FIGURE 30.1 Postcontrast axial T1-weighted MRI. A large left frontal mass results in subfalcine herniation with shift of the ventricles and septum pellucidum to the right across midline (black line).
When there is downward transtentorial herniation from a supratentorial mass, the optic chiasm will be displaced toward the sella, the interpeduncular fossa will be compressed, the brainstem will appear buckled, and the splenium of the corpus callosum will lie below the plane of the incisura (Fig. 30.2B). There is resultant displacement of the midbrain and thalamus caudally.
When there is upward transtentorial herniation, usually from a cerebellar mass, the brainstem will be compressed against the clivus, the fourth ventricle will be compressed, and brainstem structures will become superiorly displaced with respect to the plane of the incisura.
On axial images, transtentorial herniation can be assessed by evaluating the circummesencephalic cisterns. With downward transtentorial herniation, the ambient cisterns and suprasellar cistern will become effaced (Fig. 30.3). When there is upward transtentorial herniation, the quadrigeminal plate cistern becomes effaced (Fig. 30.4).
Major complications of transtentorial herniation include vascular complications, hydrocephalus, and/or hemorrhage. Infarction in both the anterior and posterior circulation can occur from compression of major arteries against the dura. Hydrocephalus affecting the third and lateral ventricles can occur from obstruction of the aqueduct of Sylvius. A more portentous sign is the presence of midbrain and pontine hemorrhages referred to as Duret hemorrhages. These hemorrhages are typically the effect of reperfusion injury in vessels which were previously compressed and occluded by the mass effect.
Uncal Herniation
A specific subset of downward transtentorial herniation is herniation of the medial temporal lobe, specifically the uncus. This is usually the result of a medially directed vector from a middle cranial fossa lesion as opposed to the more downward-directed vector of traditional downward transtentorial herniation. Uncal herniation can be identified by displacement of the mesial portion of the temporal lobe, or the uncus, into the suprasellar cistern, causing effacement of the crural cistern.
As a result of its position medial to the uncus, the oculomotor nerve (cranial nerve III) may be compressed resulting in a fixed and dilated ipsilateral pupil. Although less common, compression of the posterior cerebral artery (PCA) and/or anterior choroidal arteries can also occur. As the mass effect increases, the contralateral cerebral peduncle can be compressed against the tentorium cerebelli causing a hemiparesis on the side ipsilateral to the lesion leading to a false localization of the abnormality. The indentation of the tentorium on the cerebral peduncle has been classically termed Kernohan notch and can occasionally be visualized on imaging.

FIGURE 30.2 A: Normal midline sagittal T1-weighted MRI. The plane of the incisura (white line) runs from the posterior sella to the junction of the vein of Galen and the straight sinus. B: Sagittal T1-weighted MRI. A large tectal mass resulting in hydrocephalus and downward transtentorial herniation. Here, a line drawn along the plane of the incisura intersects the splenium of the corpus callosum and passes above the interpeduncular fossa.

FIGURE 30.3 Axial CT image in a patient with supratentorial mass effect resulting in downward transtentorial herniation. There is effacement of the suprasellar and ambient cisterns (white arrow) with preservation of the quadrigeminal plate cistern (black arrow).

