FIGURE 20.1 Schematic illustration of Simpson’s rule of discs for calculating left ventricular volume. (From Armstrong WF, Ryan T. Evolution of systolic function of the left ventricle. In: Feigenbaum’s Echocardiography. 7th ed. Philadelphia, PA: Lippincott Williams & Wilkins; 2010:127, with permission.)
Ventricular volumes obtained by 2D-ECHO have usually yielded smaller volumes than those obtained by cardiac MRI or nuclear medicine studies. This is attributed to the difficulty in excluding trabeculae and the improper visualization of the true endocardial border. In the absence of significant mitral regurgitation, SV can be obtained by subtracting the LVESV from the LVEDV.
SV = LVEDV – LVESV
Linear measurements of left ventricular function, such as the Fractional shortening method, can be quite misleading when there are regional wall motion abnormalities present and, as such, is not recommended. LVEF is a marker of left ventricular contractility and is affected by conditions that alter the preload, afterload, and contractility of the heart. Severe valvular heart disease can affect these parameters and needs to be taken into consideration when interpreting LVEF.
Right Ventricular Systolic Function
ECHO evaluation of the right ventricular function is complex due to its unique crescent shape. Multiple studies have demonstrated the clinical utility of TAPSE, S’ of the tricuspid annulus, and RIMP as surrogate measures of right ventricular ejection fraction (RVEF).
Tricuspid annular plane systolic excursion (TAPSE) is a one-dimensional measurement that represents longitudinal right ventricular function. It is measured by M-mode echocardiography with the cursor optimally aligned along the direction of the tricuspid lateral annulus obtained in the apical four-chamber view. TAPSE values less than 1.7 cm are highly suggestive of right ventricular systolic dysfunction (64). Tissue Doppler S’ of the lateral tricuspid annulus has also been shown to correlate with measures of right ventricular systolic function. An S’ velocity less than 9.5 cm/sec is indicative of RV systolic dysfunction (65).
CO and SV
CO is a key measurement in the management of the patient in shock. CO and SV can be obtained by a combination of Doppler and 2D US measurements. Pulse Doppler US allows us to measure intracardiac blood flow velocities; this is termed the velocity time integral or VTI. The VTI is the aggregate sum of blood flow velocities per heartbeat (SV) measured over time. By knowing the cross-sectional area through which blood flows through, we can then estimate blood volume (Figs. 20.2 and 20.3).
Stroke volume = cross-sectional area (CSA) × VTI Stroke volume = CSA (LVOT diameter squared × 0.785) × VTI LVOT
CO = stroke volume (SV) × heart rate (HR)
The preferred site for CSA measurement is the left ventricular outflow tract (LVOT) due to its minimal size variation during systole (66). CO measurements by the VTI method have been validated against invasive hemodynamic measurements with the PAC (67–70), and are reliable in the absence of valvular pathology.

FIGURE 20.2 A schematic demonstrates the concept of flow quantification using the Doppler technique. (From Armstrong WF, Ryan T. Hemodynamics. In: Feigenbaum’s Echocardiography. 7th ed. Philadelphia, PA: Lippincott Williams & Wilkins; 2010:219, with permission.)

FIGURE 20.3 The method for quantifying stroke volume. (From Armstrong WF, Ryan T. Hemodynamics. In: Feigenbaum’s Echocardiography. 7th ed. Philadelphia, PA: Lippincott Williams & Wilkins; 2010:219, with permission.)
Right Atrial Pressure/Central Venous Pressure
Right atrial pressure (RAP) or mean CVP can be estimated from the IVC diameter and its collapsibility on inspiration. Images are obtained of the intrahepatic portion of the inferior vena cava from the subcostal views. The combination of these two parameters (diameter and collapsibility) results in an accurate estimation of the mean RAP in patients who are not mechanically ventilated or who do not have elevated intra-abdominal pressures. In ventilated patients, an IVC diameter less than 1.2 cm appears to accurately identify patients with a RAP less than 10 mmHg. In this same group, if the IVC is small and collapsed it is suggestive of hypovolemia (64,65).
Another method to estimate RAP is through hepatic vein flow patterns obtained from the same subcostal views. Hepatic vein flow velocities have been validated in mechanically ventilated patients by a small number of patients (71) (Fig. 20.4 and Table 20.1).

FIGURE 20.4 Inferior vena cava (IVC) view. Measurement of the IVC. The diameter (solid line) is measured perpendicular to the long axis of the IVC at end expiration, just proximal to the junction of the hepatic veins that lie approximately 0.5 to 3.0 cm proximal to the ostium of the right atrium (RA). (From Rudski LG, Lai WW, Afilalo J, et al. Guidelines for the echocardiographic assessment of the right heart in adults: a report of the American Society of Echocardiography. J Am Soc Echocardiogr. 2010;23:685–713, with permission.)
