Neurophysiology of Migraines
Jean Schoenen
Sheena Aurora
Migraine is a disorder in which central nervous system (CNS) dysfunction might play a pivotal role. Because there are no consistent structural disturbances, clinical neurophysiology methods seem particularly suited to study its pathophysiology. This chapter reviews the neurophysiologic studies that have provided an insight to migraine pathogenesis. Results are in part contradictory, which may be because of the methodology, patient selection, or timing of study. Nonetheless, quantitative electroencephalographic (EEG) and magnetoencephalography (MEG) recordings during migraine attacks provide strong, albeit indirect, evidence favoring the occurrence of spreading cortical depression during attacks of migraine with, and possibly without, aura. Evoked cortical potential and nociceptive blink reflex studies demonstrate that lack of habituation during repetitive stimulation is a reproducible CNS dysfunction interictally in both migraine with and without aura. Transcranial magnetic stimulations (TMSs) show excitability changes of the visual cortex. The interictal migrainous CNS dysfunction is likely to play a role in migraine pathogenesis, has a familial character, and undergoes periodic modulations with quasi-normalization just before and during an attack as well as after treatment with certain prophylactic agents. In addition, neurophysiologic methods have revealed subclinical abnormalities of cerebellar function and neuromuscular transmission that may improve phenotyping of migraineurs for genetic and therapeutic studies.
INTRODUCTION
The exact pathogenesis of migraine remains to be determined. Migraine is an episodic disorder involving head pain and cortical phenomena without structural abnormalities. Therefore, investigations aimed at studying the function of the brain are particularly useful in providing an insight into migraine pathophysiology. Some studies that have been reviewed in the literature support the concept of central neuronal dysexcitability as a pivotal physiologic disturbance predisposing to migraine (1). Whether this dysexcitability is genuine neuronal hyperexcitability or lack of habituation of neuronal responses during repetitive stimulations remains a matter of debate.
Several psychophysical studies of the visual system tend to support this hypothesis: more intense illusions to grating patterns (2), faster low-level performance on psychophysical visual tests (3), and the known clinical hypersensitivity to environmental light stimuli (4). From these findings, it was proposed that there could be a generalized interictal hyperexcitability of the cerebral cortex in migraine, more pronounced in visual areas because of its neuronal density (5), and possibly favoring the occurrence of spreading depression, which is thought to be responsible for the migrainous aura (6). By contrast, other psychophysical tests of the visual system were thought to reflect hypoexcitability of the visual cortex (7).
The reasons for the neuronal dysexcitability may be multifactorial. Most recently, abnormal function of calcium channels has been introduced as a potential mechanism. Mutant voltage-gated P/Q-type calcium channel genes influence neurotransmitter release, possibly of excitatory amino acid systems or inhibitory serotonergic systems, and have been shown to decrease the threshold for cortical spreading depression (CSD) (8). However, mutations of these channels have been found up to now only in Familial Hemiplegic Migraine, and not in the common forms of migraine where more subtle, probably genetically determined, abnormalities of the molecular mechanisms controlling neuronal excitability and metabolism may be at work.
The methods of clinical neurophysiology are particularly appropriate for the study of migraine pathophysiology because they are atraumatic and able to detect functional abnormalities. During the past decade numerous neurophysiologic studies have yielded interesting, though partly conflicting, results (9). Some of them represent abnormalities that are undoubtedly related to the
pathogenesis of migraine; others reflect incidental, more general neuronal dysfunctions that may improve phenotyping of migraineurs. This chapter provides an overview the neurophysiologic studies in migraine with emphasis on the more recent ones.
pathogenesis of migraine; others reflect incidental, more general neuronal dysfunctions that may improve phenotyping of migraineurs. This chapter provides an overview the neurophysiologic studies in migraine with emphasis on the more recent ones.