FIGURE 30.4 Axial CT image in a patient with posterior fossa mass effect resulting in upward transtentorial herniation. Notice the effacement of the quadrigeminal plate cistern (arrow).
Tonsillar Herniation
Herniation of the cerebellar tonsils through the foramen magnum typically occurs as a result of a posterior fossa mass. By imaging, the cerebellar tonsils are displaced caudally through the foramen magnum. This can result in fourth ventricular outlet obstruction and hydrocephalus.
BRAIN EDEMA
Brain edema is a secondary effect of many pathologic conditions of the CNS. Recognition of brain edema is critical as it may often be the only sign of an underlying disease and can be the fatal endpoint of a variety of conditions due to the associated mass effect.
Pathophysiology
The two main types of brain edema are vasogenic and cytotoxic. Cytotoxic edema is related to cellular swelling, which typically occurs with cell damage such as infarction. Vasogenic edema occurs with leakage of fluid into the extracellular space, and is a common finding associated with many lesions including neoplasms and infection. A third type of brain swelling is less commonly discussed, but has critical implications. Loss of the brain’s normal blood autoregulation, termed dysautoregulation, results in the rapid increase in intracerebral blood volume causing brain swelling and herniation. This syndrome is a frequent cause of death in head trauma.
The intracranial vascular compartment, unlike the brain itself, is compressible. Therefore, as there is increasing edema of any type within the confined space of the cranial vault, the intracranial pressure will also increase and have a detrimental effect on the vascular compartment. Normally, there is a reserve blood volume and autoregulation ensures continued adequate blood flow to the brain despite increased intracranial pressure. However, this only works up to a point. Brain death occurs once intracranial pressure exceeds the capacity for blood to flow to the brain.
Diagnosis
Cytotoxic edema is best detected with diffusion-weighted MRI. A distinguishing feature of cytotoxic edema is the involvement of gray matter resulting in loss of the normal gray–white differentiation. Identification of this characteristic is important as it limits the differential diagnosis. Cytotoxic edema localizing to the cortex is primarily related to vascular injury (i.e., arterial infarction), trauma, or infection. Infectious processes can result in cortical edema as a result of the infection itself (encephalitis) or from infection-related vasospasm causing secondary infarction. As opposed to cytotoxic edema, vasogenic edema typically affects white matter and spares the cortex leaving the gray–white differentiation intact. Unfortunately, the differential diagnosis remains broad in the setting of pure vasogenic edema as this is a common endpoint of the breakdown of the blood–brain barrier.

FIGURE 30.5 Axial noncontrast CT. There is diffuse brain edema with loss of normal gray–white differentiation, effacement of the sulci, and compression of the ventricles.
Brain dysautoregulation can be a difficult finding to appreciate on imaging. There is frequently no abnormal hypodensity, loss of gray–white junction, or other easily appreciable abnormality. The diffuse nature also means it is often symmetric in appearance making diagnosis all the more difficult. The secondary mass effect, including brain herniation or diffuse sulcal effacement, may be the only appreciable finding. The patients often have a profoundly abnormal Glasgow coma scale score despite the lack of readily apparent brain abnormality, such as hemorrhage.
All forms of brain edema typically result in secondary mass effect either in the region of edema or globally (Fig. 30.5). Once the increased intracranial pressure has resulted in sustained loss of blood flow, a nuclear medicine brain death study can be used to confirm the lack of intracranial blood flow; this study is often performed with Tc-99m diethylene triamine penta-acetate (DTPA) (Fig. 30.6). It is important to note that the brain death study will normalize within several days and therefore should be performed early when brain death is suspected.
INTRACRANIAL HEMORRHAGE
Early detection of acute intracranial hemorrhage can have a significant impact on patient outcomes. The hemorrhage itself can be a source of morbidity and mortality including localized brain damage, secondary mass effect, or hydrocephalus, to name a few. Additionally, identification of the source of hemorrhage may reveal a treatable cause which can decrease the incidence of subsequent hemorrhage.
Pathophysiology
Intracranial hemorrhage can result from a wide variety of causes, the majority of which are beyond the scope of this text. Some of the more common causes will be discussed here. As with all intracranial lesions, it is important to accurately localize hemorrhage on the imaging study, as this determines the most likely etiology and further workup and treatment. Moving from the outside in, this location can be extra-axial (i.e., epidural, subdural, or subarachnoid), intra-axial (i.e., involving the brain parenchyma itself), or intraventricular.
Diagnosis
Noncontrast CT is the study of choice in the evaluation of acute intracranial hemorrhage, as it is a rapid and accessible test, which produces high contrast between the high-attenuating (bright) clot and the low-attenuating (dark) CSF. MRI is also very sensitive for the detection of blood products, and the appearance of the blood on different sequences can be used to date the hemorrhage (1). Subarachnoid hemorrhage (SAH) is more commonly visualized on fluid-attenuated inversion recovery (FLAIR) images than conventional T1- and T2-weighted images since dilution of blood with CSF alters the normal evolution of blood products resulting in lack of the usual T1 and T2 signal characteristics. The FLAIR sequence is designed to suppress signal from the CSF so that it will appear dark; however, the presence of blood in the CSF results in incomplete suppression of CSF giving it a brighter appearance than normal (Fig. 30.7). However, this finding is not specific for hemorrhage and can also be seen from causes such as meningitis, neoplasm, propofol administration, or related simply to artifact. Susceptibility-weighted images (SWI) or gradient-recalled echo (GRE) sequences are also useful for the detection of blood products, as the hemoglobin affects the magnetic field in such a way as to decrease signal, the so-called susceptibility artifact. Thus, blood appears black on susceptibility images.

