TABLE 86.2 Predisposing Host Factors to Specific Etiologic Agents of Meningitis | |
![]() |
Nuchal rigidity can be detected with passive or active flexion of the neck. Tests, such as the Kernig and Brudzinski signs, are well-described physical examination techniques but are neither sensitive nor specific (14). Jolt accentuation (worsening of headache with horizontal rotation of the head two to three times per second) also lacks sensitivity (15). Photophobia, seizures, focal neurologic deficits, and papilledema may be seen on physical examination. Some patients may not manifest the classic signs and symptoms of bacterial meningitis, particularly neonates and those with underlying immunosuppressive conditions including diabetes mellitus, chronic organ failure, neutropenia, chronic corticosteroid use, organ transplantation, and HIV infection. Ten percent to 25% of patients with bacterial meningitis present with septic shock (16,17).
Certain microorganisms may present with specific physical findings. Meningococcal meningitis may present with characteristic skin manifestations consisting of diffuse petechiae and purpura on the distal extremities. Severe cases are described as purpura fulminans. Skin findings occur in approximately one-fourth of bacterial meningitis cases, over 90% of which are due to N. meningitidis infection (3).
S. pneumoniae is the most common cause of bacterial meningitis in adults, accounting for 71% of cases in the United States (2). S. pneumoniae serotypes causing bacteremic disease are also those commonly responsible for meningitis. Focal infection is common with contiguous or distant sites, including sinusitis, mastoiditis, pneumonia, otitis media, and endocarditis. The major risk factors for pneumococcal meningitis include asplenia, hypogammaglobulinemia, alcoholism, chronic renal or hepatic disease, malignancy, diabetes mellitus, basal skull fracture with CSF leak, and the presence of a cochlear implant.
N. meningitidis commonly causes meningitis in children and young adults. Serogroups B, C, and Y are responsible for most endemic disease in North America (18). Following increasing use of serogroup C and quadrivalent (A, C, Y, W-135) meningococcal vaccines, serogroup B has become the predominant serotype (19). Epidemic disease is most commonly caused by serogroup C, with fewer outbreaks due to serogroup A. In 2000, epidemic W-135 was associated with the Hajj pilgrimage to Mecca in Saudi Arabia (20). Subsequently, meningococcal vaccination has become legally required prior to undertaking this activity. More recent outbreaks have occurred in Gambia and Burkina Faso (21,22). Risk factors for invasive meningococcal disease include nasopharyngeal carriage, terminal complement deficiency, and properdin deficiency (23,24). Although a characteristic rapidly evolving petechial or purpuric rash strongly suggests N. meningitidis, a similar rash may be seen in splenectomized patients with overwhelming S. pneumoniae or Haemophilus influenzae type b infection.
H. influenzae previously accounted for a large proportion of cases of bacterial meningitis; however, widespread vaccination against H. influenzae type b has now markedly decreased its incidence. Isolation of H. influenzae type b in adults suggests the presence of an underlying condition such as sinusitis, otitis media, pneumonia, diabetes mellitus, alcoholism, CSF leak, asplenia, or immune deficiency.
Listeria monocytogenes meningitis accounts for only 2% of cases of bacterial meningitis in the United States (2) and is associated with similar mortality rates compared to nonlisterial cases. It occurs in neonates, adults older than 50 years of age, and in those with risk factors including alcoholism, malignancy, pregnancy, and immune suppression secondary to corticosteroid therapy or organ transplantation. It is interesting that this infection is seen infrequently in HIV-infected patients; we postulate this may be due to antibiotic prophylaxis—most often with trimethoprim/sulfamethoxazole, which is active against listeria—in HIV patients with low CD4 counts. Pregnant women may be asymptomatic carriers and transmit infection to their infants. L. monocytogenes commonly makes up part of the fecal flora of farm animals and can be isolated from soil, water, or contaminated vegetables. Outbreaks have been associated with unpasteurized dairy products such as milk and cheese, as well as vegetables, and processed meats (25–27).
Aerobic gram-negative bacilli can cause meningitis in specific patients. Predisposing risk factors include recent neurosurgery, neonatal status, advanced age, immune suppression, gram-negative bacteremia, and disseminated Strongyloides stercoralis hyperinfection syndrome. Escherichia coli is a common cause of meningitis in neonates.
Staphylococcus aureus and coagulase-negative staphylococci (CoNS) can both cause meningitis, but are less common. Both Staphylococcal species exist as part of the normal skin flora, predominantly causing infections following neurosurgery or neurotrauma, or when prosthetic material is present, particularly external ventricular drains or ventriculoperitoneal shunts. Some patients with staphylococcal bacterial meningitis have underlying infective endocarditis, paraspinal or epidural infection, sinusitis, osteomyelitis, or pneumonia.
Other less common causes of bacterial meningitis include Enterococci, viridans group Streptococci, β-hemolytic Streptococci, Corynebacterium species (diphtheroids) and Propionibacterium acnes (generally only in the setting of prosthetic material), and anaerobic species.
Viruses are the most commonly isolated pathogens in aseptic meningitis. The nonpolio enteroviruses, especially Coxsackie viruses A and B, and echoviruses are common (28), accounting for 85% to 95% of all cases of aseptic meningitis with an identified pathogen (29). Enteroviruses occur worldwide, are transmitted by fecal–oral or respiratory droplet spread, and exhibit summer and fall seasonality in temperate climates. Infants, children, and young adults are commonly affected. Clinical manifestations depend on host age and immune status but generally include abrupt onset of severe headache, fever, nausea, vomiting, photophobia, nuchal rigidity, and malaise. Rash and upper respiratory symptoms are common. Only rarely is illness severe enough to require critical care services.
Arboviruses more commonly cause encephalitis but, rarely, may cause aseptic meningitis. Arboviruses include the flaviviruses (St. Louis encephalitis virus, Colorado tick fever, Japanese encephalitis virus, and West Nile virus), Togaviridae (Eastern equine encephalitis [EEE], Western equine encephalitis [WEE], and Venezuelan equine encephalitis [VEE]), and California serogroup encephalitis viruses, almost all of which are due to La Crosse virus. Arboviruses occur predominantly in the summer and early fall when vector exposure is most likely.
