




![]() FIGURE 82.1. Bidirectional NEI communication. Stimulation of the acute stress response generates immunosuppressive signals (activation of the HPA axis, the SNS, and endogenous opioids) and immune-supportive signals (release of proinflammatory peptides, such as vasopressin and prolactin). A threat to the homeostatic milieu, in the form of trauma or infection, activates the immune system. Activated immune cells produce cytokines (e.g., TNF-α, IL-1, IL-6), which signal the CNS by stimulating afferent nerves or by circulating directly to the brain. Autonomic nerve activity delivers anti-inflammatory signals in response to immune activation. |

TABLE 82.1 PROINFLAMMATORY AND ANTI-INFLAMMATORY CYTOKINES | ||||||||||||||||||||||||||||||||||||||||||||||||||||||||||||||||
---|---|---|---|---|---|---|---|---|---|---|---|---|---|---|---|---|---|---|---|---|---|---|---|---|---|---|---|---|---|---|---|---|---|---|---|---|---|---|---|---|---|---|---|---|---|---|---|---|---|---|---|---|---|---|---|---|---|---|---|---|---|---|---|---|
|
as 36-72 hours impairs macrophage response to monocyte colony-stimulating factor in mice and affects phagocytosis and superoxide production ex vivo (20). In animal models, drugs that potentiate narcotic analgesia, such as clonidine and dexmedetomidine, also have immune suppressive effects (25).
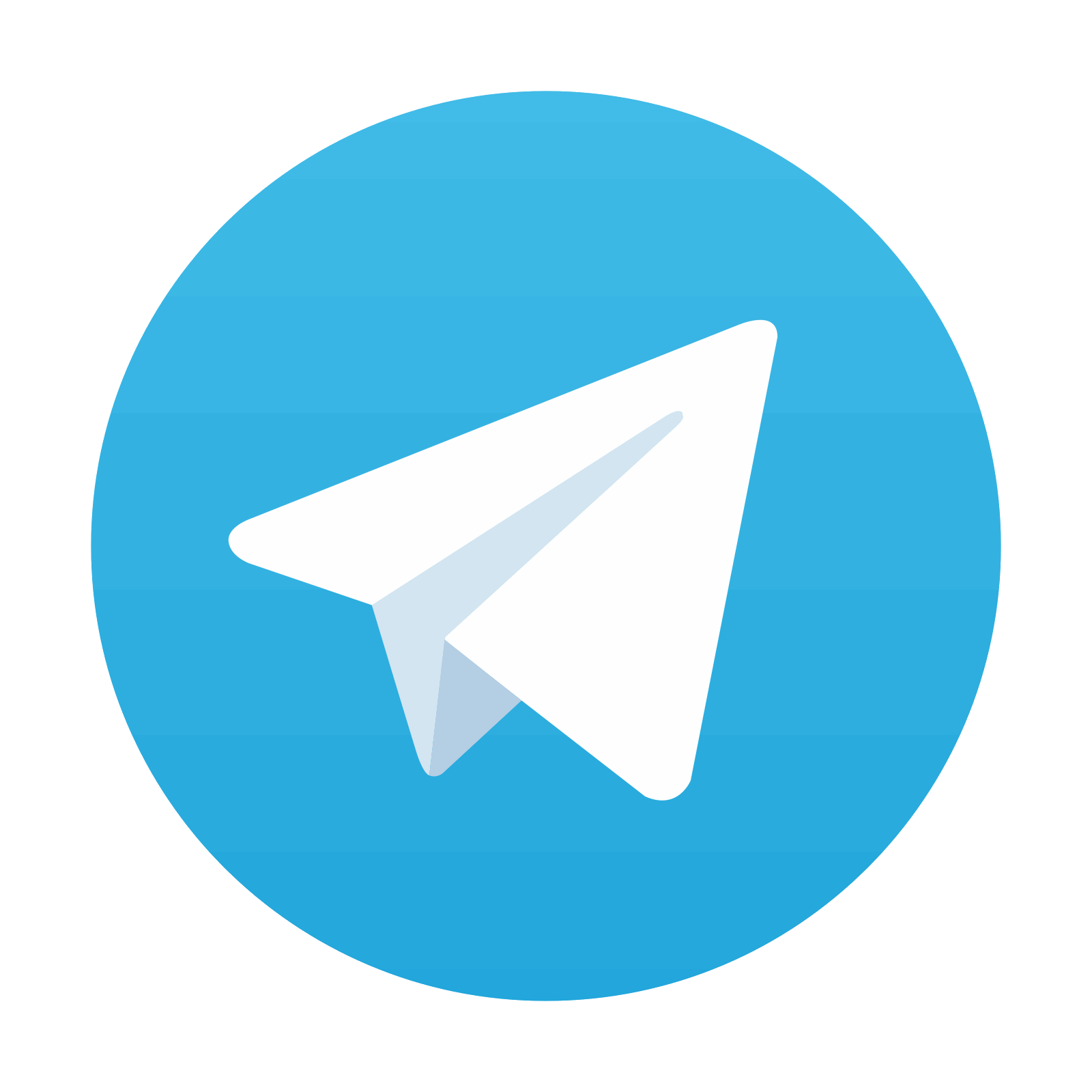
Stay updated, free articles. Join our Telegram channel

Full access? Get Clinical Tree
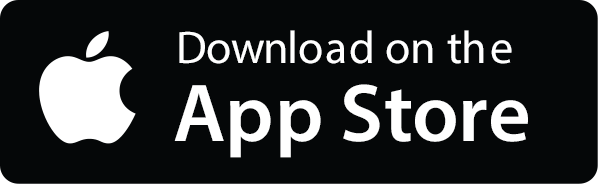
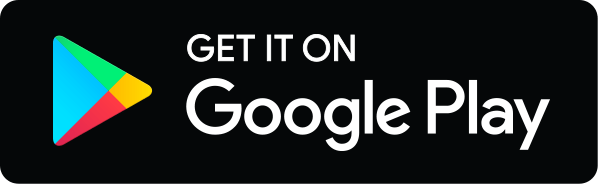
