Neurostimulation
Joshua P. Prager
Michael Stanton-Hicks
For more than 30 years, interventional pain specialists have used spinal cord stimulation (SCS) to treat a variety of pain syndromes. Unlike neuroablation, SCS offers the opportunity to evaluate efficacy during a preimplantation screening trial, spares nerve tissue, and can later be reversed if necessary. In 1967, Shealy and colleagues reported on the first clinical application of SCS for pain management using electrodes placed surgically within the subarachnoid space (1). Building on the gate control theory of pain postulated only a few years earlier by Melzack and Wall (2), they sought to activate large-diameter nerve fibers selectively, thereby closing the gate for transmission of pain via small-diameter fibers in the dorsal horn of the spinal cord. Early enthusiasm for the procedure waned due to mixed clinical results (3,4). Two developments spurred renewed interest in SCS—consistent definitions of pain types by the International Association for the Study of Pain (IASP), which allowed the indications for SCS to be refined and standardized, and technologic advances in stimulation equipment, including multichannel implanted pulse generators (IPGs) and a variety of implantable or percutaneous electrodes placed through a needle without need for incision. The percutaneous technique permitted a prognostic trial of stimulation, to demonstrate potential efficacy before committing to an open surgical procedure. As the specific indications for SCS were defined and new stimulation systems became available, outcomes improved (5). Ample clinical evidence now supports the value of SCS in relieving chronic neuropathic pain resulting from failed back surgery syndrome (FBSS), complex regional pain syndrome (CRPS) I and II, and a variety of other pain conditions (6). Equipment also continues to evolve, with rechargeable miniaturized devices, robust programming capabilities, variation of lead design for specific applications, and greater patient control of devices being among the most recent improvements.
Dorsal Column Stimulation
Historical Overview
Electrotherapy boasts an ancient although not always distinguished history. In 46 A.D., the Roman physician Scribonius Largus, who first wrote about the medical application of electricity, mentions using the electrical discharge of torpedo fish to treat gout and headache (7). The invention of the Leyden jar in 1745 allowed manmade electricity to be applied to the treatment of pain (8). By the late 18th century, the English surgeon, John Birch, reported treating cases of low back pain and gout with electrostatic machines. The Galvani–Volta controversy, which led to the invention of the electrochemical battery in 1800, introduced “galvanism,” the application of galvanic current to the human body. This therapeutic “contact electricity” eliminated the painful sparks that often accompanied earlier static electrical treatments. In 1823, Chevalier Sarlandiére proposed delivering electric current through acupuncture needles, and he began using electroacupuncture to treat patients suffering from gout, arthritis, and sciatic and lumbo-sacral neuralgias (9). The Danish scientist Hans Christian Oersted launched modern electrotherapy in 1891, by demonstrating that a magnetic field surrounds an electric current. Numerous European scientists and physicians began studying electromagnetic induction and applying “localized electrization,” “catalytic action,” and “interrupted” current clinically. In his Treatise on Medical Electricity, Julius Althaus became the first to report using transcutaneous current applied to peripheral nerves as a method of pain relief (10,11).
In North America, electrotherapy was championed by the medical profession as well as exploited by quacks. In 1891, Drs. Beard and Rockwell (12) published a popular and influential treatise on electrotherapy that contained a chapter on neuralgia and low back pain. By the turn of the 19th century, the majority of American physicians were employing electrical machines in their practices, often without any scientific basis. Release of the Flexner Report in 1910 triggered major reforms in medical education and effectively ended the era of pseudoscientific therapies, including electrotherapy. In addition, conditions previously treated with electrotherapy increasingly seemed amenable to diagnosis with radiography and treatment with analgesics. Yet electrophysiologic experiments continued, most notably using induction coil techniques for cardiac pacing (13) The gate theory of pain, proposed by Melzack and Wall in 1965 (2), furnished a credible anatomic and physiologic explanation for the efficacy of analgesic electrotherapy that could be tested experimentally. Their theory—that nonpainful stimuli can inhibit pain—formed the original basis for contemporary neurostimulation. As the gate theory continues to be refined and expanded, and simultaneously as the mechanism of neurostimulation is better understood, there is now controversy as to how well the gate theory explains how SCS actually produces pain relief.
Shealy and his neurosurgeon colleagues (1), noting that Wall had demonstrated pain reduction by peripheral nerve stimulation (14), viewed dorsal column stimulation as a natural extension. They applied advances in cardiac pacemaker technology to the electrical inhibition of neuropathic pain due to cancer by directly delivering current through electrodes implanted subdurally using externally powered stimulators. First reports of their experimental results were so controversial that they were refused publication in the Journal of Neurosurgery (15). Although Shealy made remarkable gains and enjoyed early success, he eventually abandoned dorsal column stimulation because of technical problems, surgical complications,
the difficulty of selecting appropriate patients, and high failure rates among patients in the hands of inexperienced implanters. Subsequent progress in all of these areas has established neurostimulation as an effective therapy for carefully selected and screened patients in expert hands. Today the North American Neuromodulation Society and the International Neuromodulation Society act as interdisciplinary forums for continuing improvements.
the difficulty of selecting appropriate patients, and high failure rates among patients in the hands of inexperienced implanters. Subsequent progress in all of these areas has established neurostimulation as an effective therapy for carefully selected and screened patients in expert hands. Today the North American Neuromodulation Society and the International Neuromodulation Society act as interdisciplinary forums for continuing improvements.
Table 41-1 Definitions of Key Terms Associated with Spinal Cord Stimulation | ||||||||||||||||||
---|---|---|---|---|---|---|---|---|---|---|---|---|---|---|---|---|---|---|
|
Practitioners adopted the broader term “spinal cord stimulation” to describe the procedure more accurately, as the complex mechanisms of electrical stimulation became clearer. The clinical goals of SCS have always been to relieve pain and to reduce the use of medication, with patients potentially benefitting from better quality of life (QOL), renewed ability to perform activities of daily living (ADL), and return to work in some cases (6). Achieving these goals depends, in turn, on the technical goal of producing “stimulation paresthesia at a subjectively tolerable (comfortable) level, overlapping (covering) a patient’s topography of pain” (6). Yet, technical success does not necessarily guarantee clinical success. Thus, the development of SCS has relied heavily on clinical observations, continuing elucidation of the anatomy, physiology, and psychology of pain, and successive technical improvements. Two clinical observations stand out—SCS more effectively curbs neuropathic or sympathetically mediated pain than nociceptive pain, and stimulation appears to suppress chronic but not acute pain. Today, the most common indication for SCS in North America is chronic neuropathic pain (6,16). Europeans use SCS most often to treat ischemic pain associated with intractable angina pectoris (17) and peripheral vascular disease (18).
As SCS has become more standardized, so too have the definitions for key terms. This chapter uses the terminology and definitions developed by the North American Neuromodulation Society (Table 41-1) (19).