West Nile virus (WNV) came to widespread attention in 1999 when the first North American cases were identified. The virus subsequently spread extensively across North America, and caused three large outbreaks in 2002, 2003, and 2012 (30). WNV infection is asymptomatic in 80% of cases. Symptomatic patients present with West Nile fever (approximately 20%–25%) (31) or neuroinvasive disease (<1%). WNV fever is a self-limited febrile illness characterized by fever, headache, malaise, myalgias, and often a rash (50%). WNV neuroinvasive disease may present as encephalitis, meningitis, or flaccid paralysis. Meningitis, however, is the least common presentation of neuroinvasive disease. Infections occur in late summer or early fall, as nearly all human infections are due to mosquito bites. Rarely, transmission can occur in utero or via donated blood or organ transplantation.
Lymphocytic choriomeningitis (LCM) virus is a zoonotic infection, transmitted by contact with infected rodent (mouse, rat, hamster) secretions or excretions, or rarely via organ transplantation (32–34), which causes aseptic meningitis (35,36). Presenting manifestations include systemic symptoms of fever, myalgias, and malaise, as well as headache and meningismus, with occasional rash, orchitis, arthritis, myopericarditis, and transient alopecia.
Six of the eight recognized human herpesviruses can cause meningitis. Herpes simplex viruses (HSV) are most commonly associated with aseptic meningitis during primary genital infection (37). HSV-2 infection is responsible for most infections, however, HSV-1 genital infection and concomitant meningitis can also occur. Meningitis is much less likely in the setting of genital herpes recurrences. Headache, photophobia, and meningismus are common presenting symptoms. Genital lesions are present in 85% of patients with primary HSV-2 meningitis and generally precede meningeal symptoms by several days.
Herpes zoster aseptic meningitis, with or without typical skin lesions, has also been reported, particularly in older patients. Cytomegalovirus (CMV), Epstein–Barr virus (EBV), and human herpesvirus 6 (HHV-6) are all capable of causing aseptic meningitis but occur very rarely, predominantly in immune-suppressed populations.
HIV-associated aseptic meningitis occurs with primary infection in approximately 5% to 10% of patients (37). Cranial neuropathies may be present along with headache, fever, and meningismus. Symptoms are usually self-limited.
Mumps, now rare as a result of universal vaccination programs, was once a relatively common cause of aseptic meningitis. The clinical manifestations include fever, vomiting, headache, and parotitis in approximately 50% of patients. Meningismus, lethargy, and abdominal pain may also be present. Sporadic outbreaks in susceptible individuals continue to occur worldwide including the United States (38–40).
There are a number of less common causes of aseptic meningitis. Spirochetal meningitis may be caused by Treponema pallidum or Borrelia burgdorferi. T. pallidum, the etiologic agent of syphilis, is acquired by sexual contact, placental transfer, or direct contact with active lesions; these include condyloma lata, mucous patches, or the rash of secondary syphilis. Syphilitic meningitis usually occurs during primary or secondary infection, complicating up to 2% of untreated infections during the first 2 years. B. burgdorferi is transmitted by the Ixodes tick and causes Lyme disease. It is the most common vector-borne disease in the United States. Meningitis can occur during the first stage of disease, concurrently with erythema migrans at the tick bite site. Dissemination of the microorganism in the second stage of disease, 2 to 10 weeks following exposure, may also result in aseptic meningitis. Late or chronic disease may include subacute encephalopathy but not meningitis.
Mycobacterium tuberculosis may cause a subacute or chronic form of meningitis. Infection of the meninges results from rupture of a tuberculous focus into the subarachnoid space. In very young patients, concomitant disseminated systemic infection is common. Epidemiologic risk factors include a known prior history of tuberculosis (TB) exposure, residence in an endemic area, contact with an active case, incarceration, homelessness, and HIV infection. Tuberculin skin testing (TST) is negative in over half of patients with tuberculous meningitis (41,42). A negative skin test, therefore, cannot be used to exclude the diagnosis. Newer tests, such as interferon-gamma release assays (IGRA) and nucleic acid amplification tests (NAT) may be available in some centers.
Fungal meningitis, although uncommon, should be considered particularly given the high mortality associated with untreated infection. Cryptococcus neoformans predominantly affects immune-compromised hosts but can also infect the immunocompetent. This encapsulated yeast is distributed worldwide but prefers wet-forested regions with decaying wood and is found in particularly high concentrations in pigeon guano. Risk factors for cryptococcal infection include HIV/AIDS, prolonged corticosteroid therapy, immunosuppression transplantation, malignancy, and sarcoidosis. Clinical presentation is typically indolent, occurring over 1 to 2 weeks, and is characterized by fever, malaise, and headache. Meningismus, photophobia, and vomiting occur in less than one-third of patients. Cryptococcus gattii, a serotype usually restricted to tropical climates, emerged on Vancouver Island, British Columbia (BC), Canada in 1999 and has since been responsible for numerous cases of CNS infection in predominantly immunocompetent hosts in BC and the U.S. Pacific Northwest.
Coccidioides immitis, a dimorphic fungus, is found in soil in the dry desert regions of the southwest United States, Mexico, and Central and South America. Infection results after inhalation of arthroconidia, usually following a dust storm or during building construction. Infection is usually confined to the respiratory system in those with competent immune systems. However, extrapulmonary dissemination to the meninges can occur in patients with immune compromise or during pregnancy. Risk factors for the development of disease include travel to or residence in an endemic region and immune deficiency. Coccidioidal meningitis is universally fatal if untreated.
Less common fungal causes of meningitis include Blastomyces dermatitidis, Histoplasma capsulatum, Sporothrix schenckii, and rarely, Candida species. B. dermatitidis and H. capsulatum are endemic in the Mississippi and Ohio River Valleys. S. schenckii has been reported worldwide, with most cases in the tropical regions of the Americas.
Candida is part of the normal flora of skin and gastrointestinal tract. Candidal CNS infection is most commonly the result of meningeal seeding in candidemic patients. Predisposing risk factors for candidemia include the use of broad-spectrum antibiotics, the presence of indwelling devices such as vascular or urinary catheters, parenteral nutrition, ICU admission, prolonged hospital stay, and immune compromise. Specific risk factors for Candida CNS infection include ventricular shunts, trauma, neurosurgery, or lumbar puncture (43,44). Candida albicans is the most commonly isolated species; however, non-albicans species are becoming more prevalent, particularly in ICU populations.