FIGURE 30.6 Tc-99m diethylene triamine penta-acetate brain death study. Projection images demonstrate no evidence of intracranial blood flow. There is increased activity over the nasal region (the “hot nose” sign commonly seen in brain death due to persistent external carotid arterial flow). Images from over the abdomen indicate perfusion of both kidneys, important information in a potential organ donor.

FIGURE 30.7 Axial fluid-attenuated inversion recovery (FLAIR) MRI. Subarachnoid blood is present as bright signal in the Sylvian fissures and sulci. A small amount of intraventricular hemorrhage is seen layering dependently in the left lateral ventricle.
Epidural Hematoma
An epidural hematoma occurs in the potential space between the inner table of the calvaria and the dura. It usually results from a skull fracture lacerating a meningeal artery, although it can occur as a result of venous injury from trauma or surgery. The arterial pressure is sufficient to separate the bone from the dura except at the sutures where the dura is very tightly adherent. This results in the classic biconvex shape of the hemorrhage, which is confined by suture lines (Fig. 30.8). An epidural hematoma is a surgical emergency as it can continue to expand and result in considerable mass effect, brain herniation, and death.
Subdural Hematoma
A subdural hematoma is located between the dura mater and the arachnoid, and usually results from tearing of bridging veins that course from the cortex to the dura. It is differentiated from an epidural hematoma in that it crosses sutures and has a crescent shape (Fig. 30.9). The etiology can be trauma, especially when there is rotational shear injury, but may also be secondary to a coagulopathy. Subdural hematomas are more common in elderly patients, where atrophy has resulted in a stretching of the bridging veins, predisposing them to injury.

FIGURE 30.8 Axial noncontrast CT. Large right epidural hematoma with significant mass effect resulting in subfalcine herniation. Note the classic biconvex shape as the blood is confined by the frontoparietal suture anteriorly and the parietooccipital suture posteriorly. There was an associated parietal bone fracture (not visualized on this image).

