FIGURE 122.1 Localization of neuromuscular disorders, with the most important exemplars for each of the nine localizations. ALS, amyotrophic lateral sclerosis; CIDP, chronic inflammatory demyelinating polyneuropathy.
TABLE 122.1 Salient Features of Neuromuscular Disorders |
![]() |
TABLE 122.2 Electrodiagnostic Features in Nonfocal Neuromuscular Disorders |
![]() |
Brain imaging may help establish a diagnosis in stroke or CPM; in other conditions, such as hypoxia or even acute spinal cord ischemia, imaging may be normal or nondiagnostic. If the cause of apparent weakness remains in question, electrodiagnostic testing is of value in suggesting or helping to exclude a neuromuscular localization.
ELECTRODIAGNOSTIC STUDIES
Nerve conduction studies (NCSs), late wave analysis, needle electromyography (EMG), and repetitive stimulation are the standard procedures available to assist in the diagnosis of neuromuscular disorders, and can be performed using portable equipment in the intensive care setting. Table 122.2 summarizes the typical electrodiagnostic findings in disorders of nerve, neuromuscular junction, and muscle.
Nerve Conduction Studies
Motor nerves that are usually tested by NCSs include the tibial, peroneal, and ulnar nerves (11). Figure 2A illustrates the typical motor nerve conduction procedure and normal response from stimulation of the tibial nerve. The nerve is stimulated at two or more sites, and the response is recorded with surface electrodes over a muscle innervated by that nerve. The muscle response is called a compound muscle action potential (CMAP), because it is the sum of all muscle fiber action potentials activated by nerve stimulation. The stimulus at each site is gradually increased until no further increase in CMAP amplitude is seen. This indicates that all of the motor neurons capable of generating an action potential have been activated. The latency to the onset of the CMAP reflects the speed of conduction in the fastest nerve fibers. Motor nerve conduction velocity is calculated by measuring the distance between the sites of stimulation, and dividing that distance by the proximal minus the distal latency, which is the time it takes for the stimulus to traverse that nerve segment. By itself, motor nerve conduction can be diagnostic of focal or generalized demyelinating neuropathy (see Fig. 2C,D). Interpreting a low-amplitude response (see Fig. 2B) requires additional studies, since low CMAP amplitude can reflect not only loss of nerve axons or conduction block, but also defective neuromuscular transmission or loss of muscle fibers.
Sensory nerves are mostly evaluated on electrodiagnostic studies are radial, ulnar and sural nerves (11). In orthodromic sensory NCSs, the skin is stimulated and a response is recorded over the nerve. Antidromic studies are more commonly performed by stimulating the nerve and recording over the skin. The result in either case is a sensory nerve action potential (SNAP), with a latency that reflects conduction in the fastest nerve fibers, and an amplitude that is proportional to the number of functioning nerve fibers. The normal SNAP amplitude is small and measured in microvolts. Abnormal SNAPs are indicative of pathology distal to dorsal root ganglion—either plexopathy or neuropathy. SNAPs are normal in radiculopathy if the pathology spares the dorsal root ganglia in CNS disorders as well as in disorders of neuromuscular transmission and muscle. In a patient with weakness and abnormal motor NCSs, small or absent SNAPs suggest sensorimotor neuropathy or plexopathy.

FIGURE 122.2 Typical motor nerve conduction findings. The sweep speed is 5 ms/div in all traces, but the gain is 5 mV/div in (A) and (C), 1 mV/div in (B), and 500 μV/div in (D). A: Tibial motor nerve conduction in a normal subject. The drawing shows the two sites of stimulation over the posterior tibial nerve at the ankle and knee, and the sites of surface electrodes recording the response of the medial plantar muscle group. The two waveforms are the responses to stimulation at the ankle and knee. Conduction velocity is calculated as the distance between proximal and distal sites of stimulation divided by the time it takes for the stimulus to travel between the sites of stimulation (PL minus DL). B: Tibial motor nerve conduction in a patient with axonal sensorimotor neuropathy. CMAP amplitudes are less than 2 mV, with normal being greater than 4 mV. The amplitudes are similar with proximal (knee) and distal (ankle) stimulation. The conduction velocity is slightly slow, but is greater than 80% of the lower limits of normal. C: Peroneal motor nerve conduction study in focal nerve compression. CMAP amplitude drops sharply with nerve stimulation above the site of compression, at the fibular head. This conduction block is caused by focal nerve demyelination. Because there is no damage to axons, the nerve responds normally to stimulation distal to the area of demyelination. Conduction velocity is slow across the fibular head. D: Acquired diffuse demyelinating polyradiculoneuropathy. Multifocal demyelination affects some neurons more than others, resulting in prolonged and irregular CMAPs. Conduction velocities less than 70% of the lower limits of normal are also diagnostic of demyelination. DL, distal latency; PL, proximal latency; CMAP, compound muscle action potential.
Action potentials in motor neurons, called F waves, are normally generated at the axon hillock and travel distally to nerve terminals in muscle; when the nerve is stimulated in the course of NCSs, action potentials travel both orthodromically (distally) and antidromically (proximally) from the point of stimulation. The antidromic action potential depolarizes the axon hillock and, in a minority of neurons, this depolarization generates another action potential that travels orthodromically down the nerve. F wave are small and late muscle action potential can be recorded from most muscles. Increased F-wave latency, when combined with normal distal motor nerve conduction, indicates disease in the proximal portions of the nerve. Abnormal or absent F waves are the earliest electrophysiologic manifestation of GBS (12,13).
In patients whom a neuropathic process is suspected, electrodiagnostic studies begin with NCSs in bilateral lower extremities and one upper extremity preferably on the side that is more symptomatic if the symptoms are asymmetric. In myopathies, NCSs are not necessary and are only required when there is severe atrophy of distal muscles of the affected limb. In disease processes where there is damage to myelin sheath (demyelination), general findings on NCSs include decrease conduction velocity, prolonged F wave, prolonged distal latency, reduced SNAP, and reduce/absence of CMAP. There are two other measures; temporal dispersion and conduction block that help differentiate between a uniform and a segmental process. In uniform demyelinating processes (Hereditary Neuropathies) conduction block and temporal dispersion are not seen.
EMG entails inserting a recording needle electrode into a muscle and observing (a) insertional activity consisting of action potentials generated by mechanical deformation of muscle fibers; (b) resting activity, which in normal muscles is only seen when the needle is near an end plate; and (c) voluntary activity, which consists of motor unit action potentials (MUAPs). The motor unit consists of a motor neuron and all the muscle fibers innervated by it. The MUP is the sum of action potentials of all the muscle fibers in the vicinity of the recording needle electrode that are activated by a single motor neuron. Insertional and resting activity does not require patient cooperation, and can be observed in comatose or uncooperative patients. One to 3 weeks after denervation associated with axon damage, denervated muscle membranes become hypersensitive and discharge both spontaneously and in response to needle insertion, causing increased insertional activity and the of positive sharp waves and fibrillations, which are spontaneous or needle movement–induced discharges of single muscle fibers. Similar abnormal spontaneous activity can be seen in disorders of muscle, particularly in the inflammatory myopathies, perhaps as a result of functional denervation caused by muscle fiber damage. If the patient is cooperative or happens to activate the muscle during the needle EMG study, additional information can be obtained by observing MUP activity. MUP morphology is of diagnostic value: high-amplitude, long-duration motor unit potentials indicate denervation with reinnervation, and low-amplitude, polyphasic motor unit potentials suggest primary disease of muscle or neuromuscular junction. Observation of motor unit recruitment with increasing effort can be diagnostic of denervation in the absence of any other abnormality. With denervation, MUP firing frequency increases with increasing effort, but insufficient numbers of different MUPs are recruited. Conversely, rapid recruitment of small polyphasic MUPs suggests myopathy or a disorder of neuromuscular transmission. Increased variability of the shape of a single MUP suggests a disorder of neuromuscular transmission.
EMG in neuropathic processes should begin with distal muscles since those muscles are affected earlier in the course of the disease. If a motor neuron disease is of concern, both distal and proximal muscles of upper and lower extremities should be evaluated (11). Electrodiagnostic findings in motor neuron disease include low motor amplitude, normal distal motor latencies, normal conduction velocities, and normal sensory NCS. On EMG, patients have spontaneous muscle activity, for example, fibrillation, fasciculation, or positive sharp waves with small or large MUAPs (EMG-NCV). In neuromuscular junction disorders MUAPs can be normal or polyphasic with small duration and low amplitude which is secondary to decrease in neuromuscular transmission (11).