Meningitis caused by protozoa or helminths are extremely rare. The free-living amoebas Acanthamoeba, Balamuthia, and Naegleria fowleri are associated with fresh water exposure. They are usually acquired by individuals diving into contaminated lakes or swimming pools. N. fowleri can cause a primary amoebic meningoencephalitis. Acanthamoeba and Balamuthia rarely cause meningitis; they commonly present as encephalitis. As for helminths, Angiostrongylus cantonensis (the rat lungworm) is the classic infectious cause of eosinophilic meningitis (>10% eosinophils in the CSF) (Table 86.3). Humans are incidental hosts and develop neurologic symptoms as a result of larval migration through the CNS. A. cantonensis is endemic in Southeast Asia and the Pacific Islands and is acquired by ingesting raw mollusks such as snails or slugs. Gnathostoma spinigerum, acquired by ingestion of raw and undercooked fish and poultry, is not primarily neurotropic like A. cantonensis but may also cause eosinophilic meningitis as a result of migration of larvae up nerve tracts to the CNS. Gnathostomiasis is endemic in Asia, especially Thailand and Japan, and more recently in Mexico. Baylisascaris procyonis, a roundworm infection of raccoons, rarely causes human eosinophilic meningoencephalitis following accidental ingestion of ova from raccoon feces in contaminated water, soil, or foods (45).
TABLE 86.3 Cerebrospinal Fluid Tests in Suspected CNS Infection | |
![]() |
DIAGNOSIS
Lumbar puncture (LP) should be performed emergently in all patients suspected of having bacterial meningitis unless contraindicated. Unnecessary delays are unfortunately common while neuroimaging is performed to exclude mass lesions. Complications associated with LP are uncommon but may include post-LP headache, infection, bleeding, radicular pain or paresthesias, back pain, and very rarely cerebral herniation (46). A study evaluating the clinical features at baseline associated with abnormal findings on computed tomography (CT) scan, and thus, increased risk of brain herniation, identified: age greater than or equal to 60 years; a history of CNS disease such as a mass lesion, stroke, or focal infection; immune compromise such as HIV or immunosuppressive therapy; a history of seizure within 1 week of presentation; and focal neurologic findings (47). Based on these findings, guidelines for which adult patients should undergo CT prior to LP have been recommended (Table 86.4) (48).
Nosocomial meningitis is rare in nonneurosurgical patients; nevertheless, LP is often performed in hospitalized patients with unexplained fever and/or decreased level of consciousness. The yield of performing an LP in the nonneurosurgical population is extremely low and of questionable utility.
TABLE 86.4 Indications for Imaging Prior to Lumbar Puncture in Adults with Suspected Bacterial Meningitis |
![]() |
CSF analysis is extremely important in the diagnosis of meningitis. Basic laboratory analyses, including cell count and differential, protein, glucose, Gram stain, and bacterial cultures, are most useful in distinguishing between viral, bacterial, tuberculous, and fungal infection (see Table 86.3).
Bacterial Meningitis
Bacterial meningitis usually presents with an elevated systemic white blood cell (WBC) count due to neutrophilia and left shift (immature forms such as bands and/or myeloids). Leukopenia is occasionally present in severe infection. Thrombocytopenia may be the result of sepsis, disseminated intravascular coagulation, or meningococcemia alone. Renal and hepatic dysfunction may occur as part of multiorgan failure in severe disease. Blood cultures should always be drawn prior to the administration of antimicrobials, particularly if an LP cannot be performed immediately and are positive in 50% to 80% of cases (3,12).
CSF analysis in bacterial meningitis classically reveals a neutrophilic pleocytosis with hundreds to thousands of cells and greater than 80% neutrophils. In fact, a low CSF WBC count is usually a marker of poor prognosis in this setting. The CSF glucose concentration is usually low and should always be compared with a simultaneous serum glucose measurement. An abnormal CSF-to-serum glucose ratio (<0.5) is common in bacterial meningitis—and is often much lower than 0.5. Acute illness in diabetics may increase serum glucose levels markedly, making the CSF-to-serum glucose ratio inaccurate. CSF lactate levels may be useful in distinguishing bacterial from aseptic meningitis (49–51). CSF protein and opening pressure are usually elevated in bacterial meningitis (Table 86.5).
TABLE 86.5 Typical CSF Parameters in Patients with Meningitis |
![]() |
Gram staining permits rapid identification of bacterial species—with a sensitivity of 60% to 90% and specificity of close to 100% in patients with bacterial meningitis (3,51). The Gram stain is more likely to be positive in patients with high bacterial loads. Gram-positive diplococci suggest S. pneumoniae infection, gram-negative diplococci suggest N. meningitidis infection, gram-positive rods suggest L. monocytogenes infection, and small pleomorphic coccobacilli suggest H. influenzae infection.
CSF bacterial cultures are positive in approximately 80% of cases (5,12). The yield decreases significantly in patients treated with antimicrobials prior to CSF collection. Antigen assays (latex agglutination tests) have been used in these cases, but due to their low sensitivity are no longer routinely offered by many laboratories. Broad-based NAT (52) and 16S rDNA-based gene sequencing (53) may be useful for the diagnosis of culture-negative meningitis.
Viral Meningitis
In acute viral meningitis, the CSF cell count is usually in the low hundreds with a lymphocytic predominance. A predominance of neutrophils may be seen in the first 24 hours of disease, occasionally confusing the diagnosis. The CSF glucose concentration is usually within normal range. CSF protein is often mildly elevated, and the opening pressure is usually normal.
Viral cultures and NAT are most commonly used in the diagnosis of viral meningitis, but NAT is more sensitive. Enteroviruses may be cultured from CSF, throat, or rectal swabs, however, CSF NAT testing is both more sensitive and specific. NAT for HSV is also widely available, and in HSV-1 encephalitis, HSV NAT demonstrated a specificity of 99% and sensitivity of 96% when CSF was studied between 48 hours and 10 days from symptom onset (54). False negatives occur mostly within the first 72 hours of infection. The diagnosis of WNV neuroinvasive disease can be made by detection of serum IgM or a fourfold rise in IgG between acute and convalescent titers. WNV NAT of serum and CSF are also available; however, the sensitivity is higher in CSF due to short-lived viremia in humans.