Mechanism of Action
The first application of SCS for chronic pain was based on the gate theory of pain (2), which postulated that a gate existed in the dorsal horn of the spinal cord that governed the central transmission of neural activity signaling pain. This gate was opened when small afferent fiber activity exceeded large afferent fiber activity in the peripheral nervous system; conversely, the gate was closed when large fiber activity predominated. To its early proponents, SCS represented a means of electively closing the gate, thereby reducing or eliminating painful inputs to the spinal cord and brain. Although the general mechanism of pain relief produced by SCS is still understood in these gating terms, the truth is considerably more complex. How can we explain, for example, that closing the gate works effectively to manage chronic, neuropathic pain but not acute, nociceptive pain? Researchers at the Karolinska Institute in Sweden (20) and the University of Oklahoma (21) have published in-depth reviews of current experimental and clinical studies of the mechanisms operating in SCS.
Understanding the mechanism of action that underlies SCS begins with a knowledge of the anatomy of the peripheral nervous system (PNS) and central nervous system (CNS). Figure 41-1 depicts the peripheral nerve fibers and their relationship to the spinal cord tract. Electrical impulses (action potentials) generated by sensory stimuli travel along afferent nerve fibers of the PNS to the spinal cord, which is part of the CNS. These sensory impulses ascend three orders of neurons
as they move from the PNS to the CNS. First-order neurons are the primary afferent fibers. Second-order neurons exist in the dorsal horn of the spinal cord, where excitatory and inhibitory signals are processed and modulated. Projection to higher brain structures involves third-order neurons. The brain receives, interprets, and responds to incoming nociceptive signals projected from the spinal cord to the thalamus, which processes input before relaying it to the cerebral cortex. The cerebral cortex identifies, modulates, and interprets sensations, and then oversees behavioral responses. Initially, the brain may suppress incoming impulses through antinociception or a descending analgesic pathway, thereby reducing pain perception. However, intense, prolonged noxious stimuli eventually cause tissue damage, which sensitizes peripheral nerves. In the presence of persistent and prolonged pain, signals in the spinal cord switch from inhibition to amplification, and damaged nerves undergo physiologic changes resulting from intracellular enzyme cascades, receptor modifications, and novel gene expression. These changes characterize the progression of pain as a symptom of acute injury to pain itself as a chronic disease (see Chapters 31 and 32).
as they move from the PNS to the CNS. First-order neurons are the primary afferent fibers. Second-order neurons exist in the dorsal horn of the spinal cord, where excitatory and inhibitory signals are processed and modulated. Projection to higher brain structures involves third-order neurons. The brain receives, interprets, and responds to incoming nociceptive signals projected from the spinal cord to the thalamus, which processes input before relaying it to the cerebral cortex. The cerebral cortex identifies, modulates, and interprets sensations, and then oversees behavioral responses. Initially, the brain may suppress incoming impulses through antinociception or a descending analgesic pathway, thereby reducing pain perception. However, intense, prolonged noxious stimuli eventually cause tissue damage, which sensitizes peripheral nerves. In the presence of persistent and prolonged pain, signals in the spinal cord switch from inhibition to amplification, and damaged nerves undergo physiologic changes resulting from intracellular enzyme cascades, receptor modifications, and novel gene expression. These changes characterize the progression of pain as a symptom of acute injury to pain itself as a chronic disease (see Chapters 31 and 32).
![]() Figure 41-1. The spinal cord tract and peripheral fibers. From Oakley JC, Prager JP. Spinal cord stimulation: Mechanisms of action. Spine 2002;27(22):2574–2583, with permission. |
Table 41-2 Relative Conductivity of Intraspinal Elements | ||||||||||||||||||||
---|---|---|---|---|---|---|---|---|---|---|---|---|---|---|---|---|---|---|---|---|
|
Electrical Effects
Electrical stimulation depends on the conductivity of the intraspinal elements in relation to the electrical lead position. A depolarized neuron that is electrically positive (+) produces an action potential, allowing painful signals to proceed. A hyperpolarized, negatively charged (–) neuron loses the ability to propagate an action potential or raises the threshold for propagation. Careful placement of external electrodes allows SCS to produce the same effects. The negatively charged cathode is the active electrode in SCS; the positively charged anode shields neuronal structures from the effects of stimulation. The relative position of electrodes and their distance from the spinal cord are major determinants of axonal activation and paresthesia distribution (22).
Cerebrospinal fluid (CSF) is the most conductive intraspinal element, so any electrical field that encompasses the CSF has the greatest potential to be conducted to nearby structures (Table 41-2). Of the intraspinal structures, the longitudinal white matter demonstrates the greatest conductivity, transverse white matter is much less conductive, and gray matter falls in between (Fig. 41-2). Epidural fat demonstrates high impedance; thus, a lead placed in the most posterior region of the epidural space, in a part of the spine with large epidural spaces such as in the thoracic region, demonstrates low conductivity. Thus, paresthesia thresholds are highest in the mid thoracic region and lowest in the cervical area, where the posterior epidural space is smallest. Dura mater, which also has low conductivity, presents no significant resistance because it is so thin. Vertebral bone is least conductive, insulating thoracic (the heart) and pelvic organs from the electrical field produced by SCS.
Large-diameter nerve fibers have relatively low thresholds for recruitment by external cathodes. In the spinal cord, particularly near the dorsal column, the primary afferent fibers are conveniently segregated from motor fibers, which may possess a similar activation threshold. These afferent neurons can be selectively activated and may close the gate through their respective collateral processes to the dorsal horn. The original term “dorsal column stimulation” came from the position of the stimulating electrodes over the dorsal columns of the spinal cord. Although topographically accurate, the term proved physiologically simplistic and “spinal cord stimulation” is now preferred (see also Chapter 32).
The clinical goal of SCS is to produce an electrical field that stimulates the relevant spinal cord structures without stimulating the nearby nerve root. The threshold current necessary
to stimulate a nerve fiber increases with the distance between the fiber and the cathode, and is inversely related to the fiber diameter (23). This information allows predictions regarding the recruitment order of fibers, whether in a peripheral nerve, spinal root, or central pathway. Short pulses favor stimulation of large nerve fibers; wide pulses favor stimulation of smaller fibers. In SCS, improved paresthesia coverage may be achieved with pulses of 0.5 to 1 ms.
to stimulate a nerve fiber increases with the distance between the fiber and the cathode, and is inversely related to the fiber diameter (23). This information allows predictions regarding the recruitment order of fibers, whether in a peripheral nerve, spinal root, or central pathway. Short pulses favor stimulation of large nerve fibers; wide pulses favor stimulation of smaller fibers. In SCS, improved paresthesia coverage may be achieved with pulses of 0.5 to 1 ms.
Barolat described the placement of the active electrode (cathode) in relation to the area in which paresthesia is produced (24). He and his colleagues mapped sensory responses to epidural stimulation, as demonstrated in Figure 41-3. The lead contact typically rests several levels above the intended area for concordant paresthesia. If the lead is close to the midline, the electrical field will reach the spinal cord before reaching the nerve root at the level of the lead. The low threshold of the dorsal root sensory fibers makes it imperative that lead position be sufficiently midline to avoid recruitment of the root. Stimulation of the low-threshold nerve root produces the sensation of paresthesia along the nerve root and, although it is neurostimulation, it is not SCS.