Disorders of neuromuscular transmission may be postsynaptic, as is the case in myasthenia gravis or most pharmacologic neuromuscular blocking agents, or presynaptic, as in Lambert–Eaton myasthenic syndrome (LEMS) and with exposure to botulinum toxin. Repetitive nerve stimulation (RNS) results are dependent on safety factor of the neuromuscular junction. Safety factor is the number of activated acetylcholine receptors required to produce an action potential, large enough to depolarize the membrane and subsequently muscle contraction (14). In presynaptic disorders, acetylcholine release from the presynaptic membrane is not enough to activate the postsynaptic receptors available. On the opposite side, in postsynaptic disorders, there is enough acetylcholine released from presynaptic membrane but not enough receptors available in postsynaptic membrane to be activated and generate the action potential required for muscle contraction. RNS studies are helpful in distinguishing these two categories. RNS is performed at a slow rate (2–3 Hz) and a fast rate (20–30 Hz) (11). Repetitive supramaximal stimulation of a motor nerve at a constant rate for five to ten stimuli is a simple way to assess for deficits of neuromuscular transmission. The resultant CMAP amplitudes should be constant. In patients with neuromuscular disorders, given multiple slow stimulations repetitively, the amount of acetylcholine released from presynaptic membrane decreases with each stimulation, with subsequent lower CMAPs and NMJ block (11).
Progressive decrements in amplitude across the first four to five responses with 3-Hz stimulation are seen in both postsynaptic and presynaptic disorders of neuromuscular transmission. Progressive increments in response at rates of stimulation above 20 Hz suggest a presynaptic neuromuscular block, as in LEMS, or botulism. An alternative—and more tolerable—means to look for this incremental response is to stimulate the nerve supramaximally just before and just after 10 to 60 seconds of sustained voluntary contraction of the muscle being tested. An increase in amplitude after exercise—postexercise facilitation—is seen in pre- and postsynaptic disorders; however, increases greater than 100% are seen only in presynaptic disorders (15). Repetitive stimulation is important in documenting residual neuromuscular blockade as a cause of weakness in ICU patients. RNS should not be performed close to central venous catheters or pacemakers.
CLINICAL MANAGEMENT
Prehospital Management
Neuromuscular disorders can present acutely as a neurologic emergency. The first responder priority is to assess the patency and stability of the patient’s airway, breathing, and circulation (ABC’s). A brief neurologic assessment must be made in order to rule out major neurologic disorders such as acute strokes, subarachnoid hemorrhage, seizures, toxin exposures, etc.
A detailed history helps formulating a diagnosis by localizing the disorder and understanding the underlying pathophysiology. Patient should be interviewed regarding presenting symptoms, the pace of progression, the pattern of weakness (ascending vs. descending), and diurnal variation and fatigability (steady progression vs. fluctuating pattern). The emergency neurological life support (ENLS) algorithm is a useful guide to assist the physician with deducing the level of the lesion (16).
Evaluation for Intensive Care Unit Admission
Indications for intensive care of patients with neuromuscular disorders include airway protection, respiratory failure, and autonomic instability. It is worth pointing out that many of the signs typically associated with respiratory failure in patients with obstructive pulmonary disorders, such as increased respiratory excursion, may not be seen in patients with neuromuscular causes of respiratory failure. Instead, difficulty clearing secretions, staccato speech and repiratory symptoms such as air hunger, tachypnea and shallow breathing are characteristic. An inability to lay supine and paradoxical respirations are manifestations of severe diaphragmatic weakness. Confusion and somnolence with rising partial pressure of carbon dioxide (PaCO2) may lessen respiratory distress and make recognition of respiratory insufficiency more difficult. Bedside tests of respiratory function that indicate a need for respiratory support include the inability to count to 20 on one breath, a forced vital capacity of less than 15 mL/kg, a negative inspiratory force of less than 25 cm H2O, and a positive expiratory force of less than 40 cm H2O (17–20). Patients with facial weakness may have difficulty performing bedside tests, necessitating greater reliance on blood gas monitoring. Intubation may be required earlier in patients with dysphagia in order to protect the airway.
Autonomic dysfunction and pain can be features of the GBS and are discussed under that topic below. Patients who are immobile from weakness or other causes may suffer compressive neuropathies. The peroneal nerve at the head of the fibula and the ulnar nerve at the elbow are especially vulnerable. Constant attention to positioning is required to prevent pressure palsies. Pneumatic compression devices should not cover the proximal fibula.
Neuromuscular disorders do not affect mentation, and it is important to maintain communication to inquire about discomfort and pain, involve patients in care and decision making, and support them through what can be a terrifying experience. Bowes, a physician who recovered from ventilator-dependent GBS, gives a personal account of her experience that is instructive for caregivers (21). Additional aspects of the intensive care of neuromuscular disorders are discussed below with reference to specific disorders.
Table 122.3 lists some of the neuromuscular conditions that can cause respiratory failure. Weakness can develop gradually, and initial management might not require immediate need for intensive care, except when intercurrent illness, such as aspiration pneumonia, complicates the hospital course. ALS inevitably leads to respiratory failure. In a minority of patients with ALS respiratory insufficiency is the presenting symptom, and leads to intensive care admission before the diagnosis is made. The diagnosis is suggested by finding fasciculations, atrophy, weakness, and hyperactive reflexes in the absence of sensory loss, extraocular muscle weakness, or autonomic dysfunction, and by excluding alternative causes, such as spinal cord compression or neuropathy. Electrophysiologic studies can be helpful. NCSs document normal SNAPs, and needle EMG reveals denervation in muscle of three or more extremities or two extremities and bulbar muscles. Needle EMG can also document diaphragm denervation. Early in the course, clinical and electrophysiologic findings may not suffice for definitive diagnosis.
Respiratory weakness is seen in some muscle disorders, and is a terminal event in Duchenne muscular dystrophy. Respiratory weakness can be the presenting feature of adult-onset acid maltase deficiency, a glycogen storage disease (22), and it is a feature of some of the congenital myopathies and muscular dystrophies. Respiratory weakness can occur in inflammatory myopathies in the context of severe proximal limb weakness, and polymyositis associated with Jo-1 antibodies can be associated with interstitial pulmonary disease (23).
RESPIRATORY FAILURE
The main cause of respiratory failure in patients with neuromuscular disorders is muscle weakness, which can be significant in respiratory muscles, or causes inability to protect ones airway. In most causes this leads to intrathoracic infections, notably pneumonia. Although uncommon, respiratory failure can be the only presenting symptom in some of the neuromuscular disorders, however in most causes, it develops during the course of the disease (24–26).
TABLE 122.3 Neuromuscular Causes of Respiratory Failure |
![]() |
Three groups of muscles aid with inspiration; the diaphragm, which is responsible for 70% of inspiratory tidal volume and is innervated by phrenic nerve (C3–5): external intercostal muscles: accessory muscles.
Exhalation is assisted through two groups of muscles; internal intercostal muscles and abdominal muscles. Bulbar muscles are responsible for speech and swallowing (24).
Weakness of respiratory muscles prevents patients from taking deep breaths causing a decline in tidal volume and subsequently CO2 retention and hypercapnic/hypoxic respiratory failure.
Patients with neuromuscular disorders have reduced respiratory reserve and can decompensate quickly with any additional respiratory load.
In patients with skeletal abnormalities related to the underlying disease (kyphoscoliosis in some of muscular dystrophies) respiratory failure can appear early (24).
Upon assessing patients for respiratory status, it is important to identify which muscles are weak and the degree of weakness. Bulbar muscles weakness causes difficulty in mastication, swallowing, and maintaining airway, which increases the risk of aspiration pneumonia. Weakness of respiratory muscles, in particular the diaphragm, causes a decrease in inspiratory tidal volume causing atelectasis and eventually pneumonia.
Accurate diagnostic evaluation includes the following evaluation.
- Detailed history regarding similar symptoms in the past, prior intubation for respiratory failure secondary to muscle weakness, diplopia, swallowing difficulties, recent changes in voice.
- Detailed cranial nerve examination for evaluation gag reflex, cough, and tongue movements/strength as well as signs of swallowing difficulty like dystonia and drooling.
- Abdominal examination to evaluate paradoxical abdominal movements as a sign of diaphragm weakness and pending respiratory failure.
- Continuous pulse oximetry evaluation.
- Chest x-ray for evaluation of underlying pulmonary diseases.
- ABG: provides PO2 and PCO2 measures that help in assessing oxygenation and ventilation.
- Serial bedside spirometry to assess the progression of muscle weakness. Forced vital capacity, Maximum inspiratory pressure (also know as negative inspiratory pressure) and maximum expiratory pressure assess diaphragmatic strength, with a vital capacity of less than 15 mL/kg indicating an immediate risk of respiratory failure. Patient’s lack of cooperation for an accurate measurement can cause variable values reduced accuracy of measurement.