FIGURE 30.9 Axial noncontrast CT. Bilateral subdural hematomas, left greater than right. There is a fluid–fluid level on the left secondary to settling out of the blood products as the patient was in a prolonged supine position.
Subarachnoid Hemorrhage
SAH is located between the arachnoid and pia mater, and therefore is detected on imaging studies as blood filling the sulci and basilar cisterns (Fig. 30.10). Although noncontrast CT is the imaging study of choice, small volume or subacute SAH may not be detectable with CT, and therefore a lumbar puncture to look for xanthochromia may still be warranted. Once an SAH has been diagnosed, an investigation of its cause is necessary. The leading cause is aneurysmal rupture. Arterial venous malformation (AVM) is a less common etiology. The most appropriate initial imaging study to search for a vascular abnormality is CT angiography (CTA) (Fig. 30.11). If an underlying etiology cannot be identified, a conventional angiogram is warranted. If this also is negative, the patient should be reimaged in 1 week to look for possible recanalization of a thrombosed aneurysm. In 10% of the cases no etiology will be identified.
Patients with SAH need to be monitored for vasospasm. This typically first appears around day 3, peaks at day 7, and then begins to subside becoming uncommon after day 14. Vasospasm is detected on CTA as constriction of vessels, often with compensatory physiologic dilatation of the more distal vessels. CT perfusion imaging can aid in determination of the physiologic significance of underlying vasospasm. If a patient with vasospasm has compensatory blood flow through collaterals maintaining their brain perfusion, they often can be followed or treated with modified triple-H therapy (euvolemia, hypertension [HTN] [mean arterial pressure 90 to 110 mmHg], and hemodilution). A patient with vasospasm and decreased perfusion may need endovascular intervention, which can be performed with an intra-arterial calcium channel blocker such as verapamil or by angioplasty (Fig. 30.12).

FIGURE 30.10 Axial noncontrast CT. Diffuse subarachnoid blood products fill the anterior interhemispheric fissure and the basilar cisterns. This patient was found to have a ruptured anterior communicating artery aneurysm.

FIGURE 30.11 Volume surface–shaded rendering from a CT angiogram. There is a small aneurysm projecting anterosuperiorly at the origin of the middle cerebral artery on the right (arrow).
Intraparenchymal Hemorrhage
Intraparenchymal hemorrhage has many etiologies and can be divided into traumatic versus nontraumatic causes. Traumatic causes include blunt and penetrating injuries resulting in a contusion, or rotational forces resulting in shear injury and diffuse axonal injury (Fig. 30.13). Nontraumatic causes include HTN, amyloid angiopathy, hemorrhagic stroke, hemorrhagic tumor, coagulopathy, and venous obstruction. In the setting of nontraumatic injury, the underlying etiology may not be evident on CT or MRI and correlation with the clinical history is vital.
Parenchymal hemorrhage related to HTN usually occurs with an acute elevation of blood pressure in the background of chronic HTN. Chronic HTN produces small-vessel disease that leads to lipohyalinosis. This affects the penetrating arteries such as the lenticulostriates and thalamoperforators, and explains why hypertensive hemorrhage most commonly occurs in the basal ganglia and thalamus (Fig. 30.14).
Amyloid angiopathy is deposition of β-amyloid in the media and adventitia of small and midsized arteries of the leptomeninges and cortex. This leads to stenosis of the vessel lumen and weakening of the vessel wall, eventually resulting in the formation of microaneurysms. This predisposes patients to intraparenchymal—typically lobar—hemorrhages, which can be large and multiple. The most common locations are the frontal and parietal lobes.