The goal of SCS is to create paresthesia that completely and consistently covers the painful areas, yet does not cause uncomfortable sensations in other areas (25). This paresthesia production is accomplished by stimulating Aβ fibers in the dorsal column (DC) and/or the dorsal roots (DR). Dorsal column stimulation typically causes paresthesia in several dermatomes at and below the level of the cathode. In contrast, DR stimulation activates fibers in a limited number of rootlets in close proximity to the cathode and causes paresthesia in only a few dermatomes. Because of these factors, DR stimulation may not produce sufficient pain relief. Another potential drawback to DR stimulation is that the roots also contain large proprioceptive fibers which, when stimulated, can cause uncomfortable sensations and motor responses (26). These side effects can occur at pulse amplitudes that are below the value needed for full paresthesia coverage.
Because the perception of pain is subjective, the appropriate usage level must be individually defined. The usage range is the interval between the perception threshold, the amplitude necessary to produce paresthesia, and the discomfort threshold, the point at which the patient can no longer tolerate the paresthesia. The maximum therapeutic amplitude rarely exceeds 170% of the perception threshold (23). During screening, a lead activated in the mid usage range can be physically moved to the position that produces the best pain relief coverage for the patient (22). This technique is described as active electrode screening, commonly referred to as “trolling.” More advanced systems now allow trolling to be performed electronically, minimizing the need to physically move the lead. At amplitudes used clinically for pain relief, SCS produces peripheral, antidromically activated compound action potentials over major peripheral nerves (see Chapter 32, Fig. 32-32). Peripheral nerve stimulation reduces primary afferent conduction velocity, the conduction safety factor, and neuroma discharge (14,27), and these effects are selective according to fiber size. Thus, stimulation peripherally likely blocks normal afferent activity, suggesting that SCS relieves pain partially through peripheral effects.
Clinical observations have also demonstrated that therapeutic response only occurs in a limited range of frequencies. For example, nerve fibers fail to conduct action potentials when stimulated at higher frequencies (≥300–500 pps) (23) due to a depolarization block or neurotransmitter depletion, an effect known as frequency dependent conduction. Others describe clinically useful higher-frequency stimulation (28), which may produce phase cancellation. When the pulse frequency becomes smaller than the refractory period, the largest fibers will continue to activate regularly whereas smaller fibers will not. This effect is most pronounced at junctures where larger fibers branch into smaller ones, such as where primary afferent neurons to the dorsal column branch to the dorsal horn (29). Similarly, frequencies lower than 25 to 30 Hz may exacerbate pain in patients undergoing SCS.
Evoked activity occurs in the dorsal and ventral spinal cord at the level of the dorsal horn (30). In patients who experience pain relief, inhibition of the RIII flexor reflex suggests a local dorsal horn contribution (31). Recordings from the human thalamus have also shown CNS effects during SCS, perhaps representing an evoked potential effect related to pain relief. In animals, SCS activates the anterior pretectal nucleus, which controls descending pain inhibitory action. The period of inhibitory influence noted in animals is consistent with the pain relief reported by humans after stimulation.
Neurochemical Effects
Neurochemically, SCS may act to restore normal γ-aminobutyric acid (GABA) levels in the dorsal horn and possibly to effect the release of the central neuromodulator adenosine (32), thus reducing neuropathic pain. In rats, SCS induces GABA release (33), which apparently attenuates the release of excitatory amino acids, such as glutamate and aspartate (34). Spinal cord stimulation clearly does not work through the endogenous opioid system. However, work with neurotransmitters suggests that the infusion of adenosine or
GABAB agonist baclofen may be helpful in potentiating the effects of stimulation (30,33,35).
GABAB agonist baclofen may be helpful in potentiating the effects of stimulation (30,33,35).
Sympathetic Nervous System Effects
The use of SCS in treating angina pectoris suggests that dorsal column stimulation can produce both anti-anginal and anti-ischemic activity in an organ. Canine studies have documented modulated intrinsic cardiac neuronal activity when the dorsal aspect of the thoracic cord is stimulated electrically (36). Both sympathetic afferent and efferent fibers likely contribute to suppression of intrinsic cardiac activity during SCS. Anti-anginal effects decrease noxious inputs to pain pathways in the spinal cord, and the anti-ischemic effects are mediated by release of vasoactive substances. Linderoth and colleagues (37) and Croom and colleagues (38) demonstrated that stimulation increased blood flow at two-thirds and 90% of motor stimulation threshold, respectively. The vasodilative effect requires intact
dorsal roots. Release of calcitonin gene-related peptide (CGRP) at the synaptic terminal sites also causes vasodilation, mediated through antidromic activation of interneurons (38). At high stimulation thresholds (80%), antidromic effects are seen; at low stimulation thresholds (63%), orthodromic effects are seen. The anti-ischemic and analgesic benefits of SCS noted in patients with angina may also apply to those with peripheral ischemia, and possibly to those with CRPS.
dorsal roots. Release of calcitonin gene-related peptide (CGRP) at the synaptic terminal sites also causes vasodilation, mediated through antidromic activation of interneurons (38). At high stimulation thresholds (80%), antidromic effects are seen; at low stimulation thresholds (63%), orthodromic effects are seen. The anti-ischemic and analgesic benefits of SCS noted in patients with angina may also apply to those with peripheral ischemia, and possibly to those with CRPS.
Neurophysiologic Effects
Spinal cord stimulation appears to mainly affect pain related to Aβ fiber functions but not C fibers (31). Sciatic nerve injury, for example, increases the spontaneous firing of wide-dynamic-range (WDR) neurons responsive to sensory input from mechanical, chemical, and thermal receptors in the deeper lamina of the rat dorsal horn. Spinal cord stimulation suppresses these effects (24,39). Dorsal column lesions substantially lessen the effect of SCS, suggesting a dorsal column contribution to the SCS effect. Transecting the spinal cord above the level of SCS further reduces the effect of stimulation, indicating both supraspinal and segmental mechanisms. In animals, SCS modifies brainstem activity in the anterior pretectal nucleus, which is associated with descending pain inhibitory pathways (40).
Computer Modeling
A finite-element computer model has been used to study the distribution of electrical fields in spinal tissues of varying densities, to design electrodes, and to select targets for stimulation (41). The model predicts that the DR and DC fiber thresholds for stimulation are dependent on the dorsal CSF space, the position of the stimulating cathode with respect to the physiologic midline of the spinal cord, and the arrangement of negative and positive electrodes within a stimulating array (26). Modeling predicts the clinically confirmed observation that stimulation of the dorsal column is most efficient with closely spaced longitudinal bipolar (+/-) or tripolar (+/-/+) configurations centered on the spinal cord, where the main delivered current corresponds to the orientation of the nerve fibers (42). The model also predicts that narrower electrode spacing produces a more centralized stimulation with improved stimulation selectivity, although at the expense of power consumption (43). The model suggests that a transverse tripolar arrangement across the spinal cord can be used to steer the paresthesias electrically into an area of pain, rather than moving an electrode mechanically until pain control is achieved (44).
Better DC versus DR recruitment, higher usage ranges, and preferential avoidance of dorsal roots have been modeled successfully with a 4-8-4 array and transverse tripoles (45,46).
Most predictions of the model have been confirmed clinically. Figure 41-4 illustrates transverse tripolar SCS in a patient with failed back syndrome (47). Spinal cord stimulation has been traditionally recognized as an effective therapy in the treatment of radicular pain; its use to achieve pain relief in the axial low back has been more difficult (24,48). A small, theoretical region found between the T8 and T9 vertebral level junction (i.e., corresponding to the L1 and L2 spinal nerves innervating the low back) has been identified as an optimal SCS target for low back pain (49,50).
