Abstract
This chapter summarizes the key neurochemical mediators for activation and sensitization of primary afferent fibers at their distal endings in the tissues as well as the second messenger systems activated by these substances. Peripherally acting antihyperalgesic agents acting on distal nerve endings are then reviewed. In addition, the mediators for activation and sensitization of spinal dorsal horn neurons as well as the agents acting in the spinal cord to limit/modulate neurotransmission are reviewed. Finally, some of the spinal intracellular messenger chemical systems are reviewed.
Keywords
cytokine, glutamate, neuropeptide, nociceptor, prostaglandins, sensitization
Expertise in the neurochemistry of somatosensory processing provides clinicians the knowledge needed to control pain at the site of transduction of injury-related signals by nociceptors in the periphery and, secondly, by modification of signal transmission through the central nervous system.
Neurochemistry of Pain Transduction
Numerous chemicals are released in skin following tissue injury that either directly activate nociceptors or that increase their excitability. The graphical summary shown in Fig. 2.1 reveals that there are numerous mediators, frequently referred to simply as the “inflammatory soup.”

Inflammatory Soup: Several of the key “ingredients” of this soup include the following components.
Bradykinin, a potent vasodilating peptide, plays a critical role in inflammatory pain and hyperalgesia via actions on two G protein–coupled receptors (GPCRs): the constitutively expressed B2 receptor and the B1 receptor, expression of which is increased following tissue injury (see Petho and Reeh for reviews). Following injury, bradykinin is released by kininogens and produces acute pain in humans by activation of unmyelinated and myelinated nociceptors. Bradykinin also produces transient heat hyperalgesia in humans by sensitization of nociceptors through activation of phospholipase C (PLC), protein kinase C (PKC), the production of eicosanoids and nitric oxide (NO), and modulation of the transient receptor potential vanilloid 1 (TRPV1).
Low pH (excess free H + ) of inflamed tissue also contributes to the pain and hyperalgesia associated with inflammation. Low pH selectively causes activation and sensitization of nociceptors to mechanical stimuli by opening dorsal root ganglion neuron–specific acid-sensing ion channels (DRASIC/ASIC-3; see Dussor and Sluka and Gregory for reviews). Excitation of nociceptors by protons does not undergo tachyphylaxis or adaptation, and a synergistic excitatory effect of protons and a combination of inflammatory mediators has been reported.
Serotonin, which is released from platelets in response to platelet-activating factor derived from mast cell degranulation, leads to pain by directly activating nociceptors. In humans, direct application of serotonin to a blister base produced pain, and serotonin also potentiates bradykinin-induced pain and nociceptor activation.
Histamine is released from mast cells by substance P and calcitonin gene–related peptide (CGRP). These neuropeptides are derived from activated nociceptors and produce a variety of responses, including vasodilation and edema. Exogenous histamine applied to the skin produces itch but not pain when binding at the histamine 1 (H1) receptor. Nevertheless, histamine excites polymodal visceral nociceptors and potentiates the responses of nociceptors to bradykinin and heat. Histamine H3 and H4 receptors have been implicated in pain signaling. Both are Gi/o protein–coupled receptors found in various tissues. In the spinal cord, activation of these receptors attenuates the transmission of nociceptive information. Specifically, H3 receptor agonists have been proposed as potentially beneficial in treatment of neuropathic pain. H4 receptor activation was shown to decrease pain in a nerve injury model in mice. Further research to elucidate the site of action and drug targets is ongoing.
Eicosanoids are a large family of arachidonic acid metabolites that includes the prostaglandins, thromboxanes, and leukotrienes. Eicosanoids directly activate joint afferents and sensitize these, as well as those in skin and viscera, to natural stimuli and other endogenous chemicals (for reviews see Noguchi and Okubo and Chen et al. ). Prostaglandins, synthesized by the constitutive enzyme cyclo-oxygenase-1 (COX-1) and by the inducible enzyme cyclo-oxygenase-2 (COX-2), reduce the activation threshold of tetrodotoxin-resistant Na + currents in nociceptors, increase intracellular cyclic AMP (cAMP) levels, and increase the excitability of sensory neurons. Leukotrienes, metabolites of the lipoxygenase pathway, are released by macrophages and mast cells, contribute to hyperalgesia and sensitization to mechanical stimuli by acting on GPCR and by serving as chemoattractants for cytokine-producing cells, and result in further sensitization of primary afferents.
Oxidized linoleic acid metabolites are relatives of the eicosanoids, given that they derive from arachidonic acid and have also emerged as new peripheral mediators of pain sensitization. These contribute to inflammatory and thermal injury pain and interact with the sensitizing effects of nerve growth factor (NGF) at the TRPV1 receptor.
NO released by damaged afferents and acting on soluble guanylyl cylase (sGC) can further sensitize nearby neurons, augmenting pain and inflammation in both GPCR- and non-GPCR-mediated pathways. Direct injection of NO into the skin produces acute pain in humans, and mechanical sensitivity in animal models of neuropathic pain are decreased following administration of the NO synthase inhibitor N(G)-Nitro-L-arginine methyl ester (L-NAME). Further research on NO has uncovered a dual role: one as a mediator of nociceptive information and the other as a mediator of analgesia. NO has been shown to have analgesic properties both centrally and peripherally and may mediate the analgesic effects of drugs, such as opioids and nonsteroidal antiinflammatory drugs (NSAIDs).
