis of facet joints, preoperative instability, systemic diseases (osteoporosis and inflammatory diseases), previous posterior surgery, advanced age, and ossification of the posterior longitudinal ligament. Currently there is a debate regarding the role of CSA in the presence of myelopathy. Many of the authors still use these devices in patients with either myelopathy or radiculopathy, but in some cases clinical outcomes in patients with myelopathy are not as good as in radiculopathy patients. Nonetheless, randomized clinical studies are required to address this issue.33
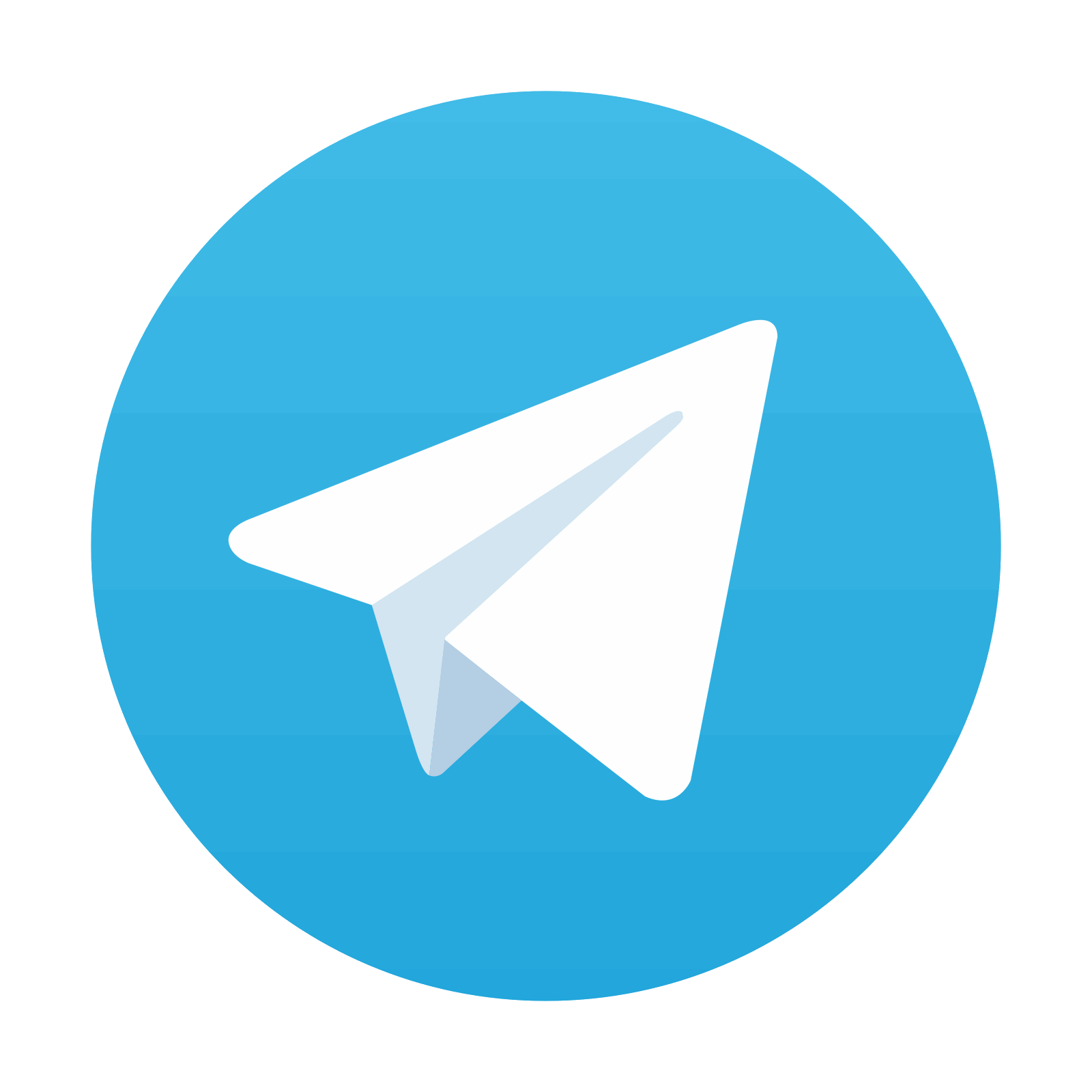
Stay updated, free articles. Join our Telegram channel

Full access? Get Clinical Tree
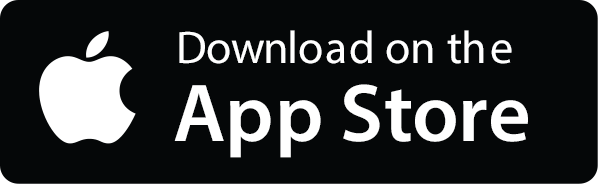
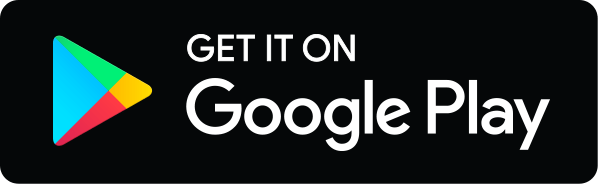