Indications for Spinal Cord Stimulation
The clinical results of SCS demonstrate that it controls the neuropathic component of pain more effectively than the nociceptive component, and treats pain associated with the sympathetic nervous system more effectively than that caused by the somatic nervous system. Neuropathic pain results from injury to the nervous system and commonly coexists with nociceptive or ischemic pain (see also Chapter 46). Anxiety and depression can also add to the perception of pain, complicating the clinical presentation. Results of SCS reported in the clinical literature support its use, in order of effectiveness, for the following indications: intractable angina (90% effective) (17), ischemic pain due to peripheral vascular disease (18), CRPS types I and II (formerly called reflex sympathetic dystrophy or causalgia) (16), and persistent neuropathic pain after spinal surgery (50% effective) (51). Recently North, Shipley, and members of The Neuromodulation Therapy Access Coalition (NTAC) published Practice Parameters for the Use of Spinal Cord Stimulation in the Treatment of Chronic Neuropathic Pain, a comprehensive, evidence-based literature review and consensus statement regarding SCS (6). Their detailed analyses represent the most extensive examination to date of
the likelihood of success with SCS for specific indications and of the associated risks and benefits.
the likelihood of success with SCS for specific indications and of the associated risks and benefits.
Table 41-3 Indications for Spinal Cord Stimulation Supported by an Evidence-based Literature Review and Consensus Statement | ||||||||||
---|---|---|---|---|---|---|---|---|---|---|
|
Failed Back Surgery Syndrome
Failed back surgery syndrome euphemistically describes a varied group of conditions suffered by patients who continue to experience debilitating nociceptive-neuropathic pain after lumbar spine surgery. Cases of FBSS described in the literature include lumbosacral pain, buttock pain, and sometimes diffuse lower extremity pain, which are attributed to incorrect diagnosis, wrong level of surgery, psychological illness, arachnoiditis, lumbosacral epidural fibrosis, radiculitis, vertebral instability, and recurrent disk herniations (52).
The lifetime prevalence of lumbar surgery in the United States is higher than in any other industrialized country, ranging from one to three surgeries per 100 Americans (53,54). Of the 200,000 Americans who undergo lumbosacral surgery to relieve chronic low back pain annually, 20% to 40% will experience continuing pain (55). As the population ages, the number of surgeries for degenerative lumbar conditions is expected to increase, as is the risk of FBSS. Unfortunately, many patients with FBSS who were referred to one multidisciplinary pain treatment center did not meet the criteria customarily accepted by neurosurgeons for surgical intervention at the time of their first surgery (56). This fact, coupled with persistent postoperative pain and sometimes by maladaptive behavior, complicates their subsequent medical management. However, some patients with FBSS are candidates for SCS, provided they meet patient selection criteria and successfully complete a screening trial.
In developing their practice parameters for the use of SCS, NTAC analyzed one randomized controlled trial (RCT) (57), 17 long-term follow-up studies, 12 short-term follow-up studies, four case or miscellaneous studies, and 31 studies of mixed indications for FBSS (6). The group also examined 16 studies of low back/leg pain or mixed indications that were not necessarily FBSS. From these sources, they concluded that there was excellent evidence (A = recommended or required; valid, useful, or non-negotiable) to support the use of SCS in treating FBSS (Table 41-3). Results of another RCT have since been published (58). These two RCTs offer compelling evidence of the efficacy of SCS in treating FBSS while also clarifying the necessary conditions for success. Both were well-controlled, randomized, crossover studies, one comparing SCS with repeat spine surgery and the other with conventional medical management (CMM). Compared with repeat spine surgery, SCS was significantly more successful (p <0.01), patients initially randomized to SCS were significantly less likely to cross over than those initially randomized to surgery (p = 0.02), and patients randomized to reoperation required increased doses of analgesics significantly more often than those randomized to SCS (p <0.025) (57). Compared with CMM, significantly more SCS patients had greater than 50% pain relief in their legs (p <0.001) 6 months after the start of the study (58). The SCS group experienced significantly improved (p <0.05) leg and back pain relief, QOL, functional capacity, and treatment satisfaction. Between 6 and 12 months, five SCS patients crossed to CMM and 32 CMM patients crossed to SCS.
The remaining body of evidence helps clarify the conditions necessary for successful treatment of FBSS with SCS. Numerous investigators have emphasized the importance of proper patient selection, a subject examined later in this chapter. It
should be noted, however, that specific pain syndromes, such as angina, peripheral vascular disease, and CRPS are beginning to include SCS as a treatment option, rather than as a last resort, for patients meeting well-defined diagnostic criteria (59). In FBSS, SCS has demonstrated efficacy for managing intractable leg pain, and to a lesser extent, low back pain (52). Yet most experienced implanters have found that SCS is far more effective in relieving radicular pain than in treating axial low back pain. Thus, FBSS patients with predominant low back pain, or mixed nociceptive-neuropathic pain may be difficult to treat with SCS. Some success in treating low back pain has been achieved using paddle electrodes and high-voltage radio-frequency coupled systems, especially those with sophisticated programming options (52). However, extensive reprogramming and long-term follow-up are probably necessary to maintain effective paresthesia coverage.
should be noted, however, that specific pain syndromes, such as angina, peripheral vascular disease, and CRPS are beginning to include SCS as a treatment option, rather than as a last resort, for patients meeting well-defined diagnostic criteria (59). In FBSS, SCS has demonstrated efficacy for managing intractable leg pain, and to a lesser extent, low back pain (52). Yet most experienced implanters have found that SCS is far more effective in relieving radicular pain than in treating axial low back pain. Thus, FBSS patients with predominant low back pain, or mixed nociceptive-neuropathic pain may be difficult to treat with SCS. Some success in treating low back pain has been achieved using paddle electrodes and high-voltage radio-frequency coupled systems, especially those with sophisticated programming options (52). However, extensive reprogramming and long-term follow-up are probably necessary to maintain effective paresthesia coverage.
Patients with FBSS who are considering SCS should have had chronic, intractable back and leg(s) pain for more than 6 months, the radicular component should be more severe than the axial component, and there should be no indication for additional spine surgery (such as progressive deformity, a lesion, or progressive motor deficit) (59). Contraindications to SCS for FBSS include an inadequate course of optimum nonsurgical care and previous failure of an SCS screening trial performed properly using current technology.
Several studies have considered the cost utility of SCS in treating FBSS. Bell and colleagues (60) compared the costs of SCS with surgeries and other treatments and reported that, in patients who responded favorably to SCS, the therapy paid for itself within 2.1 years. Devulder and colleagues (61) calculated an average annual cost of $3,660 per patient for SCS, which may be cost-effective if other therapies for FBSS have failed. In 2007, North and co-workers (62) reported on the difference in cost (cost effectiveness) and cost utility (quality adjusted life years) of SCS versus reoperation using data collected in a crossover RCT. The mean per-patient costs were US$48,357 for SCS versus $38,160 for reoperation (intention to treat), $48,357 for SCS versus $105,928 for reoperation (treated as intended), and $34,371 for SCS versus $36,341 for reoperation (final treatment). Thus, SCS was less expensive and more effective than reoperation in selected FBSS patients.
