Neuromuscular Anatomy and Physiology
Jodi H. Kirsch
Jeffrey Kirsch
▪ INTRODUCTION
One of the key activities in conducting a general anesthetic for surgery can be to provide muscle relaxation (paralysis) in order to facilitate placement of an endotracheal tube in the trachea or to provide optimum operating conditions for the surgeon with quiet, soft muscles. This is accomplished with medications that interfere with normal functioning of the junction between nerves and muscles. Knowledge of the anatomy and physiology of the neuromuscular system will help anesthesia technicians understand the mechanism of how drugs affect the neuromuscular junction (NMJ) and how nerve stimulators can be used to monitor the condition of the neuromuscular system.
▪ ANATOMY OF THE NEUROMUSCULAR SYSTEM
The neuromuscular system, as implied by the name, is composed of nerves and muscles. These nerves are considered peripheral nerves. They exit from the spinal cord and then act upon the muscles of the body. There are three different types of muscle: cardiac muscle in the heart, smooth muscle, and skeletal muscle. Although similar, each of the different types of muscles has slightly different properties. Whereas skeletal muscle can be controlled at the desire of an individual, both cardiac and smooth muscle contraction and relaxation occur independent of direct individual control. Smooth muscle is an involuntary type of muscle that is controlled by the autonomic nervous system (see Chapter 14). Examples of smooth muscle include the walls of blood vessels, lymphatic vessels, bronchioles in the lungs, urinary bladder, and the reproductive tract of males and females. Contraction or relaxation of these muscles occurs in response to activity/need, rather than by direct control of the individual. Cardiac muscle is also a type of involuntary muscle.
While our body is composed of smooth muscle, cardiac muscle, and skeletal muscle, the majority of the body is skeletal muscle. Skeletal muscle is a voluntarily controlled, striated (under the microscope)-appearing tissue that enables our body to move. Skeletal muscles attach to bones by way of tendons. Skeletal muscle is composed of many muscle fibers, which are held together by protein and fibrous tissue (Fig. 16.1). These muscle fibers in turn are composed of multiple myofibrils (the actual individual muscle cells). Within each myofibril are abundant mitochondria (the energy-producing organelle in all cells), smooth endoplasmic reticulum (the cell organelle that produces lipids, metabolizes carbohydrates, and regulates calcium levels in the cell), and most importantly multiple nuclei. Multiple nuclei are imperative for skeletal muscle function due to the high activity level required of these muscles.
The main contractile unit in skeletal muscle cells is called the sarcomere. There are many sarcomeres within one myofibril. When a sarcomere is further examined under an electron microscope, one observes repeating sections of light and dark areas. The light areas are the thin filaments. Thin filaments are composed of the protein, actin. The dark areas of the sarcomere are the thick filaments and are composed of the protein myosin. During contraction the myosin on the thick filaments binds to actin on the thin filaments. The binding of actin to myosin is a process that requires calcium and energy. The energy for the reaction is supplied by adenosine triphosphate. Overall, this process results in shortening of the sarcomere, which is anatomically
recognized as muscle contraction. The molecular basis of muscle contraction is explained by the “sliding filament theory.” This theory states that in the presence of calcium and energy, actin and myosin slide past each other and the sarcomere shortens. The protein filaments overlap but do not shorten. The overlap is what causes the sarcomere to shorten.
recognized as muscle contraction. The molecular basis of muscle contraction is explained by the “sliding filament theory.” This theory states that in the presence of calcium and energy, actin and myosin slide past each other and the sarcomere shortens. The protein filaments overlap but do not shorten. The overlap is what causes the sarcomere to shorten.
▪ THE NEUROMUSCULAR JUNCTION
The NMJ is composed of the terminal portion of a motor neuron axon and the motor end plate of a muscle fiber. The function of the NMJ is to allow the muscle to contract based on messages originating in the brain. Once the motor cortex of the brain sends the message, the message is delivered by way of action potentials (electrical signals) along the axons of motor neurons. When the action potential is sent down the axon, it eventually reaches the NMJ (Fig. 16.2).
