The events of September 11, 2001, and the worldwide proliferation of nuclear materials, including theft and smuggling, increase the possibility that a nuclear disaster may occur from the terrorist use of radiation as a weapon. There are two documented incidents of the threatened use of radiation as a terrorist weapon thus far, both of which occurred in Russia. In 1995 Chechen insurgents buried a cesium-137 “dirty bomb” in a Moscow park and alerted the media before it was detonated. In 1998 a container of radioactive materials was found attached to an explosive mine near a railroad line in Chechnya.
The threat of a nuclear terrorist attack with a nuclear device or radioactive materials is not a question of if, but when. Nuclear disasters can have different scenarios. An extreme situation would be the threat of a terrorist attack using a low-yield 10-kiloton nuclear weapon. Many people have become aware of the threat of such an attack because of increased media attention. Successful planning for a nuclear attack or accident is imperative.
This chapter presents important concepts of nuclear disaster management. The aim is to offer a basic understanding of the threat and effect of nuclear disasters, including accidents and terrorist events. This chapter provides a brief historical overview of the development of the nuclear era, the nuclear arms race, and present concerns. It highlights some basic physical and biological principles of radiation injury that are commonly misunderstood by health care professionals. The chapter reviews various nuclear disaster scenarios, summarizes medical management principles, and underscores obstacles to nuclear disaster management.
Historical perspective
Following the discovery of radioactivity in 1896, the understanding of atomic physics increased rapidly in the early twentieth century. Ernest Rutherford is recognized as the father of nuclear physics. In 1908 he received the Nobel Prize for chemistry for his “investigations into the disintegration of the elements, and the chemistry of radioactive substances.” He was directly responsible for training many physicists at Cambridge University in England. In the latter capacity, he was also indirectly responsible for much of the nuclear physics research that was being conducted at many universities by his former students throughout the world and for the development of the nuclear era.
In 1938 Hahn and Strassmann in Berlin described the phenomenon of nuclear fission. They determined that when uranium was bombarded with neutrons, the nucleus split into two parts of comparable mass with the release of an enormous quantity of energy. This observation would lead to the Manhattan Project and eventually to the production of the atomic bomb (A-bomb). In 1942 Fermi achieved the first controlled self-sustaining nuclear reaction. In August 1945 the first A-bombs (or fission bombs) were detonated over Hiroshima and Nagasaki, Japan.
The development of the A-bomb would lead ultimately to the development of the even more devastating hydrogen bomb (H-bomb). In the 1950s, justification of work on the H-bomb (fusion or thermonuclear bomb) was based on the fact that it produced less radioactive fallout and therefore was considered a “cleaner” nuclear weapon. Enhanced radiation weapons (neutron bombs) were later developed to minimize the effects of blast and heat on buildings and to maximize the effects of the neutrons on living organisms (e.g., to kill tank crews without causing much collateral damage).
During the Cold War era, the nuclear arms race between the United States and the former Soviet Union continued for more than 30 years. By the early 1980s the number of nuclear warheads worldwide was estimated to be in excess of 40,000. The explosive power of these warheads ranged from about 100 tons up to more than 200 million tons equivalent of high explosives. During this same time period the total strength of nuclear arsenals was believed to have been equivalent to about a million Hiroshima bombs or 13,000 million tons of trinitrotoluene (TNT).
With the end of the Cold War in the late 1980s, the threat of Armageddon—a nuclear war between the United States and the former Soviet Union in which 200 million Americans might not survive—was dramatically reduced. However, this apparent relief from the Armageddon syndrome has been short-lived because the twenty-first century now appears to be more dangerous and less predictable. The risk that someone might detonate a nuclear weapon in anger or retribution is probably greater than at any other time since the 1962 Cuban missile crisis. Moreover, nuclear weapons ambitions are spreading not only to states, but also to terrorist groups. Osama bin Laden talked of acquiring nuclear weapons as a “religious duty.” By 2000 some 32,000 nuclear weapons were still being deployed and posed new threats from some terrorist groups.
Today, the most likely threat from nuclear weapons is based on a scenario of a terrorist group or rogue nation using a single, low-yield device, such as a suitcase-size tactical nuclear weapon. The second most likely threat is from an improvised nuclear device (IND) used by terrorists and composed of either weapons-grade (enriched) uranium or plutonium-239. The third most likely terrorist nuclear threat is from a radiological dispersal device (RDD), also known as a “dirty bomb.” (See Chapter 77 for a more complete discussion of the RDD.) Although the detonation of an RDD does not produce a nuclear yield, RDDs are important because of their potential to cause a nuclear disaster using high explosives to disperse very high levels of radioactivity associated with materials from nuclear reactors, spent fuel storage depots, nuclear fuel reprocessing facilities, high-level nuclear waste sites, or transport vehicles.