Other Less Common Causes
CSF analysis in syphilitic meningitis is characterized by a mild lymphocytic pleocytosis, decreased glucose, and elevated protein. T. pallidum cannot be cultured, so diagnosis must be made using alternate methods, predominantly serology. Direct visualization by darkfield microscopy or direct fluorescent antibody testing may be possible if a primary chancre or skin lesion of secondary syphilis—condyloma latum or mucous patch—is present. While Treponema-specific enzyme immunoassays (EIA) for IgM and IgG have largely replaced traditional serologic tests, CSF VDRL may be used in the diagnosis of syphilitic meningitis. The specificity is high, but false positives may occur in bloody specimens. The major limitation of CSF VDRL is its low sensitivity, so a negative result should not be used to rule out infection. CSF FTA-Abs is more sensitive; however, false positives are common due to serum antibody leak into the CSF. Last, NAT has been used to detect T. pallidum DNA in the CSF but lacks sensitivity and is not available in all centers (55–57).
Lyme meningitis is characterized by a mild lymphocytic pleocytosis, low glucose, and elevated protein. The CSF concentration of B. burgdorferi antibody, compared to serum levels, is a sensitive and specific diagnostic method. NAT is currently available only in research laboratories and does not differentiate between infection and remnant DNA from prior cured infection (58). CSF oligoclonal bands and B. burgdorferi culture are also available, but neither is sensitive or specific.
The CSF analysis in tuberculosis meningitis demonstrates a lymphocytic pleocytosis, low glucose, and markedly elevated protein and opening pressure. The elevation in protein is particularly marked in the setting of CSF block. Acid-fast bacillus (AFB) smears are generally low yield, but may be optimized by sending large volumes (10–15 mL) of CSF (59). Mycobacterial cultures, although slow growing—taking several weeks—become positive in approximately 70% of cases (59). DNA probes and NAT have recently become available with great improvements in sensitivity and specificity (60). Meningeal biopsy is rarely performed but may show caseating granulomata. Sputum and urine AFB, as well as mycobacterial blood cultures, should also be included as part of the TB workup in these patients. Since CSF AFB microscopy has very low sensitivity and cannot exclude the diagnosis of TB, in most cases the combination of increased WBCs and protein, decreased glucose and negative conventional cultures may be suggestive of TB meningitis, especially in a patient with epidemiologic risk factors for TB (i.e., foreign-born) or positive TST or IGRA, and should prompt consideration of empiric anti-TB therapy.
Cryptococcal meningitis is characterized by a lymphocytic pleocytosis, decreased glucose, and elevated protein. Opening pressures may be markedly elevated. Culture of C. neoformans or C. gattii from the CSF is diagnostic; however, other simpler tests are now available. Detection of serum or CSF cryptococcal antigen (CrAg) is highly sensitive (>90%) (61). India ink was previously regarded as the standard diagnostic test, but due to its low sensitivity it has been largely replaced by antigen testing. Fungal blood cultures may also be useful, as cryptococcal meningitis occasionally occurs in the setting of disseminated cryptococcal disease, especially in HIV-infected patients.
Other fungal meningitides are similarly characterized by a lymphocytic pleocytosis, low to normal glucose, and an elevated protein. Coccidioidal meningitis may present with an eosinophilic pleocytosis and peripheral eosinophilia. Fungal cultures are diagnostic and are most useful in Candida or Aspergillus infection. Dimorphic fungal infection may be diagnosed serologically, as isolating these organisms from the CSF is challenging and of low yield (62). Detection of complement-fixing (CF) IgG antibodies or immunodiffusion tests for IgM and IgG in CSF are currently the standard diagnostic tests. Low-titer false positives may occur in the setting of parameningeal foci. As well, false negatives may occur in early disease.
Primary amoebic meningoencephalitis due to N. fowleri results in a neutrophilic pleocytosis, increased red blood cells, low glucose, and an elevated protein. Demonstration of motile trophozoites on a wet mount of CSF or biopsy specimens is diagnostic. The diagnosis of A. cantonensis, G. spinigerum, or B. procyonis requires an appropriate epidemiologic exposure, peripheral blood eosinophilia, and a characteristic eosinophilic pleocytosis. Serologic tests are helpful but performed only in reference laboratories.
TREATMENT
The initial management of the patient with suspected meningitis is primarily guided by epidemiologic risk factors and LP results. The CSF cell count, glucose, and Gram stain are crucial in guiding empiric therapy. If the LP is delayed for any reason, empiric antimicrobial therapy should not be withheld (Table 86.6), as delays in therapy have been associated with adverse clinical outcomes and increased mortality (63–65). The administration of antimicrobials should immediately follow blood culture collection and should not be delayed by neuroimaging or other tests performed prior to LP.
TABLE 86.6 Empiric Therapy of Bacterial Meningitis Based on Age and Host Factors | ||
![]() |
LP should be performed urgently in those with suspected meningitis. A protocol for the management of bacterial meningitis is presented in Figure 86.1. Imaging should be performed prior to LP in specific populations (see Table 86.4) but should not result in delays in antimicrobial therapy. Empiric therapy should be based on age, underlying host factors, and initial CSF Gram stain results, if available (see Table 86.6).
Bacterial Meningitis
The choice of antimicrobial therapy in bacterial meningitis is influenced by blood–CSF barrier penetration, effect of meningeal inflammation on penetration, and bactericidal efficacy. In general, CSF penetration is enhanced in the setting of meningeal inflammation due to increased permeability. Additionally, high lipid solubility, low molecular weight, and low protein binding increase CSF drug levels. Bactericidal efficacy may be decreased in purulent CSF, particularly with aminoglycosides, due to low pH. Penicillins, third-generation cephalosporins, carbapenems, fluoroquinolones, and rifampin achieve high CSF levels and each are bactericidal. While antimicrobials may need to be adjusted based on renal and hepatic function, the doses that follow all assume normal renal function. Therapeutic drug monitoring may be required to ensure adequate levels and prevent toxicity (e.g., vancomycin, aminoglycosides). Antimicrobial therapy should also be adjusted based on culture and susceptibility results as soon as possible (Table 86.7). In suspected meningococcal or H. influenzae meningitis, droplet isolation (single room, gowns, gloves, surgical masks, eye protection and dedicated equipment) should be enforced until 24 hours of effective antimicrobial therapy have been completed or an alternate diagnosis is reached. Isolation in other cases of meningitis, including pneumococcal meningitis, is not required.