Complex Regional Pain Syndrome
In 1864, Mitchell first described causalgia (later called “reflex sympathetic dystrophy”), but the condition’s many variations and puzzling pathophysiology created a formidable diagnostic challenge. In 1994, the International Association for the Study of Pain (IASP) replaced the name “reflex sympathetic dystrophy” with “complex regional pain syndrome” (63). The change acknowledged the unclear role of the sympathetic nervous system in the disorder, and the fact that dystrophy does not occur in all patients. Instead, two forms of CRPS were defined: type I, in which all the features of reflex sympathetic dystrophy are present but with no definable nerve injury; and type II (formerly called causalgia), in which the nerve injury is definable (64). See Chapter 46, by Binder and Baron, for a much more detailed review of CRPS.
Traditionally CRPS has been treated with physical therapy in an attempt to prevent contractures, minimize atrophy, and facilitate return to function. Several pain-relieving therapies have been offered to enhance the patient’s ability to participate in physical therapy. Sympathetic nerve blocks can be used if they provide a sufficient duration of analgesia, or if the period of postprocedure analgesia increases with each block. Numerous systemic medications have been administered to provide “balanced” analgesia during physical therapy, including serotonin/norepinephrine reuptake blockers, nonsteroidal anti-inflammatory drugs, steroids, opioids, α-adrenergic blocking agents, membrane stabilizers, N-methyl-D-aspartate (NMDA) antagonists (28) and gabenoids, gabapentin, and pregabalin. Continuous epidural infusion of anesthetics, clonidine, or opioids can also provide analgesia during rehabilitation, although the technique is labor intensive, expensive, and prone to complications such as infection or catheter occlusion. Neuroablation of the sympathetic chain provides longer-lasting analgesia but is fraught with complications, irreversible, and not uniformly effective (65). In light of the emerging understanding of peripheral–cord–brainstem interactions, neurodestructive techniques should be considered a last resort (28), if at all (66).
The NTAC found excellent evidence supporting the use of SCS to treat CRPS (Table 41-3) (6). They identified three RCTs, six long-term follow-up studies, six short-term follow-up studies, 10 case studies, and numerous studies of CRPS in mixed indications. Kemler and colleagues reported results of the first RCT in 2000 (67). Enrolled patients met the diagnostic criteria for CRPS type I established by the IASP, and all of the patients had experienced severe pain that was unresponsive to conventional treatment for at least 6 months. Patients were randomly assigned to receive SCS plus physical therapy (n = 36) or physical therapy alone (n = 18). The stimulator was implanted only if trial stimulation was successful. Intention-to-treat analysis demonstrated a significant reduction in pain for patients in the SCS group compared with patients in the physical therapy group (p <0.001). Thirty-nine percent of patients in the SCS group had a much improved global perceived effect score compared with 6% in the other group (p = 0.01). None of the patients had clinical improvement in functional status. The QOL improved by 11% overall, but only in the 24 patients who actually underwent stimulator implantation. Six of these patients required additional procedures due to complications, including removal of one device.
The same researchers reported on their original patients after 2-year follow-up (68). The SCS plus physical therapy group still had significantly improved pain relief and global perceived effect compared with the physical therapy-only group (p ≤ 0.001). There was no clinically important improvement in functional status for either group. The investigators concluded that SCS provided long-term pain reduction and improved the health-related QOL in these patients treated for CRPS. At 5-year follow-up, the effects of SCS or physical therapy only were equivalent with regard to all measured variables (69). Global perceived effect for SCS patients (n = 20) was still significantly better (p = 0.02) than for physical therapy patients (n = 13), but there was no difference in pain relief (p = 0.06). Despite the diminishing effect of SCS over time, the overwhelming majority (95%) of SCS-treated patients said they would repeat the treatment for the same result. The statistical artifact that SCS provides short-term relief without demonstrating long-term pain relief is produced by the study design of intent-to-treat analysis, with patients having the ability to cross over to SCS if more conservative therapy failed (70).
The third RCT compared the analgesic effects of carbamazepine (600 mg/day) or sustained-release morphine (90 mg/day) in patients with CRPS who were pretreated with SCS (71). Forty-three patients had SCS switched off before receiving the pain medications or placebo. They could reactivate SCS if the pain became intolerable. Compared with placebo, carbamazepine significantly delayed a pain increase, but morphine did not, perhaps because the dose was too low. Two patients
taking carbamazepine and one taking morphine preferred to continue the medication. Thirty-five returned to SCS.
taking carbamazepine and one taking morphine preferred to continue the medication. Thirty-five returned to SCS.
Most of the published studies describing treatment of CRPS with SCS have been retrospective. In reviewing 10 of these, Bennett and Cameron (28) found an overall success rate of 82% (148/180) for patients with CRPS I and 79% (23/29) for patients with CRPS II. These results are encouraging, considering that many physicians reserve SCS until all other conservative therapies have failed. These results were also obtained with relatively limited stimulation systems having few electrode contacts and limited output capabilities. More sophisticated stimulation systems, although not yet tested in RCTs, have demonstrated statistically significant improvements in pain scores and overall patient satisfaction compared with baseline (72). For example, Bennett and co-workers (72) found greater improvement in patients with dual octapolar leads versus a single quadripolar lead, probably because octapolar leads can safely deliver higher frequencies and be carefully programmed to maximize paresthesia coverage. They believe that the more flexibility afforded by a stimulation system—through numbers or arrays of electrodes; range of pulse width, frequency, and amplitude; dual-channel capabilities; and programming options—the more attractive the system is for treating CRPS.
Current thinking regarding CRPS suggests that early intervention provides a higher probability of complete reversal of symptoms or a greater degree of symptom resolution (73). Given the demonstrated efficacy of SCS in treating longer-standing cases, Prager and Chang reported on the temporary use of SCS to provide adjuvant analgesia while patients underwent interdisciplinary treatment that commenced within 8 weeks of their injury (47). Eight patients had an external pulse generator system connected to an implanted lead to facilitate physical therapy. The lead was to remain for 4 weeks, with permanent pulse generator implantation performed if stimulation was required for a longer time. After that time, if the patient desired explantation, the lead was removed. Five of the eight patients (62.5%) had sufficient symptom resolution to remove the lead. Temporary SCS is relatively inexpensive compared to multiple serial sympathetic blocks or maintenance of an epidural infusion. In addition, an implanted SCS lead without an internal pulse generator can be converted to a permanent, totally implanted system, if necessary, after functional rehabilitation is completed.
A retrospective analysis was performed of 16 consecutive patients who had permanent SCS systems implanted to treat CRPS (74); two (12.5%) had their systems explanted (at 5 and 18 months) and were relatively symptom free 2 years later. Ample evidence in the current literature supports the efficacy of SCS in treating pain due to CRPS I and II compared to other modalities, such as physical therapy and medication. Its greatest utility, however, may be in combination with other therapies early in the course of CRPS, when therapeutic interventions may prevent or moderate disability. In a preliminary report of patients receiving treatment for various types of neuropathic pain, SCS or intrathecal drug therapy was used in combination with cognitive behavioral therapy (CBT), given before or after CBT. Improvements in mental and physical function occurred only when SCS was combined with CBT (76a).