The development of human-made radioactive isotopes and nuclear weapons during and after World War II was an important factor in the birth of the nuclear weapons industry in the mid-twentieth century. Most of what we know today about nuclear disasters comes from the A-bomb experience of World War II and from radiation accidents that have occurred over the past 70 years. Information on the effects of nuclear weapons has been obtained from Hiroshima and Nagasaki and from animal experimentation conducted during the atmospheric tests of the 1950s. Data on the consequences of radiation accidents have been obtained from the methodical medical and scientific assessments of radiation accident victims. The existence of national and international registries on accidental radiation injuries has helped to characterize the types and patterns of radiation damage and to provide useful information for optimal medical management of victims. The knowledge gained from these experiences serves as the foundation of nuclear disaster management for the twenty-first century.
Current practice
Nuclear weapons produce their biological effects through the direct or indirect effects of blast, heat, and radiation. The first two effects are fairly simple and the mechanisms of their injury are readily understood. Radiation, on the other hand, is a more complex noxious agent and is generally misunderstood by health professionals. Understanding how radiation interacts with matter provides insights into methods used to detect and measure radiation, reduce the biological hazards, and manage nuclear disasters and their consequences.
Basic Principles
Radiation Physics
After a nuclear weapon detonation, a tremendous amount of energy is quickly released in the form of ionizing radiation. Ionizing radiations are defined as those “types of particulate and electromagnetic radiations that interact with matter and either directly or indirectly form ion pairs.” Ionizing radiations can be divided into two categories: directly ionizing and indirectly ionizing. Directly ionizing radiations are charged particles (electrons, protons, beta particles, alpha particles, etc.) that have sufficient kinetic energy to produce ionizations through direct collisions with bound orbital electrons of an atom or molecule. Indirectly ionizing radiations are uncharged particles or photons (neutrons, x-rays, gamma rays, etc.) that can liberate bound orbital electrons, but only indirectly.
Both x-rays and gamma rays are photons, or small quantized packets of pure electromagnetic energy, just like light. However, they have a much higher nonvisible frequency. X-rays and gamma rays differ only in their origin. X-rays are produced in the outer orbital shells of atoms, whereas gamma rays come from within the nucleus of the atoms. X-rays and gamma rays are low linear energy transfer radiations; that is, they deposit relatively low amounts of energy along the track of radiation as they penetrate tissue and deposit energy deep in the body. Many radioactive isotopes decay by emitting several different types of particles. The most common forms of particle radiation are alpha, beta, and neutron.
Alpha particles are massive, charged helium nuclei. Because they deposit all of their energy over a very short distance, alpha particles are classified as high linear energy transfer radiation. Because of their size, alpha particles can easily be stopped using a sheet of paper or light clothing. Beta particles are very lightly charged high-speed electron particles that can penetrate to a depth of a few centimeters. Neutron radiation is an uncharged particle that is emitted during a nuclear detonation almost exclusively from the fission and fusion processes themselves. Important factors that will prevent or minimize the effects of radiation exposure on humans include the principles of time, distance, and shielding. (See Chapter 105 for a discussion of radiation safety.)
Radiation Biology
Radiation biology is the field of study that describes the many changes that radiation produces in biological material. Charged particles (e.g., electrons, protons, beta particles, alpha particles, fission fragments) are directly ionizing because they can disrupt (ionize) chemical bonds and produce chemical and biological changes. Electromagnetic radiations (e.g., x-rays, gamma rays) are indirectly ionizing because they do not themselves cause any chemical or biological changes. Instead, they deposit kinetic energy into the material through which they pass and produce secondary charged particles (electrons) that can produce subsequent chemical and biological alterations. Radiation can damage a cell by indirectly interacting with water molecules in the body. This leads to the creation of unstable, toxic hyperoxide molecules (i.e., free radicals) that then damage sensitive molecules and subcellular structures. Ionizing radiations transfer energy to biological material by two mechanisms: ionization and excitation.