Noninvasive positive pressure ventilation (NIPPV) can be attempted in cases of mild respiratory dysfunction but only if rapid improvement in respiratory symptoms is expected with therapy (e.g., myasthenia gravis). Rapid sequence intubation is preferred for patients requiring an advanced airway. Succinylcholine may be ineffective at standard doses and no agents or short acting nondepolarizing agents are usually used (e.g., progressive muscular weakness). Autonomic instability might follow intubation and should be promptly treated (e.g., in GBS). Glycopyrrolate and atropine in sequence should be available to treat severe bradyarrhythmias (16,27).
The two neuromuscular disorders that most commonly lead to admission for intensive care are Guillain–Barré and myasthenia gravis. They are both autoimmune disorders and can cause rapidly progressive weakness and treatable. They will be examined in detail in this chapter.
GUILLAIN–BARRé SYNDROME
In 1859, Landry (28) described a syndrome of ascending paralysis progressing to respiratory paralysis and death. Guillain, Barré, and Strohl described the classic features of progressive weakness, sensory symptoms, and loss of tendon reflexes. They also described increased cerebrospinal fluid (CSF) protein without cells (albuminocytologic dissociation), a finding that distinguishes this disorder from poliomyelitis (29). An association with prior infection was established (30,31), and the concept that the disease was of autoimmune pathogenesis was supported by the development of an animal model—experimental allergic neuritis (EAN), in which inflammation and demyelination of peripheral nerves followed exposure to components of peripheral nerves (32).
Definition and Subtypes
GBS is an autoimmune disorder of peripheral nerves with progression over less than 4 weeks, which is manifested by weakness, reduced or absent tendon reflexes, and variable sensory and autonomic dysfunction (33). Subtypes have been distinguished based on the presence or absence of motor, sensory, and/or autonomic involvement, and on whether the predominant damage is to myelin sheaths or axons. Acute inflammatory demyelinating polyradiculoneuropathy (AIDP) is by far the most common subtype, accounting for more than 85% of cases in the United States (34). Patients have weakness and reflex loss, with varying degrees of sensory and autonomic involvement, and there is electrophysiologic and pathologic evidence of peripheral nerve demyelination. Patients who have no sensory loss and electrodiagnostic studies suggesting axonal rather than demyelinating pathology have acute motor axonal neuropathy (AMAN). This variety of GBS was first described in Asia, where it occurs epidemically following gastroenteritis caused by Campylobacter jejuni (35). Acute motor sensory axonal neuropathy (AMSAN) is diagnosed when there is both motor and sensory involvement and NCSs are consistent with early axonal dysfunction (36). The axonal forms of Guillain–Barré comprise less than 5% of cases in Europe and North America, but up to 47% of cases in China, Japan, and Central and South America (37). Acute dysautonomic dysfunction (5,38) and some forms of acute sensory neuropathy (39) are sometimes considered to be subtypes of GBS even though weakness is not a feature, since they run a similar time course, and are presumed to be autoimmune. The Miller–Fisher variant of GBS features ophthalmoplegia, ataxia, and areflexia (40). The Miller–Fisher variant may overlap with other forms of GBS (37,41).
Epidemiology and Pathogenesis
The incidence of Guillain–Barré is less than 1 in 100,000 in children, averages 1 to 2 per 100,000 in adults, and increases to 4 in 100,000 in adults over 75 years of age (42,43). In about two-thirds of patients, the disease follows an infection, most often a flu-like syndrome or gastroenteritis, by 1 to 8 weeks (44). When serologic studies are performed, evidence of infection with C. jejuni, cytomegalovirus, Epstein–Barr virus, Haemophilus influenzae, and Mycoplasma pneumoniae are found to account for the majority of cases (45,46); 5% follow surgery (31). Although 3% of cases are attributed to vaccination (44), only rabies vaccine is associated with a definite increase in the incidence of Guillain–Barré (47); influenza vaccination increase is minimal (47,48).
The pathology of AIDP consists of a multifocal inflammation of peripheral nerves, in which macrophages destroy the myelin sheath (49–52). This response is mediated by activated T cells (53). Direct binding of antibodies to Schwann cell membranes with subsequent complement-mediated destruction and secondary macrophage invasion may also play a role (54). Similar pathologic findings characterize EAN, which is considered to be a good experimental model for GBS (55). EAN can be initiated by injections of several myelin components, including myelin proteins P0 and P2, myelin basic protein, peripheral myelin protein-22 (PMP-22), and myelin-associated glycoprotein (MAG) (53). No specific myelin antibodies have been identified in the pathogenesis of AIDP.
The pathology of AMAN differs from that of AIDP in that macrophages target the axonal membrane in ventral (motor) roots and nerves at nodes of Ranvier, resulting in axonal rather than myelin damage (56,57). The pathology in AMSAN is similar to that of AMAN, except that the disorder affects both motor and sensory roots and nerves (36). Recent evidence suggests that AMAN may result from molecular mimicry. The capsule of the strains of C. jejuni implicated in patients with GBS contains a lipopolysaccharide with a structure similar to the GM1 ganglioside present in peripheral nerves. Antibodies to these strains of C. jejuni cross-react with peripheral nerve GM1 ganglioside, resulting in axonal dysfunction in experimental animals (58). Similarly, many patients with the Miller–Fisher variant have GQ1b and GT1a antibodies (59) that cross-react with Campylobacter lipopolysaccharides (60,61). Anti-GD1b antibodies have been associated with acute and chronic sensory neuropathies (37). Although there is evidence that molecular mimicry is a plausible explanation for some patients with Guillain–Barré polyneuropathy, the relationships are complex. Patients may have antibodies to several gangliosides, antibodies to a particular ganglioside can be associated with several different types of neuropathy, and infections with a specific organism may be followed by more than one kind of neuropathy (37).
Clinical Presentation and Differential Diagnosis
GBS is a subacute process. AIDP, the most common form of Guillain–Barré, presents with weakness and decreased or absent tendon reflexes. It is a non–length-dependent polyneuropathy, with proximal as well as distal weakness that usually begins in the legs and ascends, but can affect other regions first. Bilateral facial weakness occurs in half of the patients. Since symptoms can progress to oropharyngeal and respiratory weakness in 33% of patients, all patients require careful monitoring, as discussed below. Eye movements are usually spared, the exception being the Miller–Fisher variant. Sensory symptoms occur in 80% of patients. Sensory signs are less frequent and often mild, but, when prominent, can contribute significantly to disability (62). Pain and temperature sensation mediated by small unmyelinated neurons may be affected less than position and vibratory sensation, mediated by large myelinated fibers. Fifty percent have pain, either back and muscle pain that can be aggravated by movement, or neuropathic pain, such as burning paresthesias, sometimes with allodynia (pathologic sensitivity to touch). Tendon reflexes are lost early in the course of the disease; indeed, the diagnosis should be questioned if reflexes are retained. Autonomic dysfunction is present in 66% of patients, and can manifest with tachyarrhythmias or bradyarrhythmias, spontaneous or orthostatic hypotension, paroxysmal hypertension, abnormalities of sweating, urinary retention, or ileus (63). Fifty percent of patients stop progressing in less than 2 weeks, 80% by 3 weeks, and more than 90% by 4 weeks (64).
The differential diagnosis of patients presenting with subacute weakness includes disorders of the CNS, PNS, neuromuscular junction, and muscle. Although Babinski responses, brisk reflexes, and sensory level will usually distinguish spinal cord from peripheral nerve diseases, there is overlap because reflexes may be depressed acutely in CNS disorders; bladder dysfunction, while suggestive of CNS lesions, can be seen in Guillain–Barré; and CNS demyelination may occasionally accompany GBS (65). Conversely, GBS should be in the differential of patients with the locked-in syndrome when tendon reflexes are depressed. Toxic, drug-induced, and metabolic neuropathies may occasionally present over a similar time course as GBS. In patients without sensory disturbances, disorders of neuromuscular junction and muscle should be considered. Muscle disorders that can present with severe subacute or acute weakness include the periodic paralyses, hypokalemic myopathy, drug-induced myopathies, and inflammatory myopathies.