ELECTROENCEPHALOGRAM
Electroencephalography (EEG) was one of the first techniques undertaken to discern physiologic differences between migraineurs and controls. A recent review suggests that EEG is not a valuable as a diagnostic tool for primary headache disorders (10). In contrast, the technique provided some useful information to be used in the research setting. This subject has been detailed elsewhere (11,12) and only highlights are presented here. Researchers have utilized four paradigms to study the EEG in migraine, namely background rhythm, photic or “H-response,” mapping techniques using spectral analysis, and MEG. Slowing of background rhythm, both generalized (13,14) and focal (12,15, 16, 17), is described in children and adults during a migraine, but these findings are not universally reported (18, 19, 20). The enhanced photic drive response on the EEG “H-response,” which was thought to be characteristic of migraine (21), has been confirmed by spectral analysis (22,23). The specificity of the “H-response,” however, is low because it may occur with other primary headache disorders and in normal subjects (24).
Brain mapping using quantitative-topographical EEG (25) has been applied to study migraine. Briefly, unilateral reduction of α activity was observed during attacks and interictally within 3 days of an attack in migraine with visual aura in adults (24) and in children (26). Unilateral reduction of α power was also found during attacks of migraine without aura, usually on the side of the headache, and in patients with menstrual migraine up to 24 hours before the attack (24). Some studies showed an increase in α1 power (25,27,28), but a study in children did not document a difference from controls (29). Finally, nonspecific abnormalities on EEG were modified with flunarizine in one study (30).
Seven-channel MEG studies revealed long-lasting suppressions in spectral power of all frequencies faster than 1 Hz, large-amplitude waves, and prolonged DC shifts during attacks of migraine with aura (8 of 13 patients) or without aura (1 of 2 patients) (18,31).
These electromagnetic phenomena are reminiscent of those described with experimental spreading cortical depression (32). To date, CSD has been recorded successfully in animal models only (33). More recently using the whole head MEG, which permits precise localization of signals (34), DC MEG shifts were observed in migraine subjects during visually triggered aura and in a patient studied during the first few minutes of spontaneous aura. No DC-MEG shifts were seen in control subjects. This is additional evidence supporting the primary neural basis of migraine with aura and confirms that spreading cortical depression is the likely culprit for migraine aura.
Evoked Potentials
Visual Evoked Potentials
Various paradigms have been used to study visual evoked responses in migraine. Abnormal steady state response evoked by a sine-wave visual stimulus (SVEP) was seen in migraineurs, and improved after administration of propranolol (35, 36, 37). Using flash or pattern reversal (PR) the amplitude of visual evoked responses in migraineurs between attacks was found normal in most studies, but in some increased or decreased compared to controls (9). Amplitude asymmetries were reported in several studies (38, 39, 40, 41). When sequential blocks of averaged visual evoked potentials (VEPs) are recorded during continuous PR stimulation, migraineurs, with or without aura, are as a group characterized by a lack of habituation, or even potentiation, between the first and subsequent blocks of responses (24,42). This habituation deficit is of interest because it has been found by several independent groups for various modalities of sensory stimulation, including for event-related potentials and nociceptive brainstem reflexes (see below), has a familial character (43), and normalizes during attacks (44) and preventative treatment with β-blockers (45).
Auditory Evoked Potentials
Brainstem auditory evoked responses and long-latency auditory evoked cortical potentials were found normal in most studies of migraine patients (9). More recently, however, and in agreement with VEP studies, strong interictal dependence of the auditory evoked potentials (AEPs) on stimulus intensity was demonstrated in migraine (46) and attributed to deficient habituation at all, but more so at high, stimulation intensities (47). Two independent studies (43,48) found evidence for a familial influence on IDAP in migraineurs, pointing to a genetic background.
Gating of the auditory P50 response, which is thought to be generated subcortically, was markedly reduced in migraine without aura (49). Contrary to schizophrenic patients in whom P50 gating is deficient with a low-amplitude first response, migraineurs had a normal first P50.
Somatosensory Evoked Potentials
Subtle somatosensory evoked potential (SSEP) abnormalities were reported in some migraine studies: prolonged N13 latency interictally (50), reduced P22/N30 amplitude
interictally (51), prolonged N19 latency, and reduced amplitude during the aura (52).
interictally (51), prolonged N19 latency, and reduced amplitude during the aura (52).
