Neurostimulation techniques have been used in the management of pain for close to 50 years. Transcutaneous electrical nerve stimulation (TENS) represents one of the most widely utilized external neuromodulation devices. Over the past several decades, more complex forms of neurostimulation devices and techniques have been developed and implemented in pain management practice for permanent human implantation. These include peripheral nerve stimulation (PNS), peripheral nerve field stimulation (PNFS), spinal cord stimulation (SCS), and intracranial stimulation (ICS). More patients suffering from intractable pain elect to undergo neurostimulation device implantation after failure of conservative management techniques for pain control. The advantages from a patient’s perspective include an essentially side-effect free device under direct patient control that can be dynamically self-adjusted to his or her level of pain and activity.
For physicians, electrical implantable therapies have gained popularity because of safety, long-term efficacy, and cost savings.
The inspiration for development of these technologies came from the landmark “gate control theory” introduced by Melzack and Wall in 1965.1 Although this model fails to explain certain phenomena seen in painful conditions and cannot account for all of the observed effects of neurostimulation, the gate control theory remains the primary paradigm used to describe how neurostimulation acts to modify pain transmission. The gate control theory is based on the presence of interneurons in the dorsal horn of the spinal cord that receive afferent signals from peripheral C fibers (which convey painful stimuli) as well as nonnociceptive sensory fibers. When pain signals reach these dorsal horn interneurons, a “gate” is activated, allowing painful impulses to propagate along ascending fibers to the brain and resulting in conscious awareness of pain. Wall and Melzack proposed that the “gate” could be closed to transmission of painful impulses by means of selective activation of nonnociceptive sensory fibers. With this hypothesis, the notion of using neurostimulatory devices to preferentially activate nonnociceptive sensory fibers as a means of diminishing pain was born.
The application of neurostimulation has expanded dramatically since C. Norman Shealy implanted the first spinal cord stimulator in 1967. Current indications for the use of these devices (worldwide) include failed back surgery syndrome (FBSS), complex regional pain syndrome (CRPS), peripheral vascular disease (PVD) with critical limb ischemia, refractory angina pectoris, deafferentation syndromes, isolated peripheral nerve injuries, spinal cord injury related pain, interstitial cystitis, and trigeminal neuralgia. The range of painful conditions amenable to treatment with these techniques continues to broaden with more recent studies suggesting the potential for these devices to provide relief of chronic visceral pain, headaches, and poststroke pain. In addition to treatment of pain, neurostimulation may also be useful as a means to monitor evoked potentials during thoracoabdominal aneurysm repair2 and in the treatment of movement disorders (dystonia, Parkinson disease, and essential tremor), Tourette syndrome, major depression, and obsessive-compulsive disorder.
The use of electrical stimulation of peripheral nerves for pain relief was first reported more than 40 years ago by Wall and Sweet based on the aforementioned gate control theory.3 The basic goal is to place a lead (an assembly of stimulating contacts, wires and insulators) that can depolarize the axons of a peripheral nerve in order to render comfortable paresthesias within the sensory distribution of that nerve and override pain and dysesthesias. The lead may be placed on the nerve or in the subcutaneous tissues along the course of the target nerve.
The popularity of PNS began in the 1990s with the introduction of occipital nerve stimulation for headache secondary to occipital neuralgia by Reed and Wiener.4 Since then, epicranial PNS of the various sensory scalp nerves has been explored by a number of authors as treatments for chronic migraine, chronic cluster headache, and neuralgias of the supraorbital and occipital nerves (Fig. 90-1).5–8 Similarly, PNS for the extremities and trunk has been reported primarily in anecdotal form for the treatment of a variety of neuralgias9–11 (Fig. 90-2). Tables 90-1 and 90-2 summarize the reports of PNS for the management of multiple refractory chronic pain conditions. The Food and Drug Administration (FDA) has approved PNS only for neuropathic pain of the extremities and trunk.12
Selected Cases of Epicranial Peripheral Nerve Stimulation
Headache Condition | Number of Patients | Outcome |
Chronic migraine | 7 25 8 | 100% of patients with marked reduction in headache frequency and severity with normal function using SONS and ONS.13 ONS resulted in 90% average improvement in migraine disability scores at 18-month follow-up.14 All patients reported more than 50%-75% reduction in headache frequency and intensity with ONS or SONS.15 |
Chronic cluster | 14 8 8