Spinal Cord Stimulation and CRPS in Children
Complex regional pain syndrome type I is not uncommon in children. Early treatment is often effective provided it comprises CBT, including reprogramming of motor function, sometimes supported by a short course of “neuropathic drugs.” However, some children are referred very late or the condition escalates rapidly, so that all conservative treatments fail. In this situation, a short course of epidural clonidine may be helpful but requires hospitalization. A better option is the placement of a percutaneous SCS lead, which may be sufficient, over a week or so, to allow CBT to proceed. Severely affected children may be markedly improved by implantation of an SCS system (76b). This should only be carried out by highly experienced SCS implanters.
Peripheral Vascular Disease
Critical limb ischemia (CLI) is the most common vascular indication for SCS (75). In nondiabetic patients, CLI is defined as “the presence of rest pain or tissue necrosis (ulceration or gangrene) with an ankle systolic pressure of 50 mm Hg or less, or a toe pressure of 30 mm Hg or less (76). Vascular surgeons have proposed adding a microcirculatory measure to the definition, perhaps a combination of toe blood pressure (<30–50 mm Hg) and transcutaneous oxygen tension (TcpO2, <30–50 mm Hg) (77).
Intermittent pain (vascular claudication) is the earliest symptom of ischemia and probably includes both nociceptive and neuropathic elements. The analgesic effect of SCS on CLI may be due to modulation of neuropathic pain, antinociception, and primary or secondary anti-ischemic effects (78). Decades ago, Cook noticed that SCS generated autonomic changes and pain relief in patients with spinal cord lesions or multiple sclerosis (79). About the same time, Dooley observed increased peripheral blood flow in patients treated with SCS for CNS disorders (80). More recently, Ghajar and Miles (81) found that SCS increased blood flow and skin temperature in the legs if the stimulating electrode was positioned below the T10 vertebral level, preferably at T12. Exactly how SCS influences blood flow remains a matter for further study, although sustained vasodilation has been demonstrated in humans and animals during antidromic stimulation of dorsal root afferents (82). Tallis and co-workers proposed that conventional pain relief might reverse the sympathetic vasoconstriction that accompanies pain, or that SCS induces an electrical sympathetic paralysis (82).
Beginning in Europe during the 1990s, epidural SCS was used to treat peripheral vascular disease in patients who could not undergo vascular reconstruction. In these diverse patients with severe limb ischemia, SCS relieved pain, permitted greater mobility, and promoted ulcer healing due to enhanced blood flow (75). Clinicians were encouraged by these results, because the alternative was generally amputation. The Second European Consensus Document on Critical Limb Ischaemia (76) added microcirculatory criteria to the Fontaine staging system previously used to grade peripheral arterial disease. The randomized studies that have since been conducted demonstrated no statistically significant advantage for SCS in limb preservation over medical management, but have documented pain relief, greater mobility, and improved QOL for patients treated with SCS (83,84). In a study of 120 patients randomly assigned to SCS or standard treatment, limb survival was 60% in the SCS group and 46% in the standard treatment group after 2 years (84). Further refinement of the patient selection criteria, especially with regard to microcirculatory measurements, may more accurately identify CLI patients who would respond optimally to SCS.
Intractable Angina Pectoris
Angina pectoris, a reversible myocardial ischemia, represents an imbalance between oxygen demand and supply that is caused by blood vessel obstruction or vasospasm. Angina can be triggered by exercise, blood redistribution to digestion after eating, cold, or mental stress. Patients with ischemic heart disease now enjoy increased QOL and longer life expectancy due to surgical and pharmacologic therapies. In a subset of patients, however, angina pectoris proves refractory to all treatments (85,86). The European Study Group on the Treatment of Refractory Angina Pectoris offers this definition for refractory angina pectoris: “A chronic condition characterized by the presence of angina, caused by coronary insufficiency in the presence of coronary artery disease, which cannot be adequately controlled by a combination of medical therapy, angioplasty, and coronary artery bypass surgery. The presence of reversible myocardial ischemia should be clinically established to be the cause of symptoms” (87).
Approximately 100,000 patients in Europe and the United States meet this definition, most of them male, relatively young (in their early sixties), and previously treated with multiple revascularization procedures (88,89). Of all the available adjunct therapies, from medications such as amiodarone to experimental angiogenesis, SCS is considered one of the most successful and safest (90,91). For this reason, the European Study Group on the Treatment of Refractory Angina Pectoris recommends SCS as a treatment of choice (along with transcutaneous electrical nerve stimulation or TENS) for refractory angina (87). Relief of angina is most pronounced when SCS is applied in the epidural space at levels T1 to T2 (17).
Randomized and observational studies of SCS for refractory angina have demonstrated fewer anginal episodes, less use of short-acting nitrates, improved exercise capacity, and more favorable perceived QOL (92). The benefits of SCS persist for at least a year in 80% of patients, and almost 60% experience improved exercise capacity and QOL for up to 5 years (89,93,94). Separate from its pain-relieving effects, SCS therapy reduces myocardial ischemia as gauged by exercise stress testing and ambulatory ECG monitoring (95,96). Fears that SCS might obliterate the warning signs of angina, thus putting patients at risk of adverse myocardial events, are not borne out by the evidence. Spinal cord stimulation elevates the pain threshold but does not completely eliminate anginal pain, and pain perception reportedly remains intact during myocardial infarction (97,98). Nor is mortality adversely affected, according to prospective and retrospective studies of SCS for refractory angina pectoris (92). For example, in a retrospective follow-up study of 517 patients who had refractory angina and were treated with SCS, there was no negative effect on mortality (89). In a prospective study that compared SCS with bypass surgery, there was no difference between SCS and surgery regarding symptom relief at 6 months (95). The SCS group had lower mortality and cerebrovascular morbidity than the bypass group. Two-year follow-up showed that SCS was more cost effective than bypass (99) and 5-year follow-up that there was no difference in mortality (94).
Other Pain Conditions
When considering the scientific and clinical evidence for using SCS, members of the NTAC (6) analyzed well-controlled clinical studies and case reports describing other pain conditions, such as phantom limb/postamputation syndrome, postherpetic neuralgia (PHN), root injury pain, and spinal cord injury/lesion (Table 41-3). Weighing risk versus benefit and expert consensus, they concluded that SCS offers a good likelihood of a favorable outcome in treating these conditions (B = recommended; uncertain validity, apparently useful). In the absence of RCTs comparing SCS with other treatments for these pain syndromes, some individuals and groups have expressed skepticism or counseled extreme caution (100). A number of retrospective studies, however, do demonstrate long-term (5 to 20 years) successful outcomes for patients with these conditions (51,101,102,103,104,105). Many of the studies included mixed indications, with chronic, refractory pain the common denominator. This fact underscores the importance of patient selection (105,106) and the necessity of demonstrating SCS efficacy during a preimplantation trial (101,106). These factors are discussed in more detail later in this chapter.