In concordance with studies of VEPs and AEPs, Ozkul and Uckardes (53) found potentiation of median nerve SSEP N20 component in migraineurs, contrasting with habituation in healthy controls.
A recent study analyzed high-frequency oscillations (HFO) (600 Hz) embedded in the N20 component of median nerve SSEP (54). The early HFO burst, which is thought to reflect action potential traffic in thalamo-cortical projections, was reduced in migraine with and without aura compared to normal controls, and the late burst, attributed to cortical inhibitory interneurons, was normal.
Event-Related Potentials
Contingent Negative Variation
Contingent negative variation (CNV) is a slow negative cortical potential appearing during a reaction time task with a warning and an imperative stimulus. Consistent studies from different laboratories have shown that CNV amplitude is increased in migraineurs between attacks compared to healthy volunteers or tension-type headache patients (55, 56, 57, 58, 59). The difference is more pronounced for the early CNV component, which is modulated by noradrenergic systems and attention. Taking age as a variable into account, Kropp et al. (60) showed a decrease in early CNV amplitude with aging in healthy volunteers but not in migraineurs, which they interpreted as a disturbance of cerebral maturation. A strong familial influence on CNV parameters was reported by Siniatchkin et al. (61), who found abnormalities not only in migraineurs but also in healthy subjects with a positive family history for migraine (62).
Like modality-specific evoked potentials, CNV also shows a lack of habituation between attacks (59,63), especially the early component (59,64), which normalizes during the attack (64,65). Because normal CNV habituation in healthy volunteers is reached only in adulthood, it was proposed that the habituation deficit in migraineurs might reflect a disturbance of cerebral maturation (60).
The early CNV component increased in amplitude during the days preceding the attack (56,61,64,65), but decreased abruptly just before and during the attack (56,64, 65,66).
Studies of the P300 component of the classical “oddball paradigm” gave conflicting results. In a paradigm using visual stimuli, however, habituation, as assessed by the increase of latency during trial repetition, was significantly reduced in migraineurs interictally and normalized during an attack in parallel with a decrease in platelet serotonin content (67, 68, 69). The P3a component in the passive “oddball” paradigm reflects automatic processing of a “novel” stimulus. In one study, its normal habituation pattern was replaced by potentiation in migraine (70).
Transcranial Magnetic Stimulation
TMS has been developed to noninvasively study cortical physiology. Both the motor and occipital cortices have been investigated with TMS in migraine. The results of these studies are synoptically presented in Tables 39-1 and 39-2.
Transcranial Magnetic Stimulation of Motor Cortex
Most TMS studies of motor cortex in migraineurs have shown either normal or an increased motor threshold (MT) both in migraine with (71, 72, 73) and without aura (74) as well as in Familial Hemiplegic Migraine (75). The only exception to this is the study by van der Kamp et al. (76), who found reduced MT between attacks of patients with migraine with or without aura. Other parameters such as cortical silent periods and intracortical inhibition tested with paired TMS (77,78) were also normal, except in one study (79), where the cortical silent period was significantly shorter in migraine with aura patients than in controls.
Transcranial Magnetic Stimulation of Occipital Cortex
Using TMS to study the occipital cortex is perhaps more relevant to migraine because abnormal excitability of the occipital cortex may underlie either spontaneous or visually triggered migraine aura (38). Occipital cortex excitability in migraine has been evaluated by the generation of phosphenes by TMS of occipital cortex and by the modulatory effect of TMS on visual perception or on VEPs.