1 | ONS patients with a mean follow-up of 17 months resulted in cluster reduction for 3 (90%), 3 (50%), 4 (30%), and 4 patients with no change.16 ONS with median follow-up 20 months for attack frequency, 2 patients with 90%, 3 with average of 50%, and the rest with little to no response.17 Therapeutic ONS rendered two patients pain free, 2 with a 90% attack frequency reduction, 2 with 40% reduction in attack frequency, and 2 no response over mean 15- month follow-up.18 SONS rendered remission of symptoms after 2 months and lasted over 14 months at last follow-up.19 |
Hemicrania continua | 1 | Left ONS resulted in the patient being pain free at 3 months follow-up.20 |
Selected Cases of Peripheral Nerve Stimulation and Peripheral Nerve Field Stimulation for the Trunk
Medical Condition | Number of Patients | Clinical Outcome |
Low back pain | 13 6 | PNFS resulted in a 50% pain intensity reduction at 7-month follow-up.21 PNFS with a 50% reduction in pain level.22 |
Neck pain | 1 | PNFS provided 100% pain alleviation at 9-month follow-up for midaxial symptoms.23 |
Thoracic pain | 1 2 | PNFS for postthoracotomy related pain, 90% improvement.24 PNFS in postherpetic neuralgia of the chest wall gave reduction in medication and marked improvement.25 |
Inguinal pain | 3 | PNS in all patients gave greater than 75% relief at follow-up out to 12 months.26 |
Abdominal pain | 2 | PNFS applied in one patient with chronic pancreatitis and one with abdominal wall pain following liver transplantation, follow-up at 9 months with marked pain score reduction.27 |
Shoulder pain | 1 | PNFS allowed for 95% pain reduction from traumatic scapular fracture, engaged in physical therapy, and discontinued all oral medications.29 |
In contrast to PNS, PNFS is applied to the region of the body where pain is perceived and not a specific nerve or its sensory distribution. PNFS targets a very specific pain distribution and usually cannot be relied upon to spread much beyond the active portions of the length and width of the lead.30 Some authors have reported that PNFS can be expanded with the addition of a second lead in order to create interlead stimulation (cross talk) to cover a pain topographical area up to 377 square centimeters, while others have not found such cross talk influential.31,32 However, PNS produces paresthesias beyond the active electrode dimensions and often follows in the sensory distribution of the stimulated peripheral nerve.33 Because paresthesias must be felt in the area of pain in order to render relief, PNFS is believed to stimulate distal branches of peripheral nerves that are too small to be specifically distinguished. As with PNS, PNFS involves placing the leads in the subcutaneous space in order to create deep and soothing paresthesia sensations. PNFS is most frequently applied to painful regions of the axial spine that are difficult to treat with SCS, in particular the low back (Fig. 90-3).30
FIGURE 90-3.
Axial back pain related to hardware treated successfully with peripheral nerve field stimulation (PNFS) with overlapping dual 4 contact leads. N.B. Anterograde spinal cord stimulator leads (T10 level) alleviated bilateral radiating leg pain but failed to deliver adequate paresthesia coverage at the L3-5 level.

The mechanism(s) for PNS and PNFS are complex and incompletely understood. PNS is thought to activate low threshold A-β (beta) fibers that inhibit nociceptive A-Δ (delta) and C-fibers at the level of the dorsal horn.34 A-β fibers may also propagate signals via medial lemniscal tracts to the level of the thalamus in the ventroposteromedial nucleus.35 At this location, PNS may modulate the activity of ascending spinothalamic tracts at the third order neuron level. Another potential mechanism may include impulse interruptions by collision that may be important for inhibiting excessive peripheral nerve activity, for example in a case of neuroma.36 PNFS has been thought to have similar mechanisms to PNS but also include altering local blood flow, local endorphins, as well as blockade of cell membrane depolarization.30 Interruption of nociceptive traffic by PNS along afferents that traverse the calvaria at suture lines may be an underlying mechanism of how epicranial stimulation alleviates primary headache syndromes.37 Thus, there is little preclinical work to guide a quickly growing clinical interest in these techniques.
As would be expected, most studies are anecdotal series reporting 60-70% of patients having analgesic and/or functional improvement with PNS.38 A recent prospective observational trial following 100 patients over 1 year consisted of: 40-craniofacial pain, 8-thoracic, 44-lumbosacral, 3-abdominal, and 5 groin/pelvis.39 The average pain reduction was just over 40% in pain intensity, with secondary outcomes including 72% of patients reducing analgesic consumption, and chronic low back pain patients having significant improvement in the Oswestry Disability Index (ODI). In patients with chronic migraines, occipital nerve stimulation (ONS) reduced the headache days per month by 50% or headache intensity by 3 points or more at 3-month follow-up in 39% of drug-resistant patients.40 The study was prospective, multicenter, randomized, blinded, placebo-controlled, and contained optimal inclusion/exclusion criteria. It concluded that response to occipital nerve block failed to predict the outcome from PNS. Ultimately, 61% of chronic migraine patients did not achieve a positive response in this study, suggesting the need for better selection criteria given the costs of this technology.