TABLE 20.1 Relationship Between IVC Diameter and Collapsibility with Estimated Right Atrial Pressure | ||
![]() |
Systemic Vascular Resistance
If we know the SBP of a patient (by cuff pressure or arterial line), we can then calculate the MAP and obtain the estimated RAP and CO by cardiac US as already described above. We can then deduce systemic vascular resistance (SVR) by applying Ohm’s law.
SVR = MAP – CVP/CO
Left Atrial Pressure
In the critically ill patient, left atrial pressure (LAP) measurements are not easily derived, nor should they be inherently trusted. Many factors need to be assessed and integrated clinically at the bedside. The parameter that best correlates with elevated LAP is E/e′ ratio greater than 15 (<8 being normal). This ratio is obtained by pulse Doppler interrogation of the early mitral filling velocity (E) and then divided into the early diastolic mitral annular velocity (e′) as obtained by tissue Doppler velocities. The ratio has been shown to accurately predict elevated filling pressures in the heart failure population. Factors that can cause significant variability are regional wall motion abnormalities affecting the basal myocardium, preload conditions, depressed systolic function, mitral annular calcification, tachyarrhythmia with E/A fusion, and hypertrophic cardiomyopathy (72).
Pulmonary Artery Systolic Pressure and Mean Pulmonary Artery Pressure
Pulmonary artery systolic pressure (SPAP) can be quantified by measuring the right ventricular pressure through the tricuspid regurgitation peak jet velocity. Because the RV systolic pressure (RVSP) and SPAP are—in the absence of pulmonary stenosis—similar; this approach provides a simple means of quantifying pulmonary artery pressures (71).
RVSP = 4 × (TR velocity)2 + RAP
Mean pulmonary artery pressure (MPAP) can be obtained by pulsed Doppler interrogation of the RV outflow tract and obtaining the acceleration time (AT) of the pulmonic valve VTI. An AT less than 90 ms is suggestive of significant pulmonary hypertension (MPAP >25 mmHg). MPAP can also be deduced from the following formula:
MPAP = 70 – (0.45 × AT)
Stroke Volume Variation and Fluid Responsiveness
Over the last decade there has been an increase in the number of studies emphasizing the importance of SVV and fluid responsiveness in the critically ill (15,60,73). ECHO can easily measure SV by pulse Doppler interrogation of the LVOT, the preferred site for this. By obtaining the VTI and SV measurements (described in the CO section, above) a fluid challenge is given and SV is remeasured. An increase in SV greater than 10% suggests volume responsiveness in the critically ill.
As with any technology, cardiac US has its own limitations and drawbacks. The first barrier is that of the human body and its acoustic windows. Air, a poor conductor of US waves, can impair and degrade image quality in those patients who have underlying lung disease or are mechanically ventilated. Further, acoustic windows may not be accessible due to postoperative pain, positional changes of the heart, drainage catheters, or skin dressings.
Another important barrier, which must be noted, is the learning curve and skill required in both the acquisition and interpretation of US images. This is a learned skill that requires dedicated training and will also be dependent on the power of the equipment used. Training requirements and expertise needed for analysis and interpretation of ECHO have been well delineated in professional society guidelines (74,75).
Finally, one of the biggest drawbacks of ECHO is its noncontinuous nature. Echocardiography provides a “snapshot in time” thereby limiting its ability to serve as a continuous monitoring tool in the critically ill patient.
SUMMARY
The future for hemodynamic monitoring is promising given new technologic advances on the horizon. The ideal hemodynamic monitor will be noninvasive, provide continuous display of key hemodynamic variables, be readily applicable with no significant learning curve, and help guide therapeutic interventions for improved outcomes. At this time the perfect hemodynamic monitor does not exist, either invasive or noninvasive. Our current monitors operate on different physiologic principles and assumptions of which critical care practitioners need to be thoroughly familiarized with. Additionally, most devices do not provide a complete hemodynamic picture but rather a partial one. Failure to understand the physiologic principles on which devices function will lead to misinterpretation and/or misdiagnosis in complex cardiovascular conditions. As of today, echocardiography is the most complete noninvasive hemodynamic monitor at our disposal but is limited by its noncontinuous monitoring capabilities and significant learning curve.
Key Points
- Continuous and noninvasive cardiac monitoring modalities are potentially innovative tools for the bedside assessment of hemodynamic parameters.
- The ideal hemodynamic monitor will be noninvasive, provide continuous display of key hemodynamic variables, be readily applicable with no significant learning curve, and help guide therapeutic interventions for improved outcomes.
- Currently there is no ideal hemodynamic monitor that is able to provide all hemodynamic variables in a reliable and continuous fashion.
- For many modalities, data regarding measurement performance in comparison with reference methods for CO assessment are conflicting.