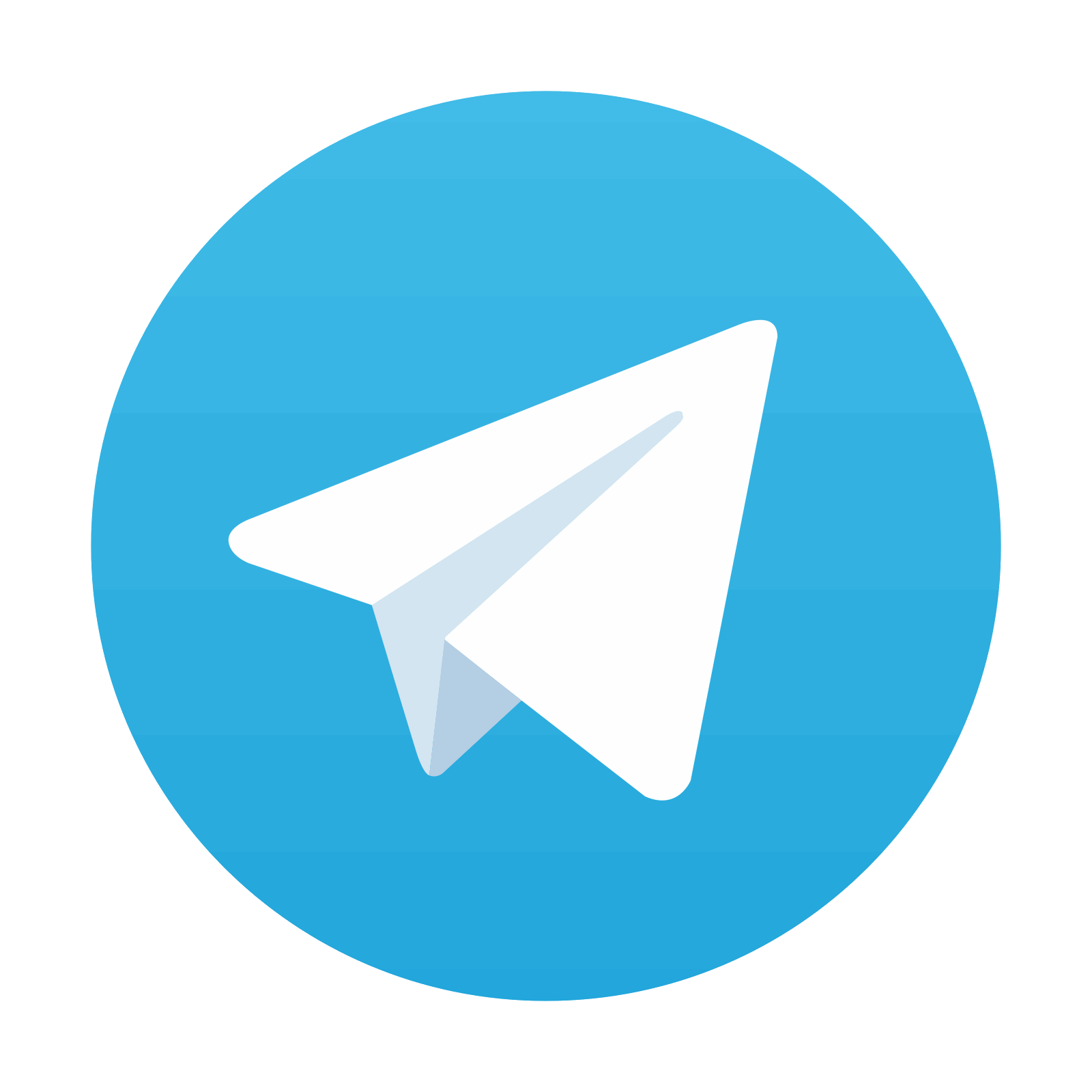
Stay updated, free articles. Join our Telegram channel

Full access? Get Clinical Tree
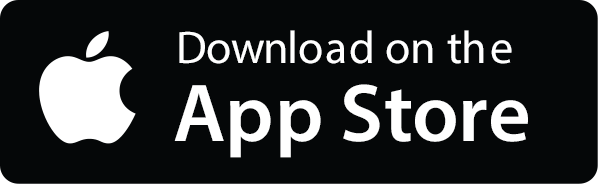
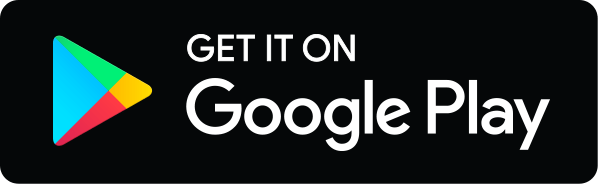