Streptococcus pneumoniae
Empiric therapy guidelines for pneumococcal meningitis have been recently modified due to increasing penicillin resistance (66–68). S. pneumoniae was once uniformly susceptible to penicillin; however, mutations in penicillin-binding proteins have resulted in varying levels of resistance. Empiric therapy therefore consists of a third-generation cephalosporin and vancomycin until susceptibility results become available (48). The recommendation to add vancomycin, however, is based only on a handful of pneumococcal meningitis cases demonstrating third-generation cephalosporin resistance (minimum inhibitory concentration [MIC] ≥2 μg/mL). It should also be noted that vancomycin penetrates the CSF poorly, particularly when dexamethasone is administered concomitantly, so aggressive dosing is required to achieve target trough serum concentrations. Once MICs are available, therapy should be adjusted accordingly. For isolates with penicillin MIC less than 0.06 μg/mL, penicillin G (4 million units IV every 4 hours) or ampicillin (2 g IV every 4 hours) should be used. For isolates with a penicillin MIC of 0.12 μg/mL or higher and ceftriaxone MIC below 1 μg/mL, treatment with a third-generation cephalosporin should be continued; either cefotaxime (2 g IV every 6 hours) or ceftriaxone (2 g IV every 12 hours). For isolates with a ceftriaxone MIC greater than or equal to 1 μg/mL, vancomycin and a third-generation cephalosporin are the recommended therapy; some clinicians administer very high doses of third-generation cephalosporins in these cases. Vancomycin should be dosed 15 to 20 mg/kg/dose (based on actual body weight) every 8 to 12 hours. Subsequent dosing should be adjusted based on serum trough vancomycin concentrations (between 15 and 20 μg/mL). A loading dose of 25 to 30 mg/kg (maximum 2 g) may be used to rapidly achieve target concentrations. Meropenem is a reasonable alternative to the above agents and does not carry the theoretical risk of decreasing seizure threshold as with imipenem. The efficacy of linezolid, daptomycin, dalbavancin, and telavancin may be promising for the treatment of highly resistant pneumococcal strains (69,70). Antimicrobial treatment duration is 10 to 14 days. Dexamethasone should be administered prior to or with the first dose of antimicrobial.
Neisseria meningitidis
The empiric treatment of meningococcal meningitis should be a third-generation cephalosporin: cefotaxime (2 g IV every 6 hours) or ceftriaxone (2 g IV every 12 hours); however, therapy should be stepped down to penicillin if susceptibility is confirmed. The duration of treatment is 7 days. Chloramphenicol (25 mg/kg, to a maximum of 1 g IV every 6 hours) is a reasonable alternative in the β-lactam–allergic patient. Meropenem (2 g IV every 8 hours) is another alternative, although there may be cross-reactivity in penicillin-allergic patients. Dexamethasone is not indicated in confirmed meningococcal meningitis.
Haemophilus influenzae
Empiric therapy for H. influenzae meningitis is a third-generation cephalosporin. Therapy can be narrowed to ampicillin, 2 g IV every 4 hours if susceptibility is confirmed. A total of 7 days of therapy is recommended. Dexamethasone should be administered as adjunctive therapy in children (71).

FIGURE 86.1 Bacterial meningitis protocol.
Listeria monocytogenes
L. monocytogenes meningitis should be treated with ampicillin 2 g IV every 4 hours. Gentamicin may be added for antimicrobial synergy, but aminoglycosides have poor penetration into CSF and have significant toxicities. When used, gentamicin should be administered as a 2 mg/kg loading dose, followed by 1.7 mg/kg every 8 hours. Trimethoprim/sulfamethoxazole (TMP/SMX), 20 mg/kg/day of the trimethoprim component, divided into 6 to 12 hourly doses, may be used in penicillin-allergic patients. Alternate therapies include meropenem and, potentially, linezolid and rifampin. Third-generation cephalosporins have no activity against L. monocytogenes. Treatment duration is 14 to 21 days.
Aerobic Gram-Negative Bacilli
Aerobic gram-negative bacilli should be treated empirically with a third-generation cephalosporin or meropenem. Susceptibility results should guide therapy in consultation with an infectious diseases specialist. For Pseudomonas aeruginosa infections, ceftazidime or cefepime, 2 g IV every 8 hours, or meropenem, 2 g IV every 8 hours, with tobramycin 2 mg/kg IV every 8 hours, should be used. Ciprofloxacin or aztreonam are acceptable alternatives if the isolate is susceptible. The duration of therapy is prolonged, generally 21 days.
TABLE 86.7 Specific Therapy of Bacterial Meningitis | |
![]() |
Staphylococcus aureus
Staphylococcal meningitis therapy depends on methicillin susceptibility. Methicillin-susceptible strains should be treated with nafcillin 2 g IV every 4 hours, whereas methicillin-resistant (MRSA) strains should be treated with vancomycin, 15 to 20 mg/kg/dose IV every 8 to 12 hours. Subsequent vancomycin dosing should be adjusted based on serum trough vancomycin concentrations (goal trough 15 to 20 μg/mL). A loading dose of 25 to 30 mg/kg (maximum 2 g) may be used to rapidly achieve target concentrations. Vancomycin is also recommended in patients with serious penicillin allergies. Infected prosthetic material should be removed if possible and antimicrobial therapy continued for 14 days minimum after removal. If removal is not possible, rifampin may be added; however, cure rates are poor with hardware retention. Linezolid, TMP/SMX, daptomycin, telavancin, and dalbavancin may be used as alternate therapies in MRSA meningitis when vancomycin cannot be used or is ineffective (including circumstances of “MIC creep”, when vancomycin MIC >1 μg/mL), however further studies are needed to establish efficacy of these agents. Tigecycline should not be used as therapeutic CSF concentrations are not achieved with standard therapy.
