New Horizons
Allan I. Basbaum
This chapter occupies the same concluding position as the late Pat Wall’s essay on “new horizons” in the prior edition. Although it is now more than 40 years since Melzack and Wall’s publication of the gate control theory of pain (1), and much has been written about the impact of this theory on pain research and management, I fear that practitioners of regional anesthesia and pain management need to be reminded of its relevance—its increasing relevance—to the development of new treatments that will advance their practice in the near term. Certainly, the rationale for many current pain treatments is based on the view that they “close the gate.” Unfortunately, endless discussions with both clinicians and basic scientists have convinced me that the great majority of pain researchers and clinicians have an incomplete understanding of the underlying principles of gate control theory. They appreciate that gate control theory argues strongly against the Cartesian view of pain as having a single labeled line for transmission, with pain afferents, pathways, and cortical areas, but overlook or do not understand many critical elements of this theory.
In formulating the gate control theory of pain, Melzack and Wall recognized the variability of the clinical condition of pain. The word allodynia had yet to be coined, but they understood that pain was not merely a consequence of the individual being exposed to a noxious stimulus. Gate control theory, of course, postulated that the activity of small-diameter afferents that respond to noxious stimulation—nociceptors in today’s parlance—is not the sole determinant of the magnitude of pain experienced. Rather, pain is a consequence of the balance of activity in small- and large-diameter afferents and the central nervous system (CNS) circuits engaged by these afferents. Later elaborations of gate control theory incorporated the important contribution of the affective/emotional impact of the particular noxious stimulus, and the importance of the context in which the stimulus is experienced; that is, the critical contribution of experiential and cognitive factors (2). Together, these ideas underscore the view that pain is a complex perception, not simply the registering of a stimulus. Although it is highly likely that an intense noxious stimulus will be experienced as painful by most individuals, and indeed in the majority of medical and surgical settings in which the stimulus is generated, this type of acute pain is very different from chronic pain conditions that pain physicians generally face. We must explain why innocuous stimuli produce pain (allodynia) after tissue or nerve injury or why pain can persist after the injury resolves.
This marvelous book covers many aspects of the pathophysiologic basis of persistent pain and illustrates the many ways that blockade or inhibition of nerve conduction can influence pain processing. It seemed redundant, therefore, merely to summarize the field in the present concluding essay. I decided instead to look back at the gate control theory, to emphasize its major features—not all of which relate to “closing the gate”—and perhaps most importantly, to ask if it is still relevant today. I believe that it is, but not for the reasons that one might expect!
Gate Control Theory: A Primer
In Figure 51.1, I have broken down the major components of the spinal cord network that constitute the elements of gate control theory of pain. The classic Melzack and Wall paper appeared soon after Patrick Wall published his seminal electrophysiologic studies on the laminar organization of the spinal cord dorsal horn (3). At that time, the focus on neurons that are responsive to noxious stimulation was in the neck of the dorsal horn, specifically lamina V. Of particular interest was the wide dynamic range (WDR) neuron, which receives convergent inputs from small- and large-diameter primary afferents. Many of these neurons send projections to supraspinal regions (reticular formation and thalamus). Gate control theory referred to this neuron as the “T cell”, for transmission neuron. Today, it would likely be identified with the lamina V WDR neuron and almost certainly as well to the lamina I nociresponsive neurons (4). Interestingly, many of the latter receive no direct monosynaptic input from large-diameter myelinated (Aβ) afferents, which partly accounts for their nociceptive-specific properties. On the other hand, it is almost certain that, in the setting of injury, many of the lamina I neurons are transformed into WDR cells, so that we can safely include laminae I and V neurons in the output that is regulated by the gate of the original gate control theory. Note that gate control theory did not equate the output of the T cell with “pain.” Rather, Melzack and Wall depicted an arrow pointing to an “action system,” a more generic term that emphasizes the motivational dimension of stimuli and circuits that produce pain. “Action system” also connotes that noxious stimuli not only evoke pain, but also generate a behavioral repertoire through which the organism responds to noxious stimuli.
Gate control theory begins with the simple view that C-fibers (i.e., nociceptors) directly activate spinal cord projection neurons that transmit a nociceptive message to higher centers (Fig. 51.1A). It is of interest that this conclusion was based on electrophysiologic grounds and on mistaken anatomy. Thus, in 1965 it was argued, based on remarkably insensitive anatomic tracing techniques, that C-fibers do not project to the superficial dorsal horn (i.e., to laminae I or II), but only to the deep dorsal horn, where they could monosynaptically activate the WDR neurons in this region. This conclusion was consistent with electrophysiologic recordings that unequivocally established that increasing the current to activate C-fibers in a peripheral nerve excited WDR neurons over and above
that produced by electrical stimulation of large myelinated afferents. In fact, modern anatomic techniques have established not only that primary afferent C-fibers massively project to laminae I and II, but also that few if any project directly to lamina V. Of course, C-fibers can directly activate some deep dorsal horn WDR neurons because the dendrites of some of these neurons extend dorsally into the superficial dorsal horn.
that produced by electrical stimulation of large myelinated afferents. In fact, modern anatomic techniques have established not only that primary afferent C-fibers massively project to laminae I and II, but also that few if any project directly to lamina V. Of course, C-fibers can directly activate some deep dorsal horn WDR neurons because the dendrites of some of these neurons extend dorsally into the superficial dorsal horn.