Alpha radiation is fully absorbed within the first millimeter of exposed skin. It is generally not an external radiation hazard. Alpha radiation poses serious health hazards if it is inhaled, ingested, or deposited in an open wound. If an alpha-emitting radioactive material is internally deposited, all of the radiation energy will be absorbed in a very small volume of tissue immediately surrounding each particle of material. The internal deposition of alpha particles can cause radiation injury over a long period. If a beta-emitting radioactive material is on the surface of the skin, the ensuing beta radiation causes damage to the basal stratum of the skin and produces deep “beta” burns, or lesions similar to superficial thermal burns. If the beta material is incorporated internally into the body, the beta radiation can cause considerable injury. Gamma radiation is highly energetic and penetrating and therefore is both an internal and external radiation hazard. Gamma rays can cause whole-body injury. Neutron radiation is extremely penetrating and can also result in whole-body irradiation. Compared with gamma rays, neutrons can cause 20 times more damage to tissues.
The sensitivity of cells, tissues, and organs to a dose of ionizing radiation depends primarily on two factors: the rapidity of cell division and the radiosensitivity of the cells. Cells are most sensitive to radiation during mitosis, when DNA is being divided. Rapidly dividing cells are more radiosensitive. Tissues and organs that depend on an active stem cell pool will be more radiosensitive than those tissues and organ systems made up of mature cellular pools with little or no stem cell activity.
Radiation Dose and Units
Quantities of radiation can be expressed in several different ways. The term radiation exposure applies to air only and is a measure of the amount of ionization produced by x-ray and gamma radiation in air. It is measured in coulombs per kilogram (C/kg) of dry air. The roentgen (R) is an older unit of radiation exposure and applies only to x-rays and gamma rays up to energies of about 3 MeV (1 R = 2.58 × 10 − 4 C/kg).
The radiation absorbed dose is the amount of energy deposited in a given mass of absorbing material. The conventional unit for absorbed dose, the rad , is equal to the absorption of 100 ergs of energy in 1 g of absorbing medium, typically tissue (1 rad = 100 ergs/g of medium). The International System of Units (SI) unit of absorbed dose, the gray (Gy), is defined as the absorption of 1 J of energy per kilogram of medium (1 Gy = 1 J/kg = 100 rad and 1 rad = 1 cGy).
The term dose equivalent is necessary because different radiations produce different amounts of biological damage. It is defined as the product of the absorbed dose and a factor, Q (the quality factor), that characterizes the damage associated with each type of radiation. In the conventional system, the unit of dose equivalent is the rem , which is calculated from the absorbed dose (rem = rad × Q). The SI unit of dose equivalent is the sievert (Sv), and Sv = Gy × Q.
Radioactivity is the activity level of a radioactive isotope expressed as the number of atoms that will disintegrate (decay) per second. One becquerel (Bq) is equal to one nuclear transformation per second. The unit in the traditional system is curie (Ci), where 1 Ci = 3.7 × 10 10 Bq. The term dose rate is the dose of radiation per unit of time.
Nuclear Disaster Scenarios
In light of the terrorist attacks of September 11, 2001, it is important to understand possible nuclear disaster scenarios. September 11 was a “day of unprecedented shock and suffering in the history of the United States. The nation was unprepared.” The greatest threat we face in the world today is from nuclear terrorism. The vast stockpiles of nuclear weapons and highly enriched uranium and plutonium in Russia are vulnerable to theft and illicit trafficking. Russia remains the most probable source for a terrorist to obtain a nuclear weapon or materials to construct a weapon, followed by Pakistan and North Korea. North Korea is believed to be the world’s most promiscuous nuclear proliferator. The leaking of weapons technologies allows smaller terrorist groups to wreak havoc and cause greater destruction. Globalization has made it easier for terrorists to travel, communicate, and transport weapons. There is a burgeoning and well-organized black market for nuclear technologies and arms. September 11 was a wake-up call to America. Americans fear more terrorist attacks and more Middle East instability. In fact, a poll taken in 2003 found that 4 of every 10 Americans say that they “often worry about the chances of a nuclear attack by terrorists.” The threats of terrorist attack will be far more serious, for example, in light of the possibility that North Korea has already developed a nuclear program.