Adjunctive Therapies
Adjunctive therapies in bacterial meningitis include corticosteroids, procedures to reduce intracranial pressure, and surgery. Corticosteroid therapy aims to decrease the inflammatory response while allowing antimicrobial therapy to eradicate infection. Although corticosteroid administration may decrease CSF penetration and bactericidal activity of antimicrobials, randomized controlled trials demonstrate benefit with its use (12). In children, the administration of dexamethasone has demonstrated a reduction in the incidence of hearing impairment and severe neurologic complications in H. influenzae meningitis (71–73). Adjunctive corticosteroid therapy has also been evaluated in adults, showing a mortality benefit in patients with pneumococcal meningitis (12,72). Based on these results, treatment recommendations suggest dexamethasone, 0.15 mg/kg, be given 10 to 20 minutes before, or at least concomitant with, the first dose of antimicrobial therapy and continued every 6 hours. Dexamethasone should be administered to all patients with suspected bacterial meningitis until Gram stain or culture results are available. Therapy should be continued for 2 to 4 days only if the Gram stain or cultures demonstrate H. influenzae in children or S. pneumoniae in adults.
Placement of an intracranial pressure monitoring device may be beneficial for patients with bacterial meningitis and elevated intracranial pressure (ICP). Admission to an ICU with expertise in ICP monitoring and management of intracranial hypertension is most appropriate (74). Standard measures—such as CSF drainage, fluid and sodium management, optimization of cerebral perfusion pressure, sedation, temperature control, hyperventilation, and osmotic therapies—should be employed in patients with high ICP (75). Transcranial Doppler ultrasonography and continuous electroencephalography (EEG) monitoring should be applied where possible. Decompressive craniectomy may be considered in patients when maximal medical ICP treatment fails to reduce severe intracranial hypertension. Other surgical interventions may be required, for example, those with basal skull fractures with persistent CSF leaks or dural defects.
Complications
Complications of bacterial meningitis can be divided into neurologic and nonneurologic complications. Neurologic complications include seizures, cerebral edema or infarction, cranial nerve palsies, venous sinus thrombosis, brain abscess, subdural empyema, and coma. Late complications include hearing impairment, obstructive hydrocephalus, learning disabilities, sensory and motor deficits, mental retardation, cortical blindness, and seizures. Nonneurologic complications include septic shock, coagulopathy, and the syndrome of inappropriate antidiuretic hormone secretion (SIADH).
Viral Meningitis
In general, the treatment for viral meningitis is supportive given its benign and self-limited course. Pleconaril has been evaluated for enteroviral meningitis with modest benefit, but remains experimental (76,77). Intravenous immune globulin has been used in agammaglobulinemic patients with chronic enteroviral meningitis. No specific therapy exists for arboviruses, mumps, or LCM. HIV-associated meningitis should be treated with combination antiretroviral therapy.
It is not clear whether antiviral treatment alters the course of HSV meningitis; nevertheless, primary episodes of genital herpes should be treated as per guidelines. Some physicians extend therapy to 14 days with concomitant meningitis. Intravenous acyclovir, dosed 5 mg/kg every 8 hours, has been used in severe disease. Therapy can be stepped down to an oral agent on discharge. Ganciclovir is the treatment of choice for CMV meningitis in immune-compromised hosts.
Other Less Common Etiologies
Syphilitic meningitis does not respond to benzathine penicillin, which is used to treat most forms of syphilis; it requires a 2-week course of high-dose IV penicillin G: 4 million units every 4 hours. Rapid plasma reagin (RPR) titers should be monitored after therapy, and repeat CSF examination should be performed if titers do not decline by fourfold at 6 months after therapy. All HIV patients with syphilitic meningitis should have repeat LP at 6 months following therapy. Patients with penicillin allergy should undergo desensitization, as there are no proven effective alternative therapies for syphilitic meningitis.
The treatment of Lyme meningitis is achieved with ceftriaxone, 2 g daily, or cefotaxime, 2 g IV every 8 hours for 14 to 28 days. Alternate therapy is penicillin, 4 million units every 4 hours, for 14 to 28 days.
The treatment of tuberculous meningitis depends largely on the expected resistance pattern based on country of acquisition and results of susceptibility testing; consultation with an infectious diseases specialist is strongly recommended. In general, standard combination therapy includes isoniazid (INH), rifampin, pyrazinamide, and a fourth drug, either a fluoroquinolone or an injectable aminoglycoside. Ethambutol penetrates CSF poorly and has been largely replaced by fluoroquinolones. If the isolate is fully susceptible, treatment can be narrowed to INH and RIF alone. For drug-resistant TB, therapy should be prescribed by infectious diseases and/or TB services. Treatment should be continued for a minimum of 12 months but may be prolonged for concomitant tuberculoma or in multi–drug-resistant infection. Adjunctive therapy with dexamethasone for the first 2 months has been shown to decrease mortality as well as neurologic deficits and is recommended (78). Pyridoxine, 25 to 50 mg daily, may be administered to prevent INH-related neuropathy.
Therapy for fungal meningitis is complicated by the lack of standardized susceptibility testing and interpretation for many fungi. The area of antifungal therapy, however, is an evolving area with an increasing number of antifungal agents from which to choose.
Cryptococcal meningitis should be treated with a 14-day induction phase of liposomal amphotericin B, 3 to 5 mg/kg/day IV, with or without flucytosine, 100 mg/kg/day PO dosed every 6 hours. Conventional amphotericin B (deoxycholate) may still be used instead of lipid formulations, however, few patients tolerate this therapy. Consolidation therapy with fluconazole, 400 to 800 mg (6 to 12 mg/kg) daily, should be continued for 8 weeks following induction. Maintenance (or suppressive) therapy with fluconazole, 200 mg per day, should be continued in organ transplant recipients for 6 to 12 months or patients with HIV/AIDS until immune reconstitution is achieved. Cryptococcal meningitis may require initial daily therapeutic LPs, an external ventricular drain, or a ventriculoperitoneal shunt to relieve increased intracranial pressure. Echinocandins, such as caspofungin, micafungin, and anidulafungin are not active in cryptococcosis.
The treatment for coccidioidal meningitis is oral fluconazole, 400 mg/day. Some clinicians initiate therapy with a higher dose of 800 mg/day or may add intrathecal amphotericin B. Treatment must be continued lifelong, as relapses are frequently lethal. Newer azoles such as voriconazole or posaconazole may be tried in patients who are refractory to fluconazole, however evidence to support this is limited.
Therapy for H. capsulatum meningitis consists of liposomal amphotericin B or amphotericin B deoxycholate. Fluconazole, 800 mg/day, for an additional 9 to 12 months, may be used to prevent relapse. If relapse does occur, long-term therapy with fluconazole or intraventricular amphotericin B is recommended.