PNS and PNFS are frequently applied to patients with favorable psychological profiles who have conditions that are difficult to stimulate using conventional SCS techniques. Unanswered questions about these techniques remain and should be resolved prior to undertaking prospective randomized control trials. These include:
Defining the optimal surgical technique, including lead depth (avoiding painful dermal or muscle stimulation) and anchoring.
Determining optimal lead and pulse generator–lead configuration, durability, and generator energy output with specific stimulation modes and parameters for the PNS.
Defining the patients who would most likely benefit from these techniques. Presently, patients who have failed all other measures with a favorable psychological profile are selected.
Selecting the optimal trial interval that balances infectious risks while ruling out a placebo effect.
Presently, the equipment used for stimulating nerves in the periphery is for an “off label” use of spinal cord stimulator devices. Thus, there is little by way of modeling studies and preclinical work to determine the optimal device design. Most complications for PNS/PNFS are minor and easily treated. Two frequent problems are lead migration and skin erosion. Although the blame can be laid on surgical technique, it is important not to lose sight of the fact that insertion techniques, lead size, and anchors were not designed with stimulation of the periphery in mind.
Over the past two decades, electrical stimulation of structures in the epidural space has gone beyond the dorsal columns. These structures include the dorsal horn, dorsal root entry zone (Fig. 90-4), dorsal root ganglia (Fig. 90-5), and posterior nerve roots (Fig. 90-6).29 Classic anterograde epidural SCS targets the dorsal columns (Fig. 90-7), resulting in large myelinated A-β fiber axons undergoing depolarization. Antidromic activation of large myelinated tracts inhibits overactive wide dynamic range neurons of the dorsal horn via activation of interneurons in neuropathic pain states.41 Similar mechanisms are thought to occur with A-β fiber activation in the nerve root/dorsal root ganglion. Additional potential anatomic targets include dorsolateral funiculus and the activation of a supraspinal negative feedback loop. Although direct activation of the dorsolateral funiculus is debated, the orthodromic activation of the dorsal columns can result in excitation of the anterior pretectal nucleus from which the dorsal horn is inhibited by the descending dorsolateral funiculus, which releases serotonin and norepinephrine.42 The neuromodulation of these targets is thought to result in suppression of allodynia, hyperesthesia, and constant pain. Lastly, alteration in sympathetic activity is noted in patients who have an SCS device that results in inhibition and increased blood flow to the periphery.43 Preclinical studies support the modulatory effects of SCS on the sympathetic nervous system as determined by pharmacological inhibitors of sympathetic outflow.44,45
FIGURE 90-4.
Dual stimulating 8 contact electrodes placed in the cervical periforaminal region with stimulating targets of the dorsal root entry zones and the corresponding exiting nerve roots in anteroposterior view. N.B. The lateral image reveals the stimulating lead on the mid-point of the facet pillars (compare/contrast to Figure 90-7 lateral view).

Although the gate control theory may explain the primary mechanism by which SCS works, it cannot account for some of the clinically observed effects of SCS. Some researchers have proposed that SCS inhibits transmission of painful impulses partly by inducing a differential conduction block of afferent nociceptive fibers via antidromic stimulation. That the effects of stimulation can outlast the duration of the stimulation would seem, however, to indicate that this is not the only mechanism by which neurostimulation influences pain transmission.
Investigators have speculated that neurohumoral mechanisms are also involved. Studies have been conducted to elucidate which mediators may contribute. Substances that have been purported to be involved in the neuromodulatory effects of SCS include endogenous opioids, (γ)-[gamma] aminobutyric acid (GABA), adenosine, substance P, serotonin, calcitonin gene-related peptide (CGRP), acetylcholine, and nitric oxide. Several mediators may also play a role in the sympathetic inhibitory effects of SCS. Table 90-3 summarizes the role of putative neurotransmitters in the neurochemistry of SCS mechanisms.