Detonation of Nuclear Weapons
Strategic nuclear weapons
Strategic nuclear weapons are devices constructed by a government or country. This category of arms made up the main strategic arsenal during the height of the Cold War. These weapons generally have a yield (or size) from 170 kilotons to 24 megatons. Interestingly, the Soviets are reported to have had some warheads with yields as high as 100 megatons. Strategic nuclear weapons could be carried to the intended target by various delivery vehicles, including land-based intercontinental ballistic missiles, submarine-launched ballistic missiles, or long-range bombers. The detonation of a strategic nuclear weapon in the United States today is unlikely. However, the risk of such an event would most likely be associated with the accidental launch of a former Soviet Union nuclear weapon resulting from equipment malfunction or warning system failure, or dissidents getting hold of strategic weapons and launching them.
Tactical nuclear weapons
Tactical nuclear weapons are also fabricated by a government or country and have yields from less than 0.1 to more than 100 kilotons. These nuclear systems could be used by a military organization for employment against, for example, military targets in a theater of war. Such weapons are artillery shells, ground-mobile rockets and missiles, and air-launched bombs. Tactical nuclear weapons may be the size of a suitcase or backpack.
Imagine a terrorist scenario in which a 10-kiloton nuclear weapon is stolen from a Russian arsenal and detonated in New York City, San Francisco, Houston, Washington, Chicago, or Los Angeles. If a terrorist group, such as Al Qaeda, rented a van and carried this nuclear weapon into the middle of Times Square in New York and detonated it next to the Morgan Stanley headquarters on Broadway, Times Square would vanish within a second. The resulting fireball and blast wave would destroy instantly the theater district, the New York Times building, Grand Central Station, and every other building within a third of a mile from “ground zero.” The resulting firestorm would engulf Rockefeller Center, Carnegie Hall, the Empire State Building, and Madison Square Garden. On a typical workday, more than 500,000 people may crowd into an area within a half-mile radius of Times Square. A noontime detonation could potentially kill all of these people, including hundreds of thousands of others who would die from collapsing buildings, fire, and fallout. The electromagnetic pulse from the blast would destroy phones, radios, and other electronic communications. Emergency medical services and hospitals would be overwhelmed by the injured.
Improvised nuclear device
An IND is fabricated by a nongovernmental organization or terrorist group. It is made up of either highly enriched uranium or plutonium-239. Tens of thousands of potential weapons (softball-size lumps of highly enriched uranium and plutonium) remain today in unsecured storage facilities in Russia, vulnerable to theft by criminals who could sell them to terrorists. Since the collapse of the former Soviet Union, there have been hundreds of confirmed cases of successful theft of nuclear materials. If successful, detonation of an IND could produce a nuclear yield similar to that of Hiroshima, with similar releases of radiation, blast, thermal pulses, and considerable radioactive fallout. Fabrication of such a device would be difficult because of the sophisticated expertise and engineering required. A terrorist organization might be able to produce a partial yield, producing less effect.
Effects of Nuclear Weapons
In this section, the basic characteristics of the effects of nuclear weapons are summarized, with an emphasis on the information of greatest use to nuclear disaster managers. There are numerous other references that treat this subject in much greater detail. , , ,
The main physical effects of nuclear weapons are blast, thermal radiation (heat), and nuclear radiation. These effects depend on the yield of the weapon, physical design of the weapon, and method of employment. The altitude at which a weapon is detonated will determine the relative effects of blast, heat, and nuclear radiation. Nuclear detonations are usually classified as airbursts, surface bursts, or high-altitude bursts.
The blast and thermal effects of a detonation produce the greatest number of immediate human casualties. Immediately after detonation the temperature at the center of the fireball reaches 1,000,000 °C, producing a shock wave traveling at supersonic speeds, followed by hurricane-force afterwinds and an intense flash of thermal radiation. A 5-psi blast wave and 160-mph blast winds associated with the blast wave would destroy a two-story brick house, rupture the human eardrum, or hurl a person against stationary structures. A pressure level of 15 psi could produce serious intrathoracic injuries, interstitial hemorrhage, edema, and air emboli as well as serious abdominal injuries, such as hepatic and splenic rupture.
The thermal radiation is associated with burns, including flash burns and flame burns , and certain eye injuries, including flash blindness and retinal burns . Flash burns result from the skin’s exposure to a large quantity of thermal energy in a very short time, leaving the affected skin with a charred appearance. Flame burns result from the contact with a conventional fire, such as that on clothing. Flash blindness is a temporary condition; however, a retinal burn that results from looking directly at the fireball causes a permanent blind spot.