For candidal meningitis, the preferred initial therapy is IV liposomal amphotericin B 3 to 5 mg/kg/day, with or without flucytosine, 25 mg/kg dosed every 6 hours and adjusted to maintain serum levels of 40 to 60 μg/mL. Fluconazole therapy, in susceptible species, may be used for follow-up or suppressive therapy. The duration of therapy is at least 4 weeks after resolution of symptoms. All prosthetic material must be removed to achieve cure.
Primary amoebic meningoencephalitis caused by N. fowleri is usually fatal. A few cases have had good outcomes with early diagnosis and treatment with high-dose intravenous and intrathecal amphotericin B, rifampicin and steroids to control cerebral edema. Fluconazole, miltefosine, and azithromycin may be additionally prescribed. Eosinophilic meningitis caused by A. cantonensis and G. spinigerum are treated supportively. Corticosteroids are recommended to decrease the inflammatory response to intracranial larvae. Antihelminthic therapy is relatively contraindicated, as clinical deterioration and death may occur following severe inflammatory reactions to dying larvae.
PREVENTION
Chemoprophylaxis (medications) and immunoprophylaxis (vaccines) are available to prevent infection in close contacts of cases or during outbreaks. Temporary nasopharyngeal carriage with H. influenzae, N. meningitidis, and S. pneumoniae may occur following exposure to an index case and is a risk factor for the development of invasive disease. Chemoprophylaxis is recommended to eliminate nasopharyngeal carriage in individuals at risk.
Prophylaxis is indicated in selected household/close contacts (those having more than 4 hours < 3 feet from the index case), and child-care or preschool contacts within 5 to 7 days before onset of disease, of cases of H. influenzae type b. The recommended therapy is rifampin, 20 mg/kg (usual adult dose of 600 mg daily) for four doses, and should be guided by your local public health department.
Prophylaxis for N. meningitidis is also recommended for close contacts of cases. This includes intimate contacts (e.g., kissing) and close contacts with greater than or equal to 4 hours of contact 1 week prior to the onset of illness. Most close contacts include housemates, child-care center contacts, cellmates, and/or military recruits. Medical personnel exposed to oropharyngeal secretions during intubation, nasotracheal suctioning, or mouth-to-mouth resuscitation should also receive chemoprophylaxis. Rifampin, 600 mg orally every 12 hours for a total of four doses, or single doses of ciprofloxacin (500 mg orally) or ceftriaxone (250 mg intramuscularly) are all efficacious. It is recommended that ciprofloxacin be avoided in children younger than 16 years of age and in pregnant women, based on joint cartilage injury demonstrated in animal studies. Chemoprophylaxis is not indicated in S. pneumoniae infection.
Vaccination is available for the prevention of H. influenzae serogroup b, N. meningitidis, and S. pneumoniae infections and is part of routine childhood immunization. Unvaccinated children 2 years of age or younger, exposed to an index case, should receive chemoprophylaxis and vaccination.
S. pneumoniae vaccination is available in two preparations: the 23-valent polysaccharide (PPSV23) vaccine and the 13-valent conjugate (PCV13) vaccine. The conjugate vaccine is recommended routinely in all children 23 months of age or less. Adults at high risk of invasive disease—sickle cell disease and other hemoglobinopathies, functional or anatomic asplenia, HIV infection, immune compromise, and chronic medical conditions—and all patients over 65 years of age should receive both PPSV23 and PCV13. Additional specific vaccine recommendations have been published by the United States Advisory Committee on Immunization Practices (79–81). S. pneumoniae vaccination is not indicated as postexposure prophylaxis.
Several different meningococcal vaccines are available in the United States, including both conjugate and polysaccharide vaccines. Conjugate vaccines are preferred due to superior immunogenicity. Quadrivalent and monovalent vaccines are available and should be offered to high-risk populations, including those with specific immune deficiencies (see Table 86.2), those traveling to endemic and epidemic regions, laboratory workers routinely exposed to N. meningitidis, first-year college students living in dormitories, and military recruits. Vaccination during outbreaks of meningococcal disease due to a serogroup contained in a vaccine should be performed in consultation with public health authorities. A pentavalent meningococcal vaccine is currently under development (82).
ENCEPHALITIS
Encephalitis is defined as inflammation of the brain parenchyma. Although encephalitis and meningitis may present with similar clinical findings, the two syndromes are pathophysiologically distinct. The major distinguishing feature is the presence or absence of normal brain function. Patients with meningitis may be drowsy or lethargic but should have normal cerebral function, whereas those with encephalitis generally have altered mental status. Occasionally patients may present with a combination of findings in an overlap syndrome of meningoencephalitis. It is important to distinguish between the two syndromes, as the etiologic agents and treatments may differ.
Encephalitis is most commonly viral or postinfectious (Table 86.8). Viral encephalitis is caused by direct viral invasion of the CNS whereas postinfectious encephalitis is an immune-mediated process. Unfortunately, it may be difficult to differentiate between the two; however, encephalitis with resolving infectious symptoms suggests a postviral cause. The most common viruses causing postinfectious encephalitis include mumps, measles, varicella zoster virus (VZV), rubella, and influenza.
PATHOPHYSIOLOGY
Access to the CNS is highly virus-specific and occurs via hematogenous or neuronal routes. In hematogenous invasion, viral infection is acquired at an initial site of entry, with primary site replication, transient viremia, and CNS seeding. Retrograde transport within motor and sensory axons to the CNS occurs in the neuronal route of entry. After CNS entry, viruses enter neural cells, causing inflammation and cell dysfunction. Clinical manifestations are the result of specific cell-type invasion. Oligodendroglial cell invasion causes demyelination, whereas cortical invasion results in altered mental status, and neuronal invasion may result in focal or generalized seizures. Thus, focal pathology is the result of specific neural tropism.
Herpes simplex encephalitis (HSE) is the most common cause of sporadic encephalitis in Western countries, accounting for 20% to 40% of cases (83,84). HSE is caused by type 1 virus in greater than 90% of cases, occurs year-round, and affects all age groups. Two-thirds of cases are due to reactivation of the virus in the trigeminal ganglion, with retrograde transport along the olfactory tract to the orbitofrontal and mediotemporal lobes. Untreated HSE has a mortality rate up to 70%, and almost all survivors suffer neurologic sequelae (85,86). Outcomes correlate strongly with the severity of disease at presentation, as well as the time to initiation of antiviral therapy. Other herpes viruses, such as VZV and HHV-6 can rarely cause encephalitis; however they generally affect immune-compromised patients. VZV encephalitis may occur with or without concomitant cutaneous lesions.