Diagnostic Workup
Evaluation should include appropriate imaging to exclude CNS disorders, routine laboratory studies, and lumbar puncture. Upper motor neuron signs should prompt an magnetic resonance imaging (MRI) study of the spine to rule out structural lesions (66). The CSF is normal during the first days of illness, after which there is a transient elevation of protein with minimal or no pleocytosis. Although this diagnostic finding can be very helpful, the increases in CSF protein levels can be delayed until the second week of the illness, allowing for a normal LP. A repeat LP should be considered if there are high suspicions regarding the diagnosis (67). A significant pleocytosis should raise the question of infection such as Lyme disease or West Nile virus (68); when the clinical picture is otherwise classic for Guillain–Barré, CSF pleocytosis should suggest human immunodeficiency virus (HIV) infection in which immune dysregulation increases the incidence of autoimmune disorders (69). Blood tests are mainly done to rule out other underlying causes of weakness that can mimic GBS (67). Blood should be sent for basic metabolic profile, B12, porphyrins, tests for Lyme disease and HIV, and heavy metal levels. Recently, the association between antiganglioside antibodies and GBS (acute motor axonal variant), mainly GM1a, GM1b, GD1a, GalNac-GD1a, and GQ1b has been used to increase diagnostic accuracy (67). This is especially true for GQ1b and the Miller–Fisher variant of GBS.
Electrodiagnostic studies must be interpreted in the context of the clinical findings. Early in Guillain–Barré, nerve conduction and F-wave studies may be normal if recorded from a limb without severe weakness. Delayed or absent F waves, sometimes replaced by axon reflex waves, are the earliest abnormalities (70). Conduction blocks are seen in the later stages of the disease (67). The much less common axonal variants of Guillain–Barré are distinguished from AIDP by electrophysiologic studies. NCSs should show low-amplitude motor CMAPs without marked conduction slowing, conduction block, or dispersion in both AMAN and AMSAN. AMAN is distinguished from AMSAN by preservation of SNAP amplitudes (71). If axons of the motor neurons are involved (AMAN) then motor nerve action potential amplitudes are reduced. If sensory axons are affected as well (AMSAN) a decrease in sensory amplitudes is also noted (67). When recorded from a paralyzed muscle, normal motor NCSs with normal F waves indicate that the weakness does not result from a peripheral cause, and should direct attention toward an alternative diagnosis with localization to the CNS.
Management
Patients with GBS should be admitted and carefully monitored for respiratory failure, swallowing ability, and autonomic function. Specific treatment can hasten recovery and reduce hospital costs, and is recommended for all patients whose weakness threatens loss of ambulation (72). Plasma exchange was the first treatment demonstrated to be effective in GBS. Treated patients demonstrated greater improvement at 4 weeks than untreated controls, and treatment reduced the proportion of patients requiring mechanical ventilation at 4 weeks from 27% to 14% (35,73–75). Every-other-day treatment to a total of five plasma volumes is usually employed. Complications include pneumothorax and sepsis related to central venous access, hypocalcemia, coagulopathy, and hypotension. Intravenous immunoglobulin (IVIG) treatment was shown to be equally effective as plasma exchange in controlled trials (76), and has become the preferred treatment because of greater ease of administration and lesser risk of serious complications. The usual dose is 0.4 g/kg/day infused slowly for 5 days to a total dose of 2 g/kg. Complications include hypersensitivity in patients with immunoglobulin A (IgA) deficiency, acute renal failure, congestive failure, hypercoagulable state, and aseptic meningitis. Treatment is indicated in all but the mildest cases, and is best instituted early in the course of the disease. Treatment after 4 weeks is unlikely to be of benefit. High-dose corticosteroids are of no proven benefit, and in several trials treated patients had more disability than controls (77).
Autonomic instability is common and usually not life threatening, unless it is manifested by asystole or ventricular fibrillation. Hypertension should be treated cautiously, as patients may be unusually sensitive to antihypertensive medications. Paroxysmal hypertension may sometimes be a manifestation of pain, in which case adequately treating the pain can be curative. Neuropathic pain can be treated with antiepileptic medications such as gabapentin (78), antidepressants such as amitriptyline, or analgesics.
The prognosis for recovery is good in all types of GBS, but is poorest for patients with severe AMSAN. Poor recovery is correlated with age, severity of deficits at the nadir of the disease, more rapid progression of deficits (37), and, in some studies, evidence of axonal damage on electrophysiologic studies (79); however, even the most severely afflicted patients may recover fully. About half of patients with otherwise good recovery are left with mild sensory disturbances (62,80,81).
Patients with rapidly progressive weakness should be transferred to the ICU. Respiratory failure is often preceded by progression of weakness to involve the upper extremities, shoulders, neck, and face, but may occur before this weakness is evident. Tachypnea, paradoxical respirations, hypophonia, staccato speech, and a marked decrease in minute ventilation when supine are clinical indications of respiratory failure (17).
MYASTHENIA GRAVIS
The first description of myasthenia gravis is credited to Wilks in 1867 or to Erb in 1879, although Sir Thomas Willis provided a description of the symptoms more than 200 years earlier (82). Freidreich Jolly, who first described the decremental response to repetitive stimulation, called this disorder myasthenia gravis pseudoparalytica (83). Mary Walker, a physician working at St. Alfege’s Hospital in Greenwich, was the first to use acetylcholinesterase inhibitors to treat myasthenic patients (84). The dramatic, albeit temporary, reversal of weakness by physostigmine was called the “Miracle of St. Alfege’s Hospital.” The transient weakness manifested by infants born to myasthenic mothers, and the association of myasthenia with thymic tumors and with thyroiditis, led to the theory that myasthenia was an autoimmune disease (85). This was confirmed in the early 1970s with the demonstration that animals injected with concentrated acetylcholine receptor produced antibodies that caused weakness (86), as well as with the discovery of antibodies to acetylcholine receptors in the sera of myasthenic patients (87). Immunotherapy and improved critical care have lessened overall mortality from 20% to 30% in the 1950s (88) to nearly zero (89).
Myasthenia gravis has a current prevalence of about 20 per 100,000 population. There are about 60,000 patients with myasthenia in the United States (90). Myasthenia gravis occurs throughout life with bimodal peaks in the second and third decade in women and in the fifth and six decades in men (91). As the population ages, the average age of onset has increased and men are now more often affected than women (90). Myasthenic crisis occurs in about 20% of patients with myasthenia; almost 33% of these patients can experience a second crisis (92,93).
Pathophysiology
The neuromuscular junction is composed of a presynaptic motor neuron membrane, the synaptic cleft, and the postsynaptic muscle membrane. In the normal neuromuscular junction, the arrival of an action potential at the nerve terminal leads to the influx of calcium through voltage-gated calcium channels, which triggers release of acetylcholine into the synaptic cleft (94). Acetylcholine diffuses across the synaptic cleft to bind with acetylcholine receptors on the postsynaptic muscle membrane. This binding generates end-plate potentials that depolarize the muscle membrane. When the depolarization exceeds threshold, a muscle action potential is generated, which leads to a cascade of events that results in muscle contraction (95). Neuromuscular transmission fails if the end-plate potential fails to reach the threshold for muscle action potential generation. In LEMS, the end-plate potential is small because antibodies to voltage-gated calcium channels on the presynaptic membrane interfere with acetylcholine release. In myasthenia gravis, the end-plate potential is small because antibodies to the acetylcholine receptor or related sites on the postsynaptic membrane decrease the depolarization caused by normal amounts of acetylcholine.
The acetylcholine receptor is composed of four subunits (96). Antibodies to the acetylcholine receptor can be found in 80% to 90% of patients with generalized myasthenia (97). These antibodies can cause neuromuscular transmission failure by binding to the acetylcholine receptor and altering function, by increasing the rate of degradation of the receptors, and by complement-mediated destruction of the postsynaptic membrane (98). About 30% of patients with generalized myasthenia who are seronegative for acetylcholine receptor antibodies have antibodies to muscle-specific kinase (MuSK) (99). Patients with MuSK antibodies demonstrate more severe disease than patients with antibodies against the acetylcholine receptor (100). MuSK-positive patients have a higher female predominance and higher rates of facial and bulbar weakness with shoulder and neck involvement (101,102,103).
Recent studies have shown that 3% to 50% of patients who are seronegative for AChl receptor and MuSk antibody are positive for a newly found protein called low-density lipoprotein receptor–related protein 4 (Lrp4) which is responsive for retrograde signaling from muscle to presynaptic nerve terminals (101,104,105).
The thymus gland has been implicated in the pathogenesis of myasthenia gravis. Thymic abnormalities are seen in 75% of patients with myasthenia gravis. Of these, 75% have lymphoid follicular hyperplasia and 15% have thymomas (106). Acetylcholine receptors are found on myoid cells of the thymus (107). The majority of myasthenic thymuses contain B cells that can produce antibodies to the acetylcholine receptor (108). Antistriated muscle antibodies in patients with myasthenia gravis correlate highly with thymoma (109).