- Each modality has its specific advantages and limitations regarding usefulness in the clinical setting.
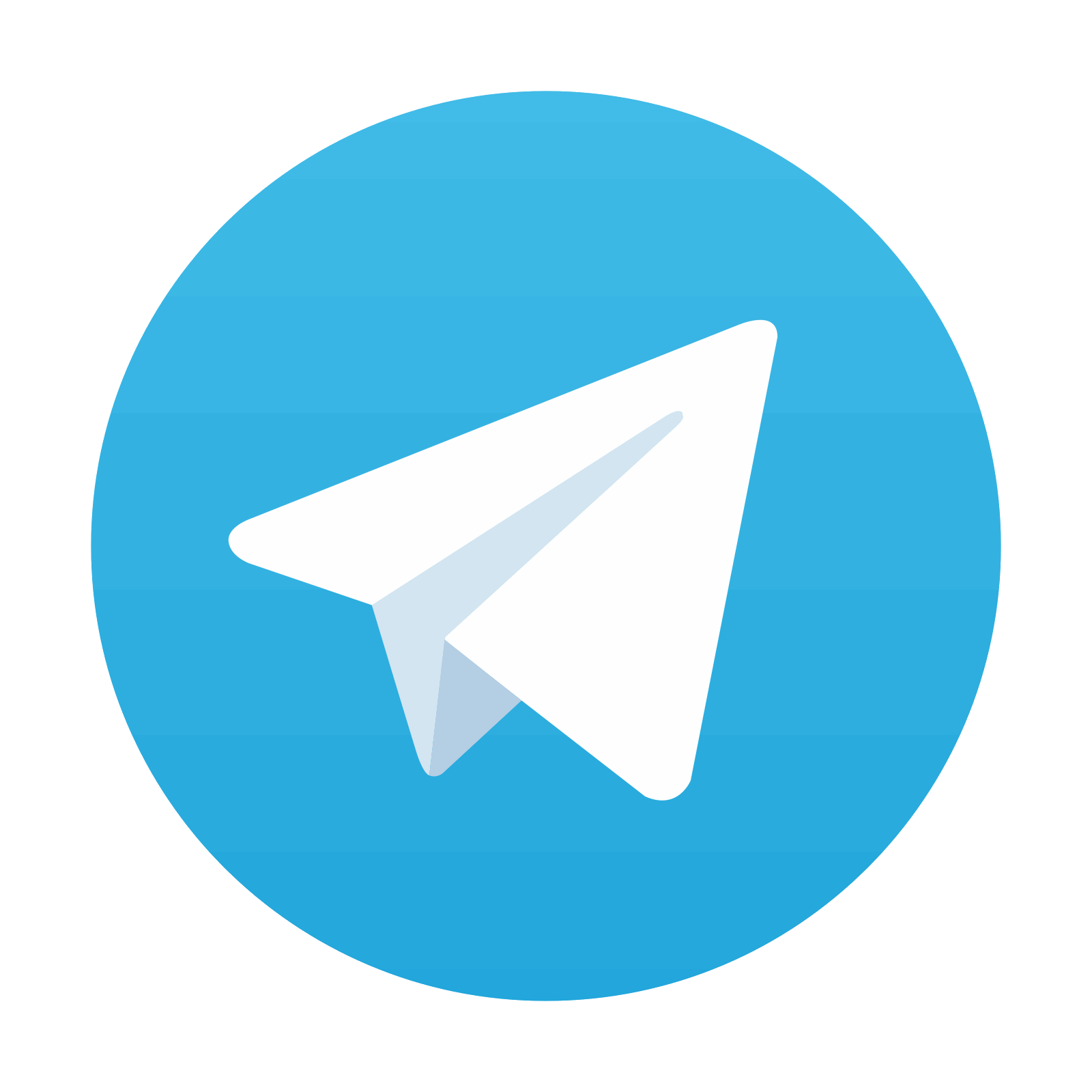
Stay updated, free articles. Join our Telegram channel

Full access? Get Clinical Tree
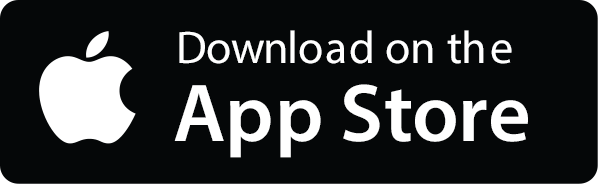
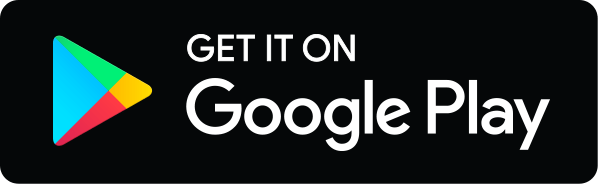