Detonation of a nuclear weapon produces a variety of nuclear radiations. Initial radiation consists of neutrons and gamma rays produced within the first minute after detonation. The main hazard from initial radiation is acute external whole-body irradiation by neutrons and gamma rays. Residual radiation is generated beyond the first minute after detonation and includes gamma rays, beta particles, and alpha particles. These radiations are produced as highly radioactive fission fragments and activated weapons material. The main hazard from residual radiation is radioactive fallout, which can remain a significant biological hazard long after detonation.
Attacks on Nuclear Reactors
The potential of terrorist attack on a nuclear power plant has been mentioned by the media and is discussed in more detail in Chapters 168 and 194 . However, the probability of an attack is low. The low probability is due to the high security surrounding a nuclear reactor and the safety systems incorporated into it. There is extensive shielding around the reactor core and large amounts of explosives would be needed to breach the reactor core. If an accident occurs, reactors are designed to slow down and stop the reaction. A reactor coolant system does contain some radioactivity, which could be released if the coolant system were damaged. Radioactive iodine and noble gases would probably be released. There would be immediate health effects local to the release. The release of large amounts of radioactive iodine could have long-term effects, such as thyroid cancer in children. In fact, this is exactly what happened after the Fukushima nuclear power plant accident, where large amounts of iodine-131, cesium-134, and cesium-137 were released into the environment. It is possible that a jumbo jet could crash into a reactor or adjacent spent fuel storage facilities, but it would be difficult in the latter situation to expose a large population to radiation from spent fuel rods. Recent computer modeling and engineering studies suggest that the construction of most reactors could withstand a direct hit from a commercial aircraft flying into a reactor at less than 300 mph. However, some scientists question these findings and believe that penetration of the containment dome could cause the reactor to melt down. The 1986 Chernobyl and 2011 Fukushima nuclear power plant accidents serve as harsh reminders of scenarios in which terrorists might possibly cause a nuclear disaster by breaching a nuclear reactor and releasing radioactive materials into the environment.
Detonation of a Radiological Dispersal Device
An RDD is a device that combines radioactive materials with conventional explosives. (See Chapter 77 for a more complete discussion of RDDs.) It may be made from traditional dynamite, TNT, ammonium nitrate, or a variety of other explosive materials. When detonated, an RDD kills or injures through the initial blast, which causes damage from the expansion of hot gases, and by dispersing radioactive materials that are highly toxic over a wide geographic area without a nuclear explosion. Some common radioactive sources that have a high probability of being used in an RDD include cobalt-60, strontium-90, cesium-137, iridium-192, radium-226, plutonium-238, americium-241, and californium-252. The dispersal effects of an RDD depend on the amount of explosives, the physical form of the radioactive source, and the atmospheric conditions. RDDs are attractive to terrorists because they are relatively easy to acquire and have the potential of causing casualties, contamination of widespread areas, adverse psychological effects on people, and economic disruption.
Simple Dispersal Device
A simple dispersal device (SDD) contains a high-energy source that irradiates people or spreads radioactive material in a populated area, such as an airport, train station, sports arena, or theater. Use of such a device to cause radiation exposure in unsuspecting people is considered a terrorist act. Common radioactive sources that could be used in an SDD are the same as the sources mentioned previously for RDDs. The threat of radiation exposure from an SDD would have the same psychological effect on people as an RDD: instilling fear.
Radiation Accidents
Information on radiation injuries has been gathered over a period of 70 to 100 years. Important information obtained from previous worldwide radiation accidents is useful in preparing and planning for nuclear terrorism and disasters. Radiation accidents can occur in situations where there are problems with nuclear reactors as well as industrial and medical sources of radiation. From 1946 to 2000, there have been more than 120 documented fatalities resulting from radiation accidents worldwide. Serious radiation accidents in the United States have been rare. From 1944 to June 2000, only 30 persons lost their lives in 13 separate radiation accidents in the United States. There were 233 other, less serious accidents during the same period. In the former Soviet Union 59 deaths occurred from radiation accidents between 1950 and 2000.
There are two categories of radiation accidents. The first category is an external exposure accident, that is, external irradiation from a source distant to or in close proximity to the body. Once a person has been removed from the source of radiation or the radiation-producing machine has been turned off, the irradiation stops. This exposed person does not become radioactive and there are no hazards to other people. The second category is a contamination accident. A person contaminated with radioactive material will continue to be irradiated until the radioactive material is removed, is eliminated, or decays. Contamination may occur in the form of radioactive gases, liquids, or particles. The contamination can be spread to other parts of the victim’s body as well as to others. There is a third category of accident: a combination of the two.