Arboviruses are acquired via vector exposure, mainly mosquitoes and ticks. These include EEE, WEE, St. Louis encephalitis, VEE, California encephalitis (caused in most cases by La Crosse virus), Japanese encephalitis, yellow fever, and WNV. Arbovirus-related encephalitides are geographically specific and most prevalent during the summer and early fall months when mosquitoes and ticks are most active.
West Nile virus, first identified in North America in 1999, causes neuroinvasive disease in less than 1% of exposed individuals. It is, however, now the most commonly diagnosed arboviral CNS infection in the United States (87). Neuroinvasive disease most frequently manifests as encephalitis and occurs in those with comorbid disease (88) such as diabetes mellitus, hypertension, renal disease, malignancy, organ transplantation, alcoholism, and advanced age (89–91). Muscle weakness and flaccid paralysis may present concurrently in patients with encephalitis.
Rabies, a zoonotic disease that requires contact with infected animals, should be considered in all cases of encephalitis. Once CNS infection is established, however, the mortality is essentially 100% although survival with aggressive therapy has recently been described (92,93). Rabies can be acquired from many sources including dogs, cats, raccoons, bats, and foxes. The history of an animal bite, although useful if present, is absent in most cases of rabies.
TABLE 86.8 Most Common Viral Causes of Encephalitis, Their Vectors or Animal Hosts, and Geographic Distributions | ||
![]() |
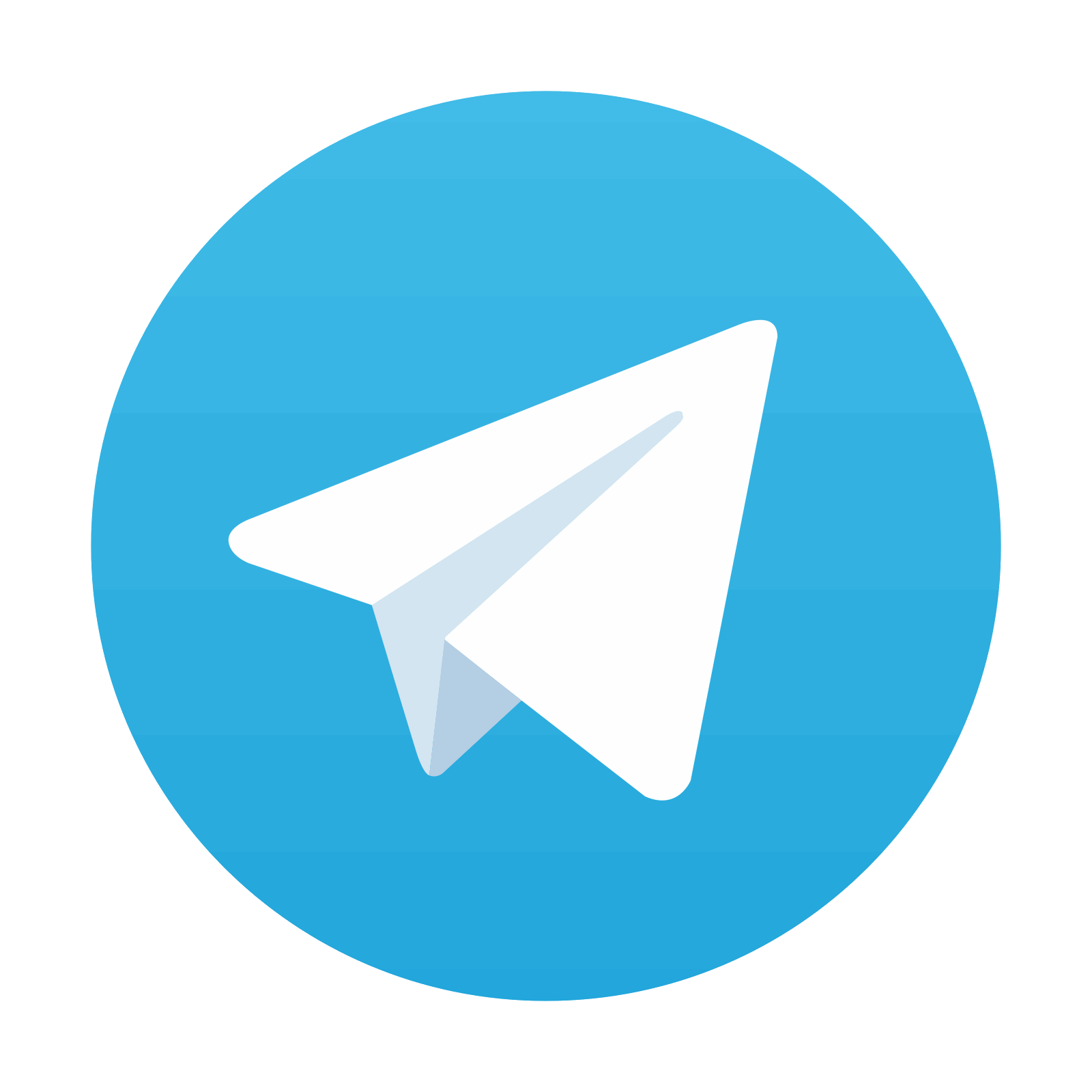
Stay updated, free articles. Join our Telegram channel

Full access? Get Clinical Tree
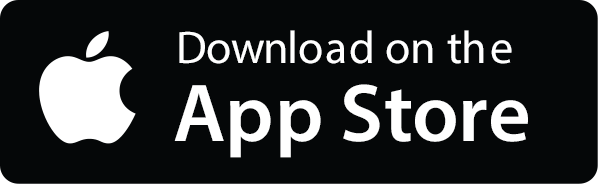
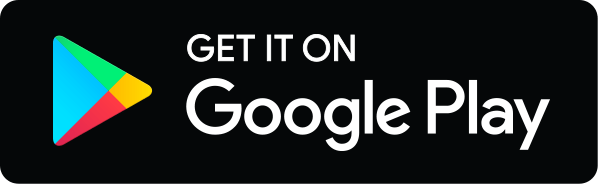