Patients with myasthenia gravis often have other autoimmune disorders, including thyroiditis, scleroderma, Sjögren syndrome, rheumatoid arthritis, pernicious anemia, polymyositis, and lupus erythematosus (110).
Clinical Presentation
Patients with myasthenia gravis present with fluctuating weakness that improves with rest. The most common complaints are ocular—namely, ptosis and diplopia related to weakness of the levator palpebrae and the extraocular muscles, respectively. Ptosis is the presenting symptom in 50% to 90% of patients, and 90% to 95% of patients complain of diplopia during the disease. Weakness can remain purely ocular in up to 15% of cases, but the majority of patients with myasthenia gravis develop generalized symptoms (83). Dysarthria and dysphagia are common complaints. Neck extensors and flexors are often more involved than other muscles in generalized disease (83). Patients may have to support their chin with their hand to prevent head drop because of neck extensor weakness or to keep their jaw shut because of weakness of the temporalis and masseter muscles. Proximal limb weakness is usually present when the disease is not purely ocular. It is sometimes asymmetric, is occasionally very focal, and can affect distal muscles. In less than 10% of cases, myasthenia presents with proximal weakness without bulbar or ocular weakness.
Myasthenic crisis is diagnosed when weakness progresses to cause dysphagia with loss of airway protection or hypoventilation. Myasthenic crisis can be precipitated by infection, surgical procedures, pregnancy, treatment with corticosteroids, sepsis, cholinergic crisis, and the rapid withdrawal of immune-suppressing medications (93). Pulmonary system infection, including aspiration pneumonia, is the most common identifiable precipitating factor (93). Many medications can also worsen myasthenic symptoms (111), often because they impair neuromuscular transmission. Aminoglycoside antibiotics, quinidine, and procainamide are particularly likely to cause weakness, and should be avoided in patients with myasthenia. Limited experience with telithromycin suggests that it should also be avoided (112). Penicillamine and α-interferon may cause autoimmune myasthenia. Evidence that other medications exacerbate myasthenia gravis is less convincing, but in patients with increasing weakness, all medications must be scrutinized against the long list of potentially offending agents (111).
Myasthenic crisis can involve muscles of inspiration, including the diaphragm and the external intercostals, along with accessory muscles of inspiration, including the scalene and sternocleidomastoid muscles. Respiratory strength can be measured by vital capacity and negative inspiratory force (113). As respiratory weakness leads to ventilatory failure, tidal volumes decline. Patients in myasthenic crisis have an intact respiratory drive, and therefore respond to the decrease in tidal volume with an increase in respiratory rate (114). Oropharyngeal muscle weakness may result in obstruction with upper airway collapse. Secretions can be difficult to control and can also obstruct the airway and lead to aspiration. In the 1950s and 1960s, mortality from myasthenic crisis was as high as 70% to 80% (115,116). In more recent reports, mortality is closer to 4% (85,86), related to improvements in intensive care as well as effective immunomodulatory treatment.
Diagnosis
The history and examination often strongly suggest the diagnosis. A history of fluctuating ptosis, diplopia, fatigable dysarthria, dysphagia, and neck weakness are highly suggestive. Sometimes the presentation may be misleading, as when ocular myasthenia presents with isolated eye muscle weakness or with a pattern of eye movements that mimics internuclear ophthalmoplegia from brainstem lesions, or when patients have proximal limb weakness without eye or bulbar weakness, suggesting a primary disorder of muscle. Conversely, patients with purely ocular findings should be worked up for other causes of ophthalmoplegia, including thyroid eye disease and retrobulbar or cavernous sinus lesions. ALS may present with bulbar symptoms, but patients with ALS do not have ptosis or eye movement weakness, and often have pseudobulbar dysarthria, characterized by slow labored speech and poorly modulated emotional expression, with laughing or crying that is disproportionate to the situation.
Many tests are available to confirm the diagnosis of myasthenia. Definitive diagnosis is important, as most patients will require years of immunomodulatory therapy. The “ice pack test” can be done at bedside to help with diagnosis of MG when improvement in ptosis is seen after applying ice pack to the eyelids (16). Pharmacologic testing with edrophonium (Tensilon), a rapid-acting, short-duration acetylcholinesterase, can result in transient increase in strength. Caution should be used in using edrophonium because anticholinergic side effects, such as bradycardia and bronchospasm, can develop. A test dose of 2 mg of edrophonium, given intravenously, should be administered, followed by increments of 2 mg, for a total dose of 10 mg. Atropine should be available to counteract muscarinic side effects (117). Definite improvement in strength, as demonstrated by improvement in ptosis, dysarthria, or resistance against the examiner’s testing, is supportive of the diagnosis. The test is, therefore, most helpful in patients with clear weakness that can be evaluated in an objective manner (117). In patients with cardiac disease, especially those on β-blockers or drugs that cause atrioventricular block, edrophonium should be avoided, as it can cause asystole (118). Fortunately, there are many alternative tests to confirm the diagnosis of myasthenia.
Serum acetylcholine receptor antibodies are found in 80% to 90% of patients with generalized myasthenia gravis, and have very high diagnostic specificity. Increased titers are also found in patients with thymoma without myasthenia, and rarely in other diseases, including systemic lupus, ALS, liver disease, and inflammatory neuropathies, and in patients with LEMS (119). Antibodies to striated muscle are present in about 30% of patients with myasthenic gravis, and are highly associated with thymoma (120). About 30% of patients with myasthenia gravis who lack antibodies to acetylcholine receptor have antibodies to MuSK. Other antibodies directed against muscle antigens can be found in patients with myasthenia gravis (121).
Patients diagnosed with myasthenia should have additional testing for associated conditions. Computed tomography (CT) or MRI of the chest is required to evaluate for thymoma (89). Tests of thyroid function are important, because about 10% of myasthenics have associated autoimmune thyroid disease, and because proximal weakness and restriction of extraocular movements can be caused by thyroid disease. Antinuclear antibody (ANA) and rheumatoid factor may detect much less commonly associated autoimmune disorders. Tuberculin test and fasting glucose should be obtained prior to treatment with steroids.
Electrodiagnostic Studies
Sensory and motor NCSs are normal except decreased CMAP amplitudes in severely weak muscles (11). The pattern seen in MG with EMG is a decrement in CMAP amplitude with slow repetitive stimulation that improves immediately after exercise and worsens again 2 to 4 minutes after exercise (11).
RNS can be performed to rule out a decrement in the amplitude or area of the fourth or fifth potential compared to the first potential. A train of 5 to 10 stimuli is delivered at a rate of 2 to 3 Hz. If the decrement is more than 10%, it is considered abnormal. The yield can be increased by testing clinically weak muscles and with repeat testing after exercise of the specific muscle. Because typically myasthenia affects proximal more than distal muscles, the test is more likely to be abnormal when testing proximal or facial muscles than when testing hand muscles (122).
The most significant finding on EMG is moment to moment variation in MUAPs amplitude (11).
Single-fiber EMG is abnormal in nearly all patients with generalized myasthenia (123), but it is not widely available because it is time consuming, is technically demanding, and requires expensive needle electrodes.
Anticholinesterase antibodies have to be discontinued 12 hours prior to RNS studies for an accurate result (11).
LEMS is an example of a presynaptic neuromuscular disorder. NCSS can be normal but frequently LEMS is caused by a malignancy and there is an associated paraneoplastic sensory or sensorimotor neuropathy, which results in abnormal values in NCS. Motor NCSS show primarily a low CMAP amplitude increasing by over 100% after 10 to 20 seconds of exercise secondary to increased accumulation of calcium and release of acetylcholine (11).
Clinical Management
The majority of myasthenic patients respond well to anticholinesterase and immunomodulatory treatment and rarely require intensive care. While these treatments can produce dramatic improvement in strength, they do not address the underlying autoimmune pathogenesis of the disease, and tend to become ineffective as the disease progresses.
Treatment
Symptomatic
Pyridostigmine, an anticholinesterase, is used for symptomatic treatment. The dose and frequency of this drug is greatly dependent on the patient’s level of weakness and the potential for side effects. Pyridostigmine does not cross the blood–brain barrier and is the most commonly used oral agent. Sustained-release pyridostigmine is appropriate for use at bedtime to mitigate weakness during the night and in the morning. Multiple daytime doses of sustained-release preparations should be avoided, as accumulation may lead to cholinergic weakness.