Neurotransmitters in Putative Neurochemical Mechanisms of Spinal Cord Stimulation as Determined in Preclinical Studies
Neurotransmitter | Proposed Modulatory Effect |
Opioid receptors (μ, κ) | No reversal of SCS analgesia but of low frequency transcutaneous electrical stimulation (TENS).46 |
γ-aminobutyric acid (GABA) | Suppression of allodynia and hyperalgesia correlate with release in dorsal horn by electrical stimulation.47,48 Baclofen, a GABA agonist enhances SCS analgesic effects.49 GABA release suppresses the release of excitatory amino acids.50 |
Adenosine | Experiments that parallel those used to evaluate the role of GABA in the allodynia-suppressing effect of SCS have been done using adenosine agonists and antagonists.51–53 |
Serotonin | Elevation in dorsal horn results in diminution of tactile and cold hyperalgesia.54 |
Substance P | Marked increase in the dorsal horn with SCS in the cat functioning as an inhibitor of pain signaling.55 |
Calcitonin-gene related peptide | Antidromic stimulation of the dorsal roots results in peripheral release and subsequent vasodilation.56 |
Nitric oxide | Blockade of nitric oxide synthase diminishes peripheral vasodilation independent of SNS.57 |
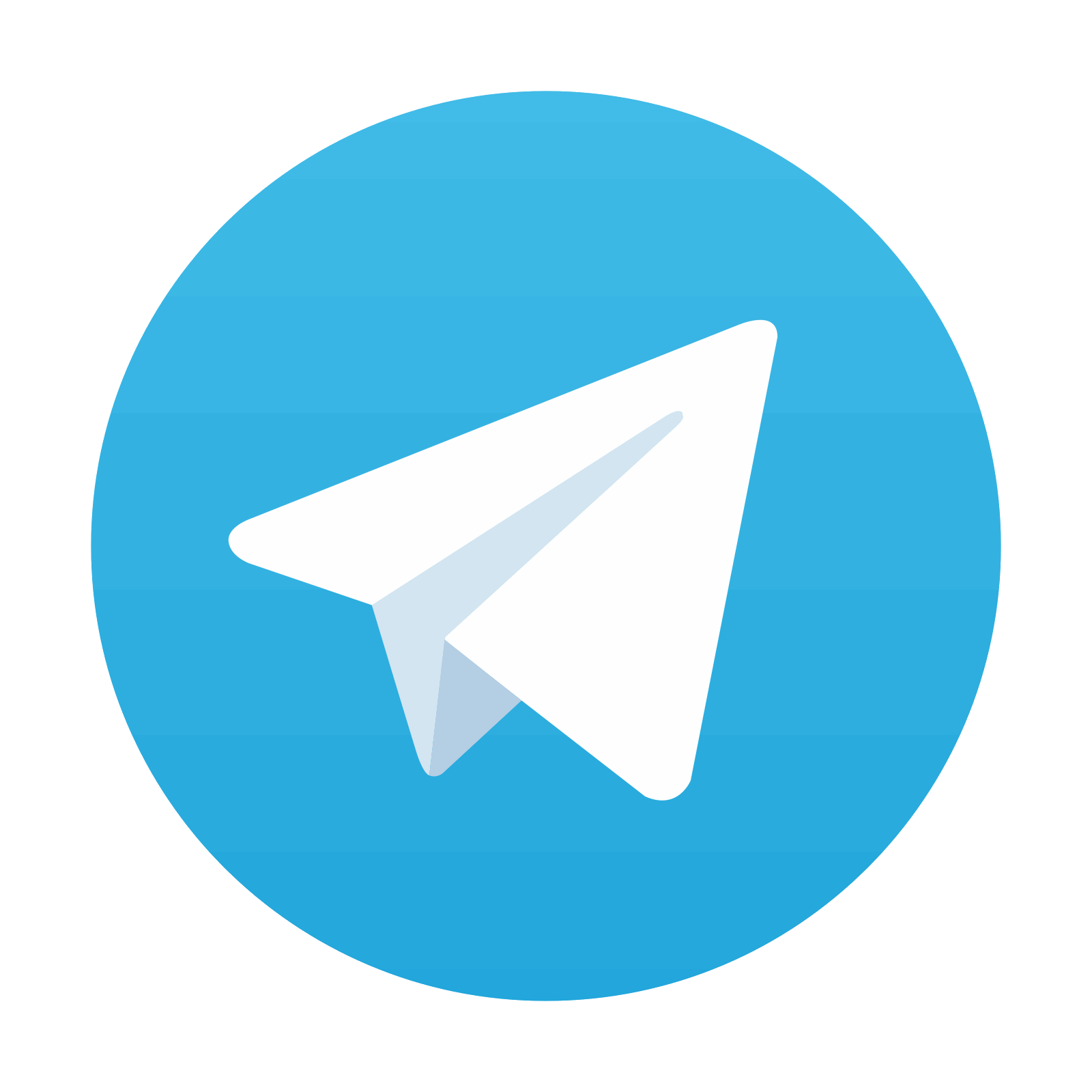
Stay updated, free articles. Join our Telegram channel

Full access? Get Clinical Tree
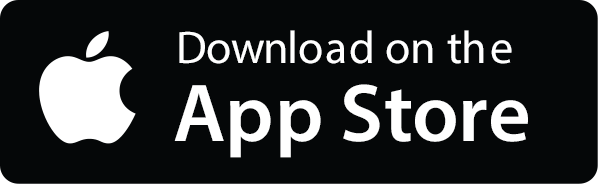
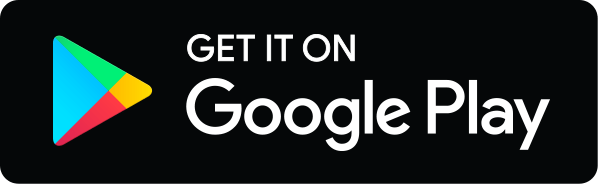