The effects seen in persons who have been exposed in radiation accidents can be categorized as either deterministic or stochastic radiation effects. Direct deterministic effects include the different types of acute and chronic radiation syndromes. The clinical manifestations of radiation injury include the latter as well as characteristic erythema, blistering, and even necrosis caused by local radiation damage. These deterministic effects occur when a specific dose-level threshold has been exceeded. The direct deterministic effects on an individual will depend on the dose of radiation received, the quality or type of radiation, the volume of tissue irradiated, and the time over which the dose is received. The stochastic effects are long term and include, for example, the induction of cancer and potential genetic abnormalities. More in-depth information on the medical management of radiation accident patients can be found in Medical Management of Radiation Accidents . In addition, improving health care following a catastrophic radiological or nuclear incident requires more educational training for health responders about ionizing radiation; radiation safety; and managing, diagnosing, and treating radiation-related injuries and illness.
Principles of Medical Management
The optimal medical treatment for injury following detonation of a nuclear weapon can be found in several valuable sources. , , , , Other articles have addressed medical treatment of individuals injured by a terrorist act involving radioactive materials. , , There is a growing body of evidence that suggests medical personnel in hospitals are unprepared for a large-scale radiological emergency, such as a terrorist event involving nuclear or radioactive materials. Clinicians and medical personnel lack sufficient training on radiological emergency preparedness and have concerns about overloading of clinical systems. The Centers for Disease Control and Prevention have provided a tool kit for use by hospital medical personnel who have to respond to a nuclear or radiological event. The medical response to radiation is the least taught topic in professional schools or civil preparedness organizations. Few medical professionals possess the basic knowledge or skills to identify, diagnose, and treat a radiation victim. Managing radiation injuries requires acute, critical, and long-term care during the course of illness.
Conventional injuries should be treated first, since radiation contamination is not a life-threatening medical emergency. Patients with traumatic blast and radiation injury should be resuscitated and stabilized. Airway, breathing, and circulation always take priority. These patients require more specialized treatment. Decontamination of patients should be carried out according to accepted standards of radiation decontamination. (See Chapter 84 for further discussion.) Specialists in hematology, oncology, radiation, and infectious disease should be consulted. Effective treatment of internally contaminated patients requires knowledge of both the radioactive isotope and its physical form. Treatment should be instituted quickly to ensure effectiveness. However, with a terrorist incidence, initially the radioactive source or sources are not known. Several general approaches may be used to treat internal radiation contamination, including reduction of absorption (Prussian blue), dilution (force fluids), blockage (potassium iodide), displacement by nonradioactive materials (oral phosphate), mobilization as a means of elimination from tissue (ammonium chloride), and chelation (calcium and zinc diethylenetriamine pentaacetate [Ca-DTPA and Zn-DTPA]). Although no drugs have been licensed in the United States to treat acute and long-term radiation injuries in the event of large-scale radiation or nuclear public health emergency, there is a goal to ensure the government stockpiles of medical countermeasures to treat radiation injuries.
Patients who have received a low whole-body radiation dose may develop gastrointestinal (GI) distress within the first 2 days. Antiemetics may be effective in reducing the GI symptoms. Symptoms will usually subside within the first day of treatment. If not, parenteral fluids should be considered. The prognosis for patients who have suffered traumatic blast, burn, and radiation injury is worse than for patients with radiation injury alone. A wound that is contaminated with radioactive materials should be rinsed with saline and treated using conventional aseptic techniques. For example, alpha-emitting radioactive isotopes that contaminate wounds are usually excised. In patients who receive whole-body doses of radiation greater than 100 cGy, the wound should be closed as soon as possible to prevent it from becoming an entry for lethal infection.
In spite of the wide availability of antibiotics, infections from opportunistic pathogens are a major problem among patients exposed to intermediate and high doses of radiation. In these cases the primary determinants of survival are treatment of microbial infections and aggressive resuscitation of the bone marrow.