Immunosuppressive
Oral prednisone is the most commonly used agent to induce remission in myasthenic patients, and is effective in 80% to 90% of patients. High-dose prednisone—60 to 100 mg/day—may cause transient worsening of myasthenia, and should not be initiated unless patients can be closely observed. Lower doses appear safer, but are not entirely free from this complication. Most patients experience gradual improvement in strength beginning 2 or 3 weeks after initiation of therapy. Once a good response has been achieved, the dose can be tapered over several months to low-dose alternate-day treatment. Doses less than 40 mg every other day are generally well tolerated. Doses of 40 mg or above every other day are associated with many long-term side effects, including diabetes, hypertension, cataracts, avascular necrosis, and increased susceptibility to infection. Azathioprine may reduce steroid dependence. Physicians should consider initiation of non-steroidal immunosuppressants such as azathioprine during the acute phase, to help with tapering steroids.
Rituximab, a monoclonal CD20 antibody (causing depletion of B lymphocytes), used in some retrospective case series for treatment of refractory MG has shown 78% to 100% improvement in clinical symptoms and a reduction in steroids or other immunosuppressant dosage when repeated in cycles over a 6 months period or more (124–126).
Treatment with IVIG can produce rapid improvement in strength, and may be useful to provide short-term improvement while waiting for steroids to become effective.
Plasma exchange has been shown to be effective for the treatment of myasthenic crisis and to prepare patients for surgery (127). Many different protocols are used, but a series of five to six exchanges of 2 to 4 L every other day is most commonly employed. Improvement can usually be seen in 3 to 4 days and can last for several weeks, but is not sustained without the addition of an immunosuppressant agent. Side effects of plasma exchange, which can be life threatening, include hypotension, bradycardia, congestive heart failure, venous thrombosis, infection, and dramatic shifts in electrolytes, including calcium. Controlled randomized trials have demonstrated that IVIG is as effective as plasma exchange in patients with myasthenic crises (128). This trial used 1.2 and 2.0 g/kg of IVIG over 2 to 5 days. Patients who fail to respond to IVIG may subsequently respond to plasma exchange (PLEX) (129). Although patients treated with PLEX may have rapid improvement in their symptoms compared to IVIG, patients receiving IVIG have fewer adverse events. This cannot be extrapolated to all patient groups though. Clinical response rates for IVIG and PLEX are usually similar and range between 55% and 65% (128) (130).
Based on current data, IVIG and PLEX can both be used in treatment of severe MG and MG exacerbation with similar efficacy but it is believed that in patients with MG crisis with respiratory failure requiring ventilator support, PLEX may be superior to IVIG. However IVIG is easier to administer, may have less complications, is readily available and may lower health care costs. Hence, decision on treatment is based on the individual patient, drug availability, and patient’s comorbidities (101).
Intravenous steroids have been used in high doses in myasthenic crisis in patients who do not respond to IVIG or plasma exchange (131), but this may be complicated by transient worsening of myasthenic weakness that may precipitate the need for, or prolong, mechanical ventilation. Other immunosuppressant medications can be useful as steroid-sparing drugs and as sole therapy for myasthenia, but they take weeks or months to produce improvement, and therefore are not appropriate choices to treat myasthenic crisis.
AAN guidelines published in 2000 considers thymectomy as an option for treatment of MG based on a 28 case series showing higher rates of medication-free remission and improvement in patients who underwent thymectomy (132).
Thymectomy is indicated in patients with thymoma, and is also accepted therapy for myasthenia gravis without thymoma, particularly in younger patients without thymic involution. However, the absence of class I evidence and the shortcomings of existing case-control or cohort trials have led to a call for prospective randomized controlled clinical trials (132).
Airway Management
Myasthenia crisis is defined by development of respiratory failure and a need for ventilator support by noninvasive or invasive mechanical ventilation. It is usually seen in 15% to 25% of patients with MG and is mostly seen in patients with known diagnosis of the disease. It can be triggered by a variety of stressors, including surgery, infections, changes in medication, etc. (66,101).
Myasthenic weakness can progress very rapidly; therefore, patients with worsening myasthenic symptoms should be hospitalized for definitive management. Patients with progressive bulbar weakness who have difficulty controlling secretions, an ineffective cough, or vital capacities less than 20 to 25 mL/kg should be admitted to the ICU (133,134). Unlike patients with GBS who would not benefit from noninvasive ventilation, patients with MG benefit greatly from noninvasive modes of ventilation in particular BiPAP which can prevent patients from reaching the point of intubation and mechanical ventilation. It is imperative to initiate supportive care with BiPAP as soon as the patient is admitted to ICU since respiratory failure rates, pulmonary complications, and pneumonia rates may increase if there is delay in treatment (67). When the vital capacity reaches 15 mL/kg, endotracheal intubation should be strongly considered. Elective intubation can help patients avoid abrupt respiratory failure and mucous plugging (135). Intermittent mandatory ventilation with pressure support is used to reduce alveolar collapse or atelectasis. Positive end-expiratory pressure can reduce complications related to atelectasis (136). If mechanical ventilation is required for more than 2 weeks, tracheostomy should be considered. The most common complications of myasthenic crisis are fever, pneumonia, and atelectasis (93). Aggressive respiratory treatment with suctioning, bronchodilator treatments, sighs, chest physiotherapy, and intermittent positive pressure can reduce the complications of mechanical ventilation and shorten the period that patients are in the intensive care unit (137). Weaning trials should be attempted with patients who show clear improvement of respiratory muscle strength, with the goal to eliminate mandatory ventilation and then reduce the amount of pressure support. If oral and respiratory secretions are concerning, the dose of pyridostigmine can be either decreased or the medication is completely stopped after intubation, and should be reintroduced prior to weaning trials. In patients on noninvasive ventilation, pyridostigmine should not be discontinued if possible.
Perioperative Care
Myasthenic crisis is common in patients undergoing surgical procedures. The main risk factors for postoperative complications include severe bulbar weakness, chronic myasthenia, respiratory illness, and decreased vital capacity preoperatively (138). Whenever possible, preoperative treatment to improve strength should be undertaken. Plasma exchange or IVIG can be performed preoperatively in patients with bulbar weakness or generalized myasthenia. A response is often seen within 3 to 6 days, although preoperative IVIG has shown a variable time to maximal response (1,139). Preoperative pulmonary function testing can be performed to assess respiratory function.
CRITICAL ILLNESS POLYNEUROPATHY AND MYOPATHY
Although limb and respiratory muscle weakness in critically ill patients was noted in 1892 by Osler (140), it was initially attributed to catabolic myopathy and diaphragmatic fatigue. Recognition that critically ill patients frequently develop new neuromuscular dysfunction was not widespread until the final decades of the 20th century. In 1984, Bolton et al. (1) described five patients who failed to wean from the ventilator after treatment of sepsis, and were found to have neuromuscular weakness related to an axonal sensorimotor polyneuropathy. They called this critical illness polyneuropathy (CIP). In 1992, Sagredo et al. (141) attributed prolonged paralysis, seen in patients in whom vecuronium had been weaned, to persistent plasma levels of vecuronium metabolites in those patients with impaired renal function. Hirano et al. (142) described severe weakness in two critically ill asthmatic patients treated with steroids and nondepolarizing blocking agents. Muscle biopsy demonstrated depletion of thick myosin filaments. They called this acute quadriplegic myopathy. Other terms for the same or similar illness have included hydrocortisone myopathy, necrotizing myopathy, thick filament myopathy, postparalysis syndrome, and acute myopathy of intensive care; we will refer to it by the most common designation: critical illness myopathy (CIM). The term critical illness neuromyopathy (CINM) is used to refer to patients in whom both neuropathy and myopathy coexist, or when it is uncertain which condition is causing the patient’s weakness. The term CINM could also be applied to patients with persistent neuromuscular blockade; however, electrophysiologic studies should exclude this as a contributing factor in most patients.
Epidemiology
The incidence of CINM is estimated to be as high as 70% of mechanically ventilated ICU patients with sepsis and multiorgan failure. Witt et al. (143) found, in a prospective study, that 70% of 43 ICU patients with sepsis and failure of more than two organs developed electrophysiologic evidence of peripheral neuropathy, and half of these patients had clinically evident neuropathy with difficulty being weaned from the ventilator. De Jonghe et al. (144) found that 25.3% of ICU patients intubated for 7 or more days developed neuromuscular weakness. All had sensorimotor axonopathy, and all who were biopsied also had myopathy. Bednarik et al. (145) prospectively studied 61 ICU patients with evidence of multiorgan failure; 27% had clinical and 54% electrophysiologic evidence for CINM. Bercker et al. (146) found that 60% of 50 consecutive patients with acute respiratory distress syndrome (ARDS) studied retrospectively developed CINM.