If pain is purely a function of the activity of nociceptors, then the direct activation of WDR neurons by “pain-responsive” C-fibers is the modern equivalent of the Cartesian, specificity view of “pain” transmission. But Melzack and Wall emphasized that the output of the WDR neuron is under incredibly complex controls. Based on other studies from Wall’s laboratory, they incorporated the contribution of the circuits engaged by large-diameter primary afferents, which generally do not respond to noxious stimulation, and which, on that basis, would be excluded from the Cartesian view of pain processing. As illustrated in Figure 51.1B, large-diameter primary afferent fibers regulate the firing of the WDR neurons by two very different mechanisms. First, they excite the neurons, something that is often forgotten. Presumably this results from direct monosynaptic excitatory input to the WDR cell or via a chain of excitatory interneurons. Second, and perhaps more importantly, large-diameter, rapidly conducting afferents also inhibit the WDR cell, via a feed-forward inhibitory mechanism.
Some of Pat Wall’s most elegant electrophysiologic studies identified the site and mechanisms through which feed-forward inhibitory control is exerted (4). The then-prevailing view was that inhibitory controls, including the circuitry that underlies presynaptic inhibition of primary afferent fibers, involve interneurons in the deep dorsal horn. Pat Wall’s studies turned attention to what at the time were completely enigmatic interneurons of the substantia gelatinosa (SG) of the superficial dorsal horn (lamina II). Space does not permit a discussion of how the SG was implicated. But it is of interest to remind readers that this postulate was formulated well before extracellular or intracellular recordings had been made from SG neurons, even before it was known that C-fibers directly project to the SG, and well before the incredibly complex neurochemistry of the SG had been characterized (see later discussion). Pat Wall’s inferences were drawn from studies of cord dorsum potentials, which led to the view that large-diameter afferents excite some SG interneurons. These interneurons, in turn, via both pre- and direct postsynaptic inhibitory controls, were proposed to regulate/inhibit the output of the dorsal horn WDR neuron. This critical feature of gate control theory is illustrated in Figure 51.1C.
It did not take long for this simple circuit to be cited as the explanation for many common clinical findings and, for that matter, for the anecdotal experiences of almost everyone. Rub your hand when it hurts, and it gets better. Shake it, and it gets better. Rubbing and shaking, of course, activate large-diameter afferents, which should “close the gate.” Interestingly, if you burn the tip of your finger, you would not just rub the tip. This is reasonable, as it will hurt even more, presumably because you have not only activated sensitized C-fibers, whose threshold has now dropped, but almost certainly the large-diameter afferents innervating the injury site. These are the large-diameter afferents that directly excite the “pain” transmission “T cell” of gate control theory. However, when you rub the area that surrounds the zone of injury, it causes profound inhibition of the WDR neuron. This inhibition is almost certainly mediated by γ-aminobutyric acid (GABA)ergic and glycinergic interneurons in the SG.
Clinically, the consequence of engaging the circuits that are activated via large-diameter primary afferents is a reduction of ongoing pain. Not surprisingly, from the moment gate control theory was published, many physicians tried to develop new and better methods to “close the gate.” Vibration is effective for some types of pain, but transcutaneous nerve stimulation (TENS) is probably the most dramatic offshoot, albeit a somewhat more expensive approach to closing the gate (Figure 51.1C). Transcutaneous stimulation does work for some conditions. Why its effects are so limited is of interest in and of itself. The explanation may teach us much about the limits that the surround inhibitory circuitry in the dorsal horn can exert upon the output of “pain” transmission neurons.
Among the most significant clinical successes that emanated from the publication of gate control theory was the development of dorsal column stimulation for the treatment of pain. The idea was to activate populations of large-diameter afferents that not only collateralize into the spinal cord, but that also send major branches via the dorsal columns to the dorsal column nuclei of the medulla (Figure 51.1D). Describing the development of dorsal column stimulation for pain control, Pat Wall wrote, in a 1996 retrospective on gate control theory (5), “to activate more large fibers we next inserted the stimulating electrodes in the cauda equina. … William Sweet’s resident, Norman Shealey advanced the electrodes rostrally, and placed them directly on the dorsal columns….” The paper describing this procedure was published in Science (6) and provided a dramatic and remarkably rapid example of translating basic science observations to the clinic. It is not clear whether the mechanism of inhibition involves antidromic activation of large-diameter afferents and their collaterals that enter the dorsal horn, thus activating local inhibitory circuits, or involves a loop through the brainstem. Interestingly, the original gate control theory included a “central control trigger” through which spinocorticospinal loops were hypothesized to regulate the output of the gate. Although Melzack and Wall postulated that the ascending loop of the central control trigger coursed in the dorsal columns, the control that it was thought to subserve is in many respects comparable to the controls exerted by descending inhibitory circuits that arise in midbrain periaqueductal gray and medullary raphe (7,8), which are generally considered to be activated by “painful” inputs. It is of interest that recent studies of Porreca and colleagues (9) have provided significant evidence for a contribution of ascending axons in the dorsal columns to the activation of spinal-bulbospinal loops that influence the transmission of nociceptive signals by dorsal horn neurons.