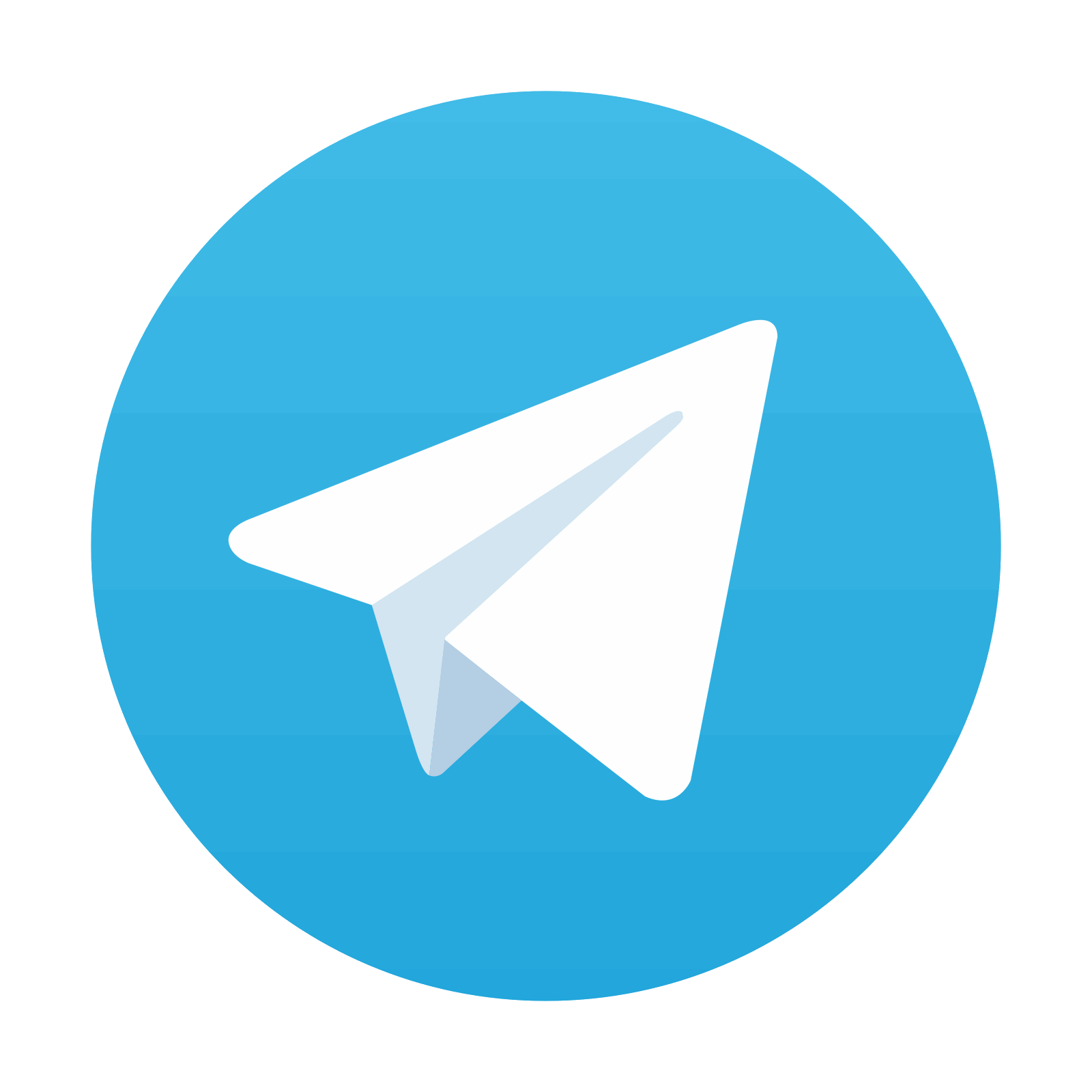
Stay updated, free articles. Join our Telegram channel

Full access? Get Clinical Tree
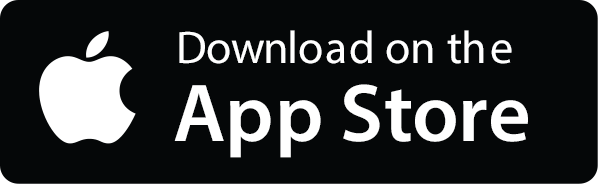
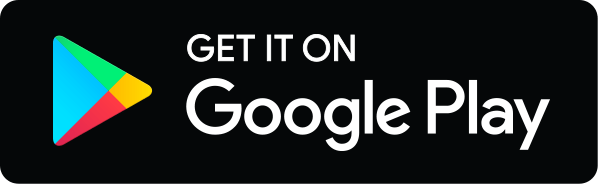