Pathophysiology
Critically ill ICU patients can develop severe weakness of limb and respiratory muscles from an axonal polyneuropathy (CIP) or a myopathy (CIM). They often coexist, thus the term critical illness neuromyopathy or a more recently coined term, ICU-acquired weakness is used to describe the entity of clinically detected weakness with no plausible cause other than critical illness (147). They may occur in approximately 50% of patients that have severe sepsis, multiorgan failure, or are mechanically ventilated for 3 or more days and can be a common cause of failure to wean from mechanical ventilation in these patients (148).
Critical Illness Polyneuropathy
Sepsis and multiorgan failure are the major risk factors for CIP (145,149,150,151); other risk factors include duration of mechanical ventilation, hyperglycemia, hyperosmolality, and hypoalbuminemia (143). The PNS is one of the organ systems damaged in systemic inflammatory response syndrome (SIRS) which can cause cytokine release. There is speculation that endothelial cell activation, increased permeability of the microcirculation, nerve ischemia and mitochondrial injury play a role in pathogenesis. A high catabolic state due to systemic inflammation and oxidative stress is also associated with skeletal muscle wasting, and diaphragmatic weakness was seen as early as 6 days in mechanically ventilated patients (152). Decreased nutrient supply, increased demand, decreased clearance of toxic substances, and tissue edema can ultimately terminate in neuronal injury and axonal degeneration. Pathologically, there is axonal degeneration without inflammation, and there is an increase in adhesion molecule expression in nerve (149).
Critical Illness Myopathy
Risk factors for CIM overlap with those for CIP; however, there is more emphasis on primary pulmonary disease (asthma and ARDS) and on the use of neuromuscular blocking agents and corticosteroids (144,153). High corticosteroid levels may increase expression of ubiquitin, leading to increased proteolytic activity (154). Muscle inactivity in itself can act as a potent stimulus of the ubiquitin-proteasome pathway of proteolysis and can cause muscle wasting. Denervation from coexisting CIP may make the muscle more susceptible. Muscle biopsy shows loss of thick (myosin) filaments; there may also be fiber-type atrophy and/or necrosis (142,155). Whether the different pathologies represent a spectrum of severity or different entities remain unsettled.
Most patients on ventilators are given neuromuscular blocking agents. Normally, the effects of these agents wear off within hours of discontinuation; however, it is not unusual for residual blockade to last 1 to 2 days (141). Early reports of more prolonged weakness after neuromuscular blockade did not consider the effects of CIP and CIM. In a recent review, Murray et al. (153) concluded that nondepolarizing neuromuscular blocking agents may potentiate muscle weakness by a number of mechanisms, including upregulation of acetylcholine receptors with fetal-type receptors that are less responsive to acetylcholine, presynaptic inhibition of exocytosis of acetylcholine, and potentiation of muscle damage by corticosteroids and by sepsis-induced ischemia.
Clinical Presentation
CIP is often preceded by SIRS, severe sepsis, or multiorgan failure. CIM may have similar antecedents, but is more likely in the setting of ventilatory failure from asthma (142,155) or ARDS (146). The use of neuromuscular blocking agents (156) and corticosteroids (144 appears to predispose to CIM, although CIM has been reported without either one (157).
CIP or CIM are most often diagnosed when sedation is weaned or sepsis and encephalopathy improve in a mechanically ventilated patient, revealing a profound generalized weakness. Neurologic examination can reveal a normal mental status and cranial nerves with generalized flaccid weakness which is sometimes worse distally and failure to wean from the ventilator. Hyporeflexia, areflexia, and muscle atrophy can be seen in CIP where reflexes are usually preserved or hypoactive in CIM. Distal sensory loss may be present in majority of patients with CIP, but is not always reported or amenable to testing (158). Sensation is normal in CIM if neuropathy does not coexist, but often encephalopathy and poor communication make this difficult to assess. Electrodiagnostic studies can therefore be key to diagnosis.
Diagnosis
The diagnosis is likely in patients with risk factors, particularly sepsis, SIRS, multiorgan failure, prolonged artificial ventilation, hyperglycemia, and exposure to neuromuscular blocking agents or corticosteroids (159). Alternative causes for weakness in the ICU must be considered and, if possible, excluded. These include medications that cause myopathy, neuromuscular blockade, or neuropathy; electrolyte disorders such as hypokalemia or hypophosphatemia; undiagnosed neuromuscular disorders such as myasthenia gravis, GBS, or ALS; and a variety of CNS disorders, including ischemic myelopathy, anterior horn cell loss from hypoxia (160), CPM that most often follows rapid correction of hyponatremia, and hemispheric stroke from hypotension, emboli, or hemorrhage (161). West Nile encephalitis (WNE) should be considered in patients who present with fever and encephalopathy, and in those who develop neuromuscular weakness, since WNE can affect anterior horn cells and, less often, peripheral nerves (162). Depending on clinical suspicions, imaging of the brain and spinal cord may be appropriate. If clinical findings suggest neuromuscular weakness rather than a CNS disorder, it may be more efficient to confirm with electrodiagnostic studies than to pursue studies for CNS disorders.
Laboratory studies can help to exclude mimicking disorders. They should include a basic metabolic profile to assess blood sugar and electrolytes. Elevation of creatine kinase (CK) may suggest myopathy, and very high CK could suggest rhabdomyolysis. Normal CK does not exclude CIM, and mild elevations in CK can be seen with acute denervation. Tests for myasthenia (see Myasthenia Gravis, above) are indicated when there is a clinical suspicion. Spinal fluid findings are not often reported in CIP. Lumbar puncture is indicated principally if there is clinical suspicion of infection.
Electrophysiologic testing and muscle biopsy can help confirm the clinical suspicion of CIM or CIP and help to distinguish them from each other as well as other disorders of nerve, neuromuscular junction, and muscle (for a more detailed synopsis, see Dhand (163)). NCSs may be difficult to perform and interpret due to multiple artifacts, extremities may be cool and difficult to warm and they may be confounded by tissue edema. EMG may not be useful when patients are not awake or unable to contract muscles to command. Nonetheless, they are usually diagnostic (159).
NCSs in CIP show a very decreased amplitude of CMAP and SNAPs without significant slowing of conduction velocities or increased CMAP durations. The abnormalities are usually length dependent. NCS findings are generally present by day 14 of critical illness, but low amplitudes may be noted within a week and as early as 72 hours after onset of sepsis (159). In patients with CIM, the CMAP is also of low amplitude, but unlike CIP, CMAP duration may be prolonged due to slowing of muscle fiber conduction velocity and reduced excitability of the sarcolemmal membrane (164,165). These changes occur within 2 weeks of critical illness (165). SNAPs should be normal in CIM, unless there is coexistent CIP. It should be kept in mind that pre-existing neuropathy may account for some of the deficits found on electrophysiologic testing, as, for example, in patients with diabetic neuropathy. Needle EMG studies may show fibrillation potentials and positive sharp waves in a multifocal pattern generally after 2 weeks but have been reported as early as 7 days after initiation of mechanical ventilation (150). Voluntary activity may be difficult to study in poorly cooperative patients and reduced response on direct muscle stimulation can be suggestive of CIM. When the patient can provide good effort, there is reduced recruitment in CIP and small polyphasic motor unit potentials in myopathy, helping to differentiate CIP from CIM.
Guillain–Barré polyneuropathy can follow surgery or trauma, and could therefore occur in settings similar to the critical illness neuromyopathies. Electrophysiologic findings diagnostic of demyelinating neuropathy—not found in CIP or CIM—would suggest this possibility. In theory, an axonal form of Guillain–Barré would be electrophysiologically indistinguishable from CIP. This highly unlikely occurrence should be considered in patients who lack the usual risk factors for CIP.
Additional electrodiagnostic studies that may be useful in diagnosis include phrenic nerve stimulation, diaphragmatic EMG, and direct muscle stimulation. While these studies do not require specialized equipment, many electromyographers do not have sufficient experience with them to be confident in their performance or interpretation. Phrenic nerve stimulation in the neck with surface recording over the diaphragm shows a normal response in patients with pulmonary causes of respiratory failure, but a low amplitude or absent response in patients with neuromuscular respiratory weakness. Needle EMG of the diaphragm can be done in patients without severe chronic obstructive pulmonary disease (COPD), ileus, or coagulopathy, and may diagnose denervation or myopathy affecting the diaphragm (166). CIP and CIM may be distinguished by comparing the muscle response to direct muscle stimulation with the response to nerve stimulation. If the response to muscle stimulation is larger than to nerve stimulation, CIP is likely; when both are small, CIM is likely (167,168).