FIGURE 30.12 A: Axial CT angiography image. Vasospasm affecting the left middle cerebral artery and left posterior cerebral artery following subarachnoid hemorrhage and aneurysm coiling. B: Frontal projection left internal carotid artery angiogram also showing vasospasm in the left middle cerebral artery. C: Frontal projection left internal carotid artery angiogram showing normal size of the left MCA following angioplasty for the vasospasm.
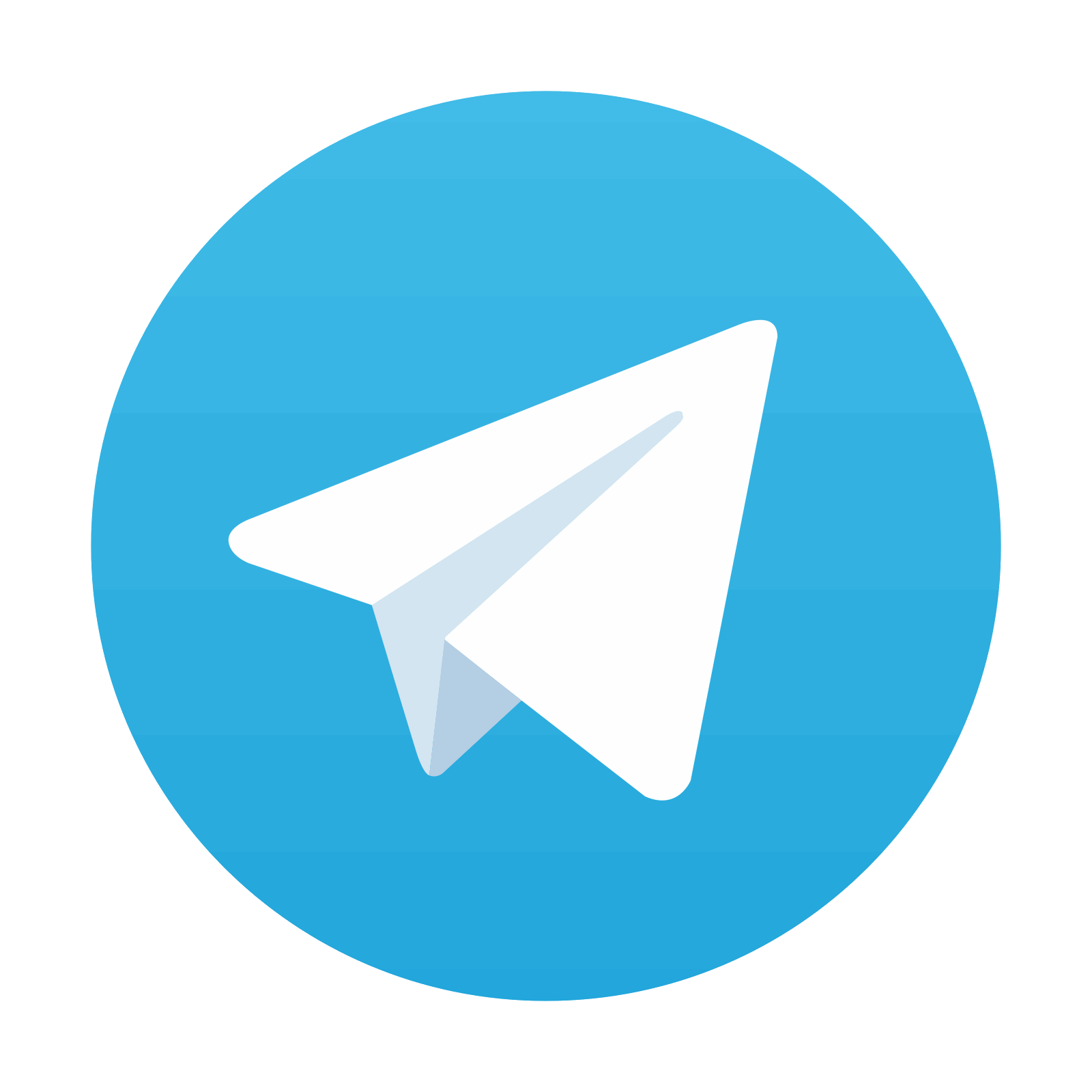
Stay updated, free articles. Join our Telegram channel

Full access? Get Clinical Tree
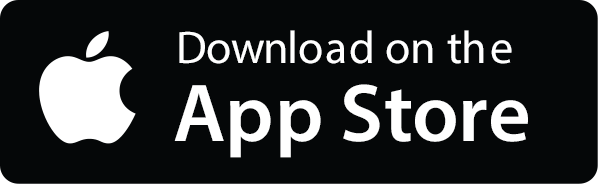
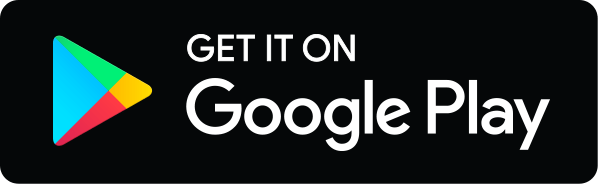