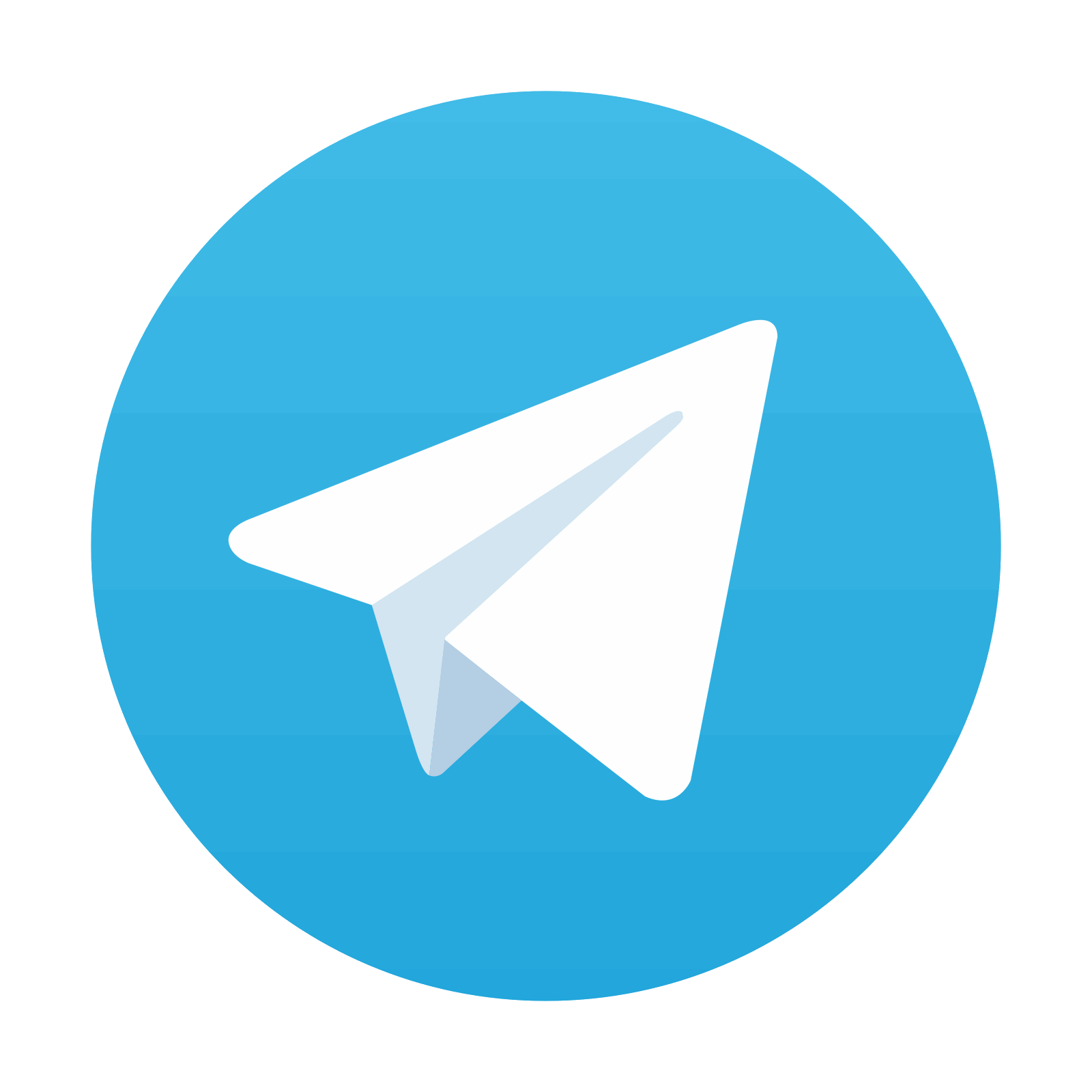
Stay updated, free articles. Join our Telegram channel

Full access? Get Clinical Tree
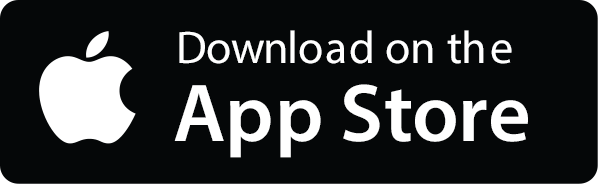
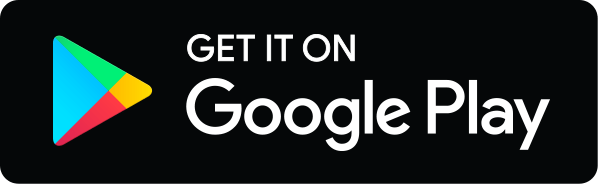