Muscle biopsy in CIP may show signs of acute denervation with atrophy of types 1 and 2 fibers and grouped atrophy may be seen in severe cases. Nerve biopsy in CIP shows signs of axonal neuropathy. Muscle biopsy in CIM shows a selective loss of thick (myosin) filaments with degree of muscle necrosis directly proportional to disease severity. Although muscle and nerve biopsies may be diagnostic, they often only change management if they demonstrate an alternative diagnosis that may be amenable to treatment, such as a demyelinating or vasculitic neuropathy, or an inflammatory myopathy. In patients whose neuromuscular deficits are clearly related to the usual risk factors for CINM, treatable conditions are not sufficiently likely to warrant biopsy (169).
Management
Treatment of hyperglycemia, early physical rehabilitation and minimization of sedation have been demonstrated to improve functional outcomes, reduce delirium and increase ventilator-free days in critically ill patients. These interventions are likely beneficial but no specific therapy for CINM exists (170). Of the factors that may contribute to the development of CINM, many—like the response to sepsis and the incidence of multiorgan failure—are related to the severity of the presenting critical illness, and are not easily modifiable. It remains to be demonstrated whether the reduced use of corticosteroids or neuromuscular blocking agents will result in a decreased incidence of CIM. Intensive insulin therapy to maintain strict normoglycemia was shown to reduce the incidence of CIP by 49% in a single-center study (171), but this practice has been shown to increase mortality in patients who are critically ill (172). Therefore, control of sustained hyperglycemia is reasonable, but aggressive insulin protocols and any hypoglycemic episodes should be avoided (172–174). Neurotoxic medications should also be avoided. Patients with neuropathy are more prone to pressure palsies, and thus, great care must be taken in positioning and support to avoid this complication. In patients that are unable to participate in strengthening exercises, early physical therapy should be directed toward prevention of contractures and improvement of functional outcome (170).
Prognosis
Long-term prognosis in CINM is still not fully studied but the initial mortality is high (152). Patients with CINM who survive the acute illness will slowly recover over weeks to months. A subset of patient with prolonged severe illness and CINM will never fully recover. Patients with CINM who fail to show clinical improvement in strength should be evaluated with periodical electrophysiologic testing. Patients with evidence of severe axonal loss, small or absent responses to nerve stimulation, and abundant positive waves and fibrillations on needle EMG are likely to have residual deficits (2,143,151,165,175–177). CIM seems to have a better functional recovery than CINM at 1-year follow-up (178).
SUMMARY
Neuromuscular diseases may necessitate admission for intensive care to provide airway protection and respiratory support. Disorders such as GBS and myasthenia gravis can lead to life-threatening precipitous weakness. Management must also address autonomic dysfunction, sensory loss, pain, and psychological distress. Neuromuscular disorders of autoimmune pathogenesis often respond to specific treatments, and a favorable outcome can be expected for the majority of such patients. Critical illness neuromyopathies have become the most common cause of weakness that develop in the intensive care setting, requiring critical care physicians to be familiar with their presentation, diagnosis, and management. Although no specific treatment is currently available for CINM, most patients improve with supportive care and intensive physiotherapy. A minority, however, are left with irreversible weakness.
Key Points
- The pattern of neuromuscular disorders differs between individuals and carries importance in differentiating one disease process from another.
- Care of the patient suffering from acute neuromuscular disease often begins in the prehospital setting.
- Neuromuscular emergencies can follow an acute condition or the worsening of a chronic neuromuscular illness.
- Respiratory failure is the most important complication observed in patients suffering from an acute neuromuscular disorder.
- NIPPV is gaining importance in the management of respiratory symptoms in myasthenia gravis, particularly in the initial stage of symptoms.
- Early diagnosis can be achieved with an accurate clinical history, physical examination, laboratory and radiologic testing.
- IVIG and plasma exchange are the cornerstones of treating GBS.
- Critical illness neuropathy and myopathy are increasingly being diagnosed as a sequela of prolonged hospitalization in critically ill patients.
- Electrophysiologic studies can provide valuable information for diagnosing the underlying disorder.
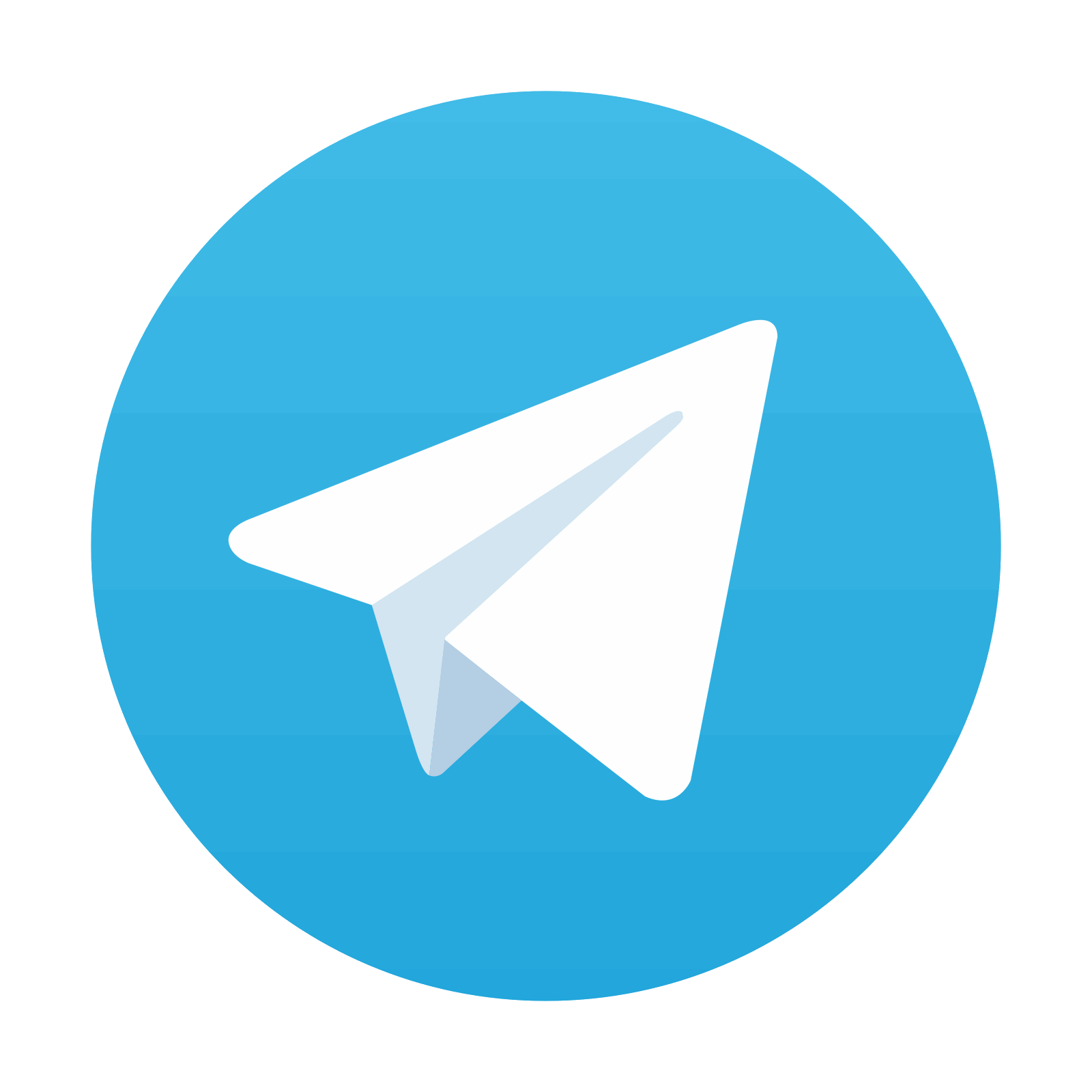
Stay updated, free articles. Join our Telegram channel

Full access? Get Clinical Tree
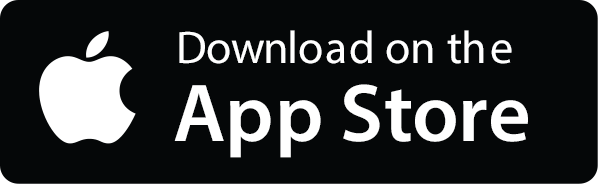
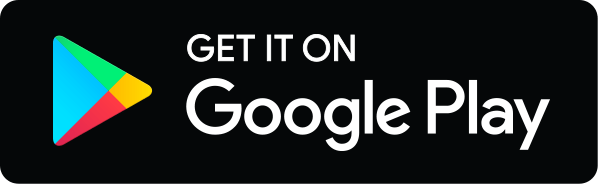