Phantom Limb/Postamputation Syndrome
The IASP defines phantom limb pain as “pain referred to a surgically or traumatically removed limb or portion thereof” (107). Severe phantom pain affects 0.5% to 5% of amputees (108) for up to 71% of the time (109). Stump pain may arise from skin changes, circulatory problems, and infection or bone abnormalities, and may not always be neuropathic (108). Most reports of treating phantom limb pain with SCS are embedded in retrospective reviews of all types of chronic pain. Krainick and colleagues (110) followed 84 patients treated with dorsal column stimulation between 1972 and 1974, including 64 amputees. Good results were obtained in 52.4% after 2 years and 39% after 5 years. Tasker and Dostrovsky (111) reported 56% of 16 patients treated with SCS responded favorably. In a series of 15 patients, Oakley found that the five with neuropathic stump pain experienced improvement of more than 50% (112). In the same series, four of the 10 patients with painful phantom said they no longer experienced phantom discomfort, although phantom awareness persisted. Pain relief was related to subjective reports of paresthesia in the phantom. These results demonstrate that SCS can provide pain relief in some cases of phantom limb/postamputation syndrome, while also pointing to the need for further study of this application.
Postherpetic Neuralgia
Two studies offer evidence of efficacy for SCS in treating PHN. The first examined outcomes for 109 patients who underwent SCS between 1978 and 1986, 10 of whom had PHN (104). Six of the 10 experienced 52.5% mean analgesia and reported long-term implant success. In a more recent study, Harke and colleagues (113) reported on 28 patients with PHN of more than 2 years duration. Long-term pain relief was achieved in 23 patients (82%), eight of whom became completely pain free. Four patients with acute PHN noted immediate improvement, although perhaps as a result of the natural resolution of PHN.
Spinal Cord Injury or Lesion
In patients with brachial plexus damage or complete avulsion of the nerve roots from the spinal cord, SCS will not relieve pain because the relevant neurons have been destroyed or severed (114). These patients can be identified through radiography and neurologic examination. In patients with less severe injuries, the extent and location of damage helps determine whether SCS will be efficacious. Still, patients with the same diagnosis may experience widely varying responses to SCS. This truth highlights our incomplete understanding of the etiology and pathophysiology of neuropathic conditions
and underscores the importance of trial stimulation before permanent implantation. However, even trial stimulation cannot guarantee longer-term success, as proven by the 60% to 70% success rate obtained after successful trial. The literature contains a number of case studies and reports from several groups on SCS for the treatment of spinal cord injury or lesion.
and underscores the importance of trial stimulation before permanent implantation. However, even trial stimulation cannot guarantee longer-term success, as proven by the 60% to 70% success rate obtained after successful trial. The literature contains a number of case studies and reports from several groups on SCS for the treatment of spinal cord injury or lesion.
In a series of 109 patients followed between 1978 and 1986, 15 with an incomplete spinal cord lesion, Meglio and colleagues (104) found no clinical usefulness for SCS in treating pain associated with central deafferentation. Cioni and colleagues (115) instituted trial stimulation in 25 paraplegic patients suffering from intractable pain due to a spinal cord lesion. Fewer than half (40.9%) reported pain relief and underwent permanent implantation. Just over 3 years later, only 18.2% had a greater than 50% reduction in their pain.
Lazorthes and co-workers (116) followed 692 patients with neurogenic pain, 17 with brachial plexus lesions and 101 with spinal cord lesions, for 20 years. Their patients were enrolled as two study groups: one a series of 279 patients and the other of 413 patients. Percutaneous SCS trials lasting 3 to 14 days were used to screen candidates. One month after implantation, the success rates were 86% and 85% for the two groups, respectively. At a mean of 10 years follow-up, the success rates were 54% and 52%. For the period from 1984 to 1990, the success rate in 301 patients was higher, 68% and 60%, respectively. The researchers concluded that SCS had therapeutic value in treating neurogenic pain secondary to partial deafferentation. For upper limb pain, they recommended ipsilateral radicular stimulation, and in cases of brachial plexus avulsion, herpes zoster, and pain of spinal or cerebral origin, they recommended thalamic stimulation if SCS failed. Their recommendations illustrate the evolution of electrical stimulation, and the newly emerging techniques of motor cortex and deep brain stimulation. In reviewing 25 years of experience with 1,336 cases of motor disorders, including 303 spinal cord injuries, Waltz (103) cited numerous technical advances, as well as our progressive understanding of neurophysiologic mechanisms, as the keys to improving the efficacy of SCS.
Weighing Potential Risk Versus Benefit
Any pain treatment plan must balance benefit against risk. Consequently, the classic chronic pain treatment continuum begins with less invasive and costly options and progresses if they fail (117). In this context, SCS has usually been relegated to the status of last resort. The decision to choose SCS ultimately falls to the patient and physician, who together can discuss individual circumstances. The potential benefits of SCS are listed in Table 41-4. It should be noted that the NTAC, in drawing up its practice parameters for SCS, classified its reported benefits as useful for information, in part because there are no generally accepted standards for measuring many of the benefits and only two RCTs available for consideration.
The most obvious potential benefit of SCS is pain relief. The criterion of 50% pain reduction has been used as a definition of success for decades (139) but lacks standardization because pain itself fluctuates, and the perception of pain is highly subjective and idiosyncratic. The commonly used visual analog scale (VAS) creates an individual framework for the assessment of pain over time, with a reduction in score considered a measure of success. Yet, patients reporting relatively modest reductions in VAS may have disproportionately greater gains in function or decreases in pain medication. For example, in one prospective study, only 26% of patients said SCS conferred 50% or greater reduction in pain, yet 70% said the therapy helped them and they would recommend it (130). Two years post implantation, two-thirds of these patients had reduced or stopped their narcotic medications. In another study, 55% of patients met the pain reduction criterion, but 90% had reduced or stopped their medication (140). Given the intractable nature of the chronic pain syndromes treated with SCS, patients may view any reduction in pain as advantageous, particularly if it allows functional improvement and less reliance on pain medications. Both RCTs (of treatment for FBSS) found significantly greater pain relief with SCS than with alternative treatments (reoperation or CMM) (57,58).
Numerous studies, including one RCT, have documented pain relief (or reduced need for medication) due to SCS for a variety of indications (see North et al., (6) for a comprehensive bibliography). The RCT compared SCS to lumbosacral spine surgery for chronic pain. Patients randomly assigned to reoperation required significantly more opiate medication than those assigned to SCS (p <0.025) (57). One prospective, multicenter study of SCS for chronic back and extremity pain found no reduction in medication among patients treated with SCS (118). However, all pain and quality-of-life measures showed statistically significant improvement during the year after implantation. This included improvements measured by the average pain VAS, the McGill Pain Questionnaire, the Oswestry Disability Questionnaire, the Sickness Impact Profile, and the Beck Depression Inventory. Using 50% pain relief as the definition of success, SCS managed pain in 55% of the patients.