The junction across which one nerve sends a signal to another nerve, muscle, or gland is called a synapse. The terminal portion of the nerve where the signal originated is the “presynaptic” nerve. Once the nerve action potential arrives at the terminal portion of the presynaptic nerve, it causes acetylcholine (ACh) to be released from the axon terminal into an area between the axon and the motor end plate, called the synaptic cleft. The ACh then travels across this area to attach to nicotinic ACh receptors on the motor end plate of the muscle (Fig.16.3).
Binding of ACh to these receptors causes sodium and potassium channels to open in the muscle cell membrane. Sodium and potassium travel opposite each other; sodium travels into the cell, and potassium trickles outward. Because sodium rushes into the motor cell much more quickly than potassium leaves the cell, the cell becomes more positively charged and depolarizes (a change in the charge of the interior of the cell membrane compared to the outside). Depolarization of the muscle cell then causes the release of calcium from sarcoplasmic reticulum in the muscle cell. Excess calcium in the muscle cell binds to the thin filament in the sarcomere, triggering a cascade of events resulting in muscle contraction.
But how does our brain send a message to the many muscles of the body to contract or relax? Let us use the example of an anesthesia technician carrying an arterial line setup to an operating room. When the anesthesia technician wants to lift his or her arm, the bicep muscle must contract. A message is sent from the brain to tell the biceps muscle that it must shorten to accommodate the weight of the arterial line setup. The message originates in upper motor neurons in the motor area of the brain. The upper motor neuron crosses to the opposite side at the level of the brainstem and then synapses with a lower motor neuron in the anterior portion of the spinal cord, just before exiting the spinal canal. Thus, movement of the right side of your body comes from a message that originates in the left side of your brain. Because the movement of the arm happens almost instantaneously, the signal must travel very quickly from the brain to the muscles. This is why motor neurons are myelinated nerve fibers, to speed conduction (see Chapter 15). Myelin is an insulating material that covers nerve axons to increase the speed of signal transmission.
▪ DISEASES THAT ALTER NEUROMUSCULAR FUNCTION
Normal function of the complex transmission of information from the brain to muscle through the NMJ is essential to each individual for normal movement. Unfortunately, there are several disease processes that interrupt this normal function with defects that affect each of the steps along the way. Most proximal to the NMJ would be a defect in the motor cortex. For example, a stroke (an obstruction in a blood vessel or a ruptured blood vessel) in the motor cortex can prevent movement in the extremity on the opposite side of the body. This type of injury would present with upper motor neuron signs and symptoms (see below). The motor signals that originate in the cortex of the brain are sent out to the body through motor neurons. Disease of
the upper motor neurons themselves can affect transmission of the signal. Two of the most common central nervous system diseases affecting motor neurons include multiple sclerosis and Parkinson’s disease. A spinal cord injury could also interfere with upper motor neurons as they pass through the spinal cord. Because the nerves have already crossed over to the opposite side of the body at the level of the brainstem, a spinal cord injury will cause upper motor neuron signs and symptoms on the same side as the injury. Upper motor nerve signs and symptoms evolve over time (weeks to months) and include increased intensity of reflexes (e.g., knee jerk reflex) and muscle spasticity.
the upper motor neurons themselves can affect transmission of the signal. Two of the most common central nervous system diseases affecting motor neurons include multiple sclerosis and Parkinson’s disease. A spinal cord injury could also interfere with upper motor neurons as they pass through the spinal cord. Because the nerves have already crossed over to the opposite side of the body at the level of the brainstem, a spinal cord injury will cause upper motor neuron signs and symptoms on the same side as the injury. Upper motor nerve signs and symptoms evolve over time (weeks to months) and include increased intensity of reflexes (e.g., knee jerk reflex) and muscle spasticity.