In their review, the NTAC (6) found 13 long-term and 12 short-term follow-up studies that demonstrated increased ability to undertake ADLs or improved QOL for patients treated with SCS. Seven years after SCS implantation, a majority of patients in one retrospective, consecutive series of 205 patients had maintained improvements in ADLs (51). In a retrospective long-term (mean 37.5 months) follow-up of 81 patients with SCS, 80% reported an improvement in QOL (123). They experienced significant reductions in the Oswestry Disability Index (p <0.01), the Hospital Anxiety and Depression (HAD) Index (p <0.01), and VAS scores (p <0.001). In the same study, patients in two “control” groups, who had no trial of SCS or a failed trial of SCS, deteriorated over time. Spinal cord stimulation reduced the frequency of awakenings during the night in 20 patients treated for FBSS (124). Ten of these patients, (59%) said SCS had increased their ability to participate in social activities or pursue a hobby. Budd noted improvements in sleep, mood, and mobility in a retrospective study of 20 patients treated for FBSS (120).
No standard measure of patient satisfaction with treatment exists. In one study of SCS for FBSS, 53% of patients expressed satisfaction with the procedure at 2.2 years follow-up and 47% at 5 years postoperatively (5). A nationwide survey by the health insurer in Belgium found that among 70 patients with a mean follow-up of 3.5 years, the effect of SCS was judged good or very good by 52% of the patients (134). Among 153 patients followed for 4 years after implantation in Belgium, 68% rated their result as excellent to good (125). One convincing measure of patient satisfaction with SCS is the fact that in the two RCTs, patients were significantly less likely to cross over to reoperation or CMM than to stay with SCS (57,58).
Several studies have noted fewer symptoms of depression in patients successfully treated with SCS (6,16,101,118,123,137). Burchiel and colleagues (137), using the depression subscale of the Minnesota Multiphasic Personality Inventory (MMPI), noted that increased depression scores correlated negatively with post-treatment pain status as measured by VAS. However,
May and co-workers (123) did not find any correlation between initial depression scores and subsequent levels of pain relief. Two prospective studies offer additional evidence. Oakley and Weiner (16) observed a trend toward improvement (p <0.06) in the Beck Depression Inventory scores, which dropped from 13.18 pre-implant to 5.18 post-implant, at an average follow-up of 7.9 months in patients being treated for CRPS. Burchiel et al., (118) measured a significant (p = 0.02) decline in the Beck Depression Inventory score 1 year after implantation in 70 patients being treated for chronic back and extremity pain.
May and co-workers (123) did not find any correlation between initial depression scores and subsequent levels of pain relief. Two prospective studies offer additional evidence. Oakley and Weiner (16) observed a trend toward improvement (p <0.06) in the Beck Depression Inventory scores, which dropped from 13.18 pre-implant to 5.18 post-implant, at an average follow-up of 7.9 months in patients being treated for CRPS. Burchiel et al., (118) measured a significant (p = 0.02) decline in the Beck Depression Inventory score 1 year after implantation in 70 patients being treated for chronic back and extremity pain.
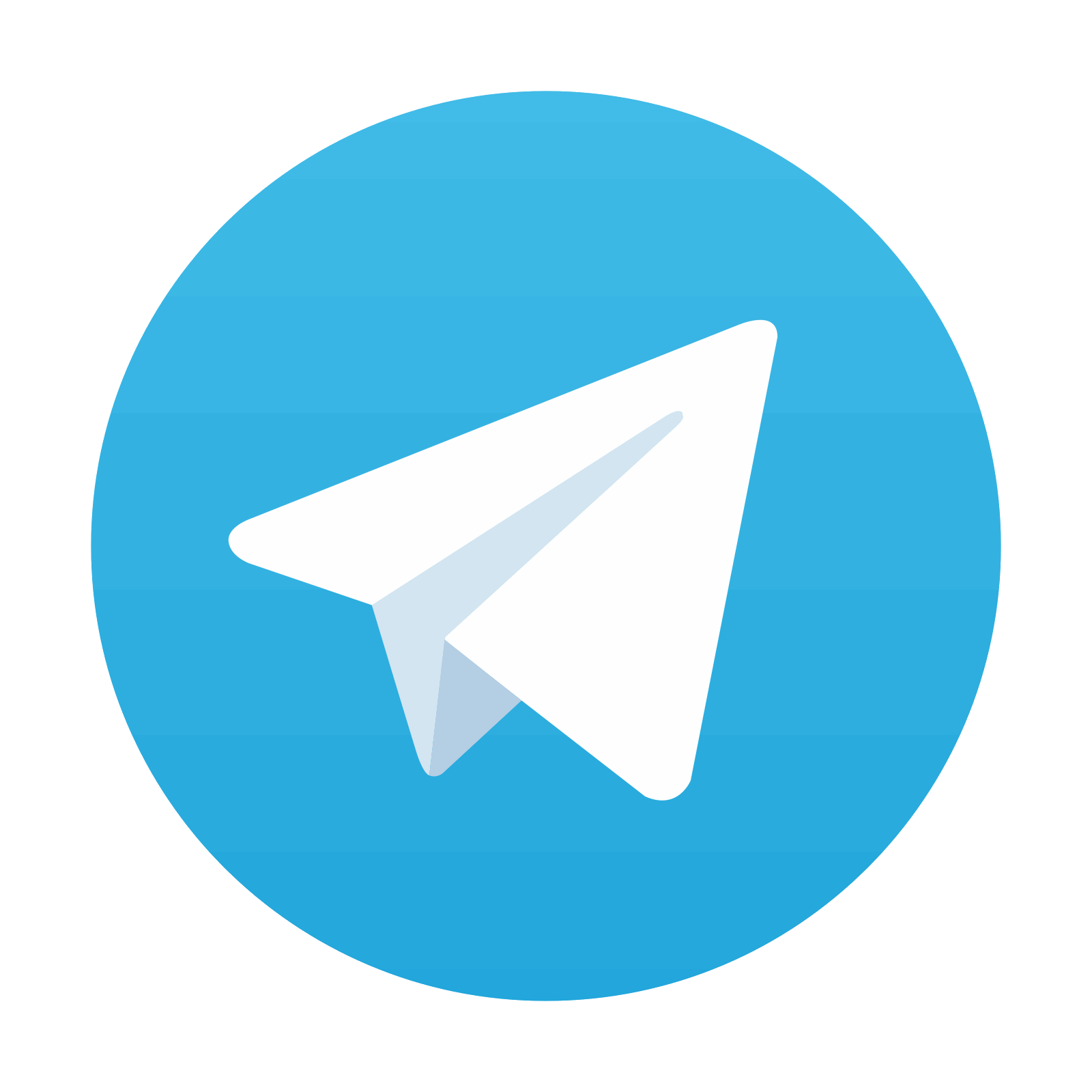
Stay updated, free articles. Join our Telegram channel

Full access? Get Clinical Tree
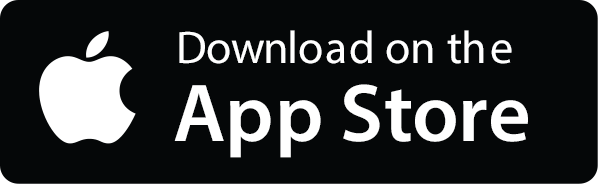
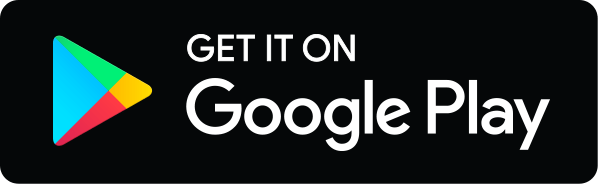