![]() ▪ FIGURE 16.3 The neuromuscular junction with release of presynaptic acetylcholine binding to postsynaptic nicotinic receptors. |
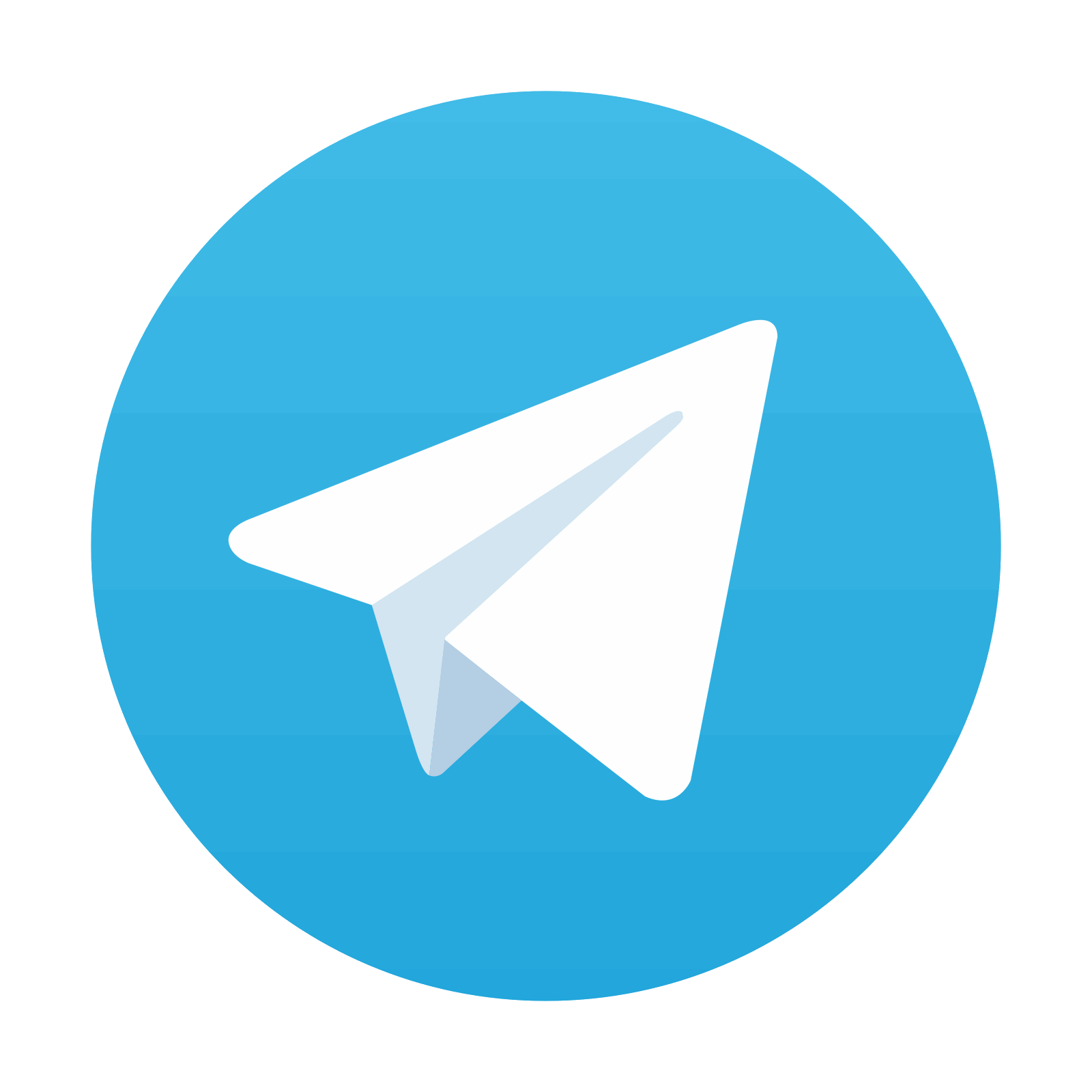
Stay updated, free articles. Join our Telegram channel

Full access? Get Clinical Tree
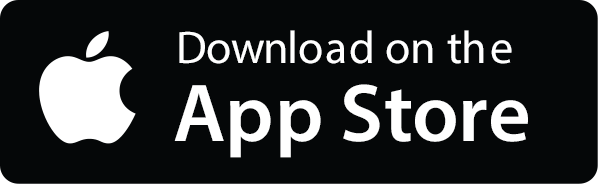
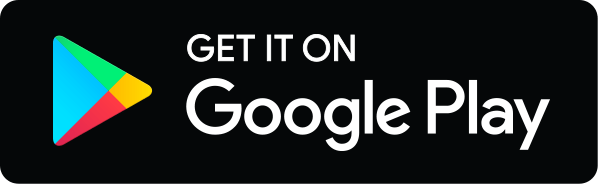