Adenosine and its monophosphate and polyphosphate derivatives (AMP, ADP, adenosine triphosphate [ATP]) are increased in the extracellular space with tissue injury and inflammation (for review see Sawynok ). Like serotonin, adenosine induces pain in humans by direct activation of nociceptors. ATP also induces pain in humans and activates C-nociceptors in healthy human skin but does not sensitize C fibers to mechanical or heat stimuli. It is thought that ATP activates nociceptive neurons in normal skin via the purinergic receptors P2X 3 and the heteromeric P2X 2 /P2X 3 receptor (discussed later).
Cytokines (e.g., interleukin-1β [IL-1β], tumor necrosis factor α [TNFα], IL-6) are released by a variety of cells, such as macrophages, microglia, astrocytes, and Schwann cells, to regulate inflammatory cell responses (see Ji et al., Tiwari et al., and Clark et al. for reviews) but also modulate pain signaling. Cytokines can largely be classified as either proinflammatory or antiinflammatory, with those in the proinflammatory category more associated with different pain syndromes. Both IL-1β and TNFα directly excite and sensitize nociceptive afferent fibers to thermal and mechanical stimuli, and IL-6 in combination with its soluble IL-6 receptor also sensitizes nociceptors to heat. Clinical studies show that TNFα levels are increased in synovial fluid of painful joints and that treatment with antibodies against TNFα improves symptoms accompanying rheumatoid arthritis, including pain. Schwann cells express receptors for certain cytokines, such as TNF, interferon (IFN), IL-1, and IL-6. Activation of these receptors triggers a cascade of downstream reactions, including downregulation of myelin synthesis, increased expression of NGF receptors, dedifferentiation, and proliferation. The activated Schwann cells then begin to synthesize and release proinflammatory cytokines, affecting neighboring Schwann cells and thus closing a positive feedback loop that can exacerbate and sustain pain. IL-18, a member of the IL-1 family, plays a significant role in neuropathic pain; inhibition of IL-18 in the spinal cord also reduced bone cancer pain in rats. In contrast, the antiinflammatory cytokine IL-10 has shown antinociceptive properties in both neuropathic and inflammatory pain.
Furthermore, a subset of chemotactic cytokines, the chemokines, plays a role in the development of ongoing pain. Dorsal root ganglion (DRG) neurons coexpress chemokine and opioid receptors, and chemokine activation can enhance or prevent opioid-induced analgesia and even promote tolerance. Monocyte chemoattractant protein 1 (MCP1) and its receptor CCR2 are upregulated in primary afferent fibers and DRG cells following nerve injury, and injection of MCP1 in control animals creates a state of mechanical allodynia. In addition, mice lacking the CCR2 receptor are less susceptible to neuropathic pain.
Excitatory amino acid (EAA) receptors play a role in the modulation of nociception. EAA receptors are present on dorsal root ganglion cells and on the presynaptic terminals of primary afferents (see Miller et al. for review). Peripheral injection of glutamate activates nociceptors by binding to both ligand-gated ion channels (ionotropic glutamate receptors, iGluRs) and G protein–coupled metabotropic (mGlu) type 1 and 5 receptors (mGluR1 and mGluR5, respectively) on unmyelinated axons. Neurons in the DRG labeled for mGluR5 also express TRPV1 that are characteristic of nociceptive neurons.
NGF may contribute to inflammatory pain via direct and indirect mechanisms. Inflammatory mediators, such as cytokines, increase NGF production in inflamed tissues (see Mizumura and Murase and Lewin et al. for reviews). In turn, NGF stimulates mast cells to release histamine and serotonin, which can sensitize primary afferent fibers. Furthermore, NGF itself may directly sensitize nociceptors and can alter the distribution of Aδ fibers such that a greater proportion of fibers have nociceptor properties. Heat hyperalgesia can be induced by NGF acting directly on the peripheral terminals of primary afferent fibers. NGF is implicated in the inflammation-induced changes in nociceptor response properties, such as an increase in the incidence of ongoing activity, increase in maximum fiber frequency, and changes in the configuration of the action potential of DRG neurons. NGF-induced hyperalgesia may be mediated via its actions on the TTX resistant sodium channel Nav1.8 and by potentiating the responses of the VR1 receptor.
Proteinases, such as thrombin, trypsin, and tryptase, which are traditionally not considered part of the inflammatory soup, are gaining increasing attention as mediators of pain and inflammation for their actions on proteinase-activated receptors (PARs). There are four classes of PARs, with PAR1 and PAR2 being implicated strongly in pain and inflammation. Both receptor types are located in the periphery on nerve fiber endings. Activation of PAR1 via thrombin leads to the release of histamine, substance P, CGRP, and cytokines. Activation of PAR2 by trypsin and tryptase creates a cascade of inflammatory reactions, including prostaglandin and bradykinin release, which would further sensitize unmyelinated primary afferents. The net effect of activation of these receptors is increased sensitivity to both mechanical and thermal stimuli. Neutrophil elastase activates PAR2 and TRPV4, resulting in nociceptor hyperexcitability and inflammation.
Matrix metalloproteinases (MMPs) are a large family of endopeptidases that have only recently been found to contribute to pain. MMP-2, and possibly MMP-9, has been suggested to be related to diabetic neuropathy. MMPs serve as a macrophage chemoattractant and convert the cytokine TNFα into its active form. Following injury, microglia release MMPs, and at least one MMP, MMP-3, is upregulated in DRG cells. Use of the MMP-3 inhibitor minocycline protects against chemotherapy-induced hypersensitivity. Furthermore, research using other MMP antagonists has found decreased MMP-mediated degradation of myelin basic protein, decreased macrophage infiltration, and subsequent decreased mechanical sensitivity. At this time, the mechanisms and receptors involved in MMP-related pain induction have not been fully explored. Research regarding this family of proteins has made some progress and distinct roles for both MMP-9 and MMP-2 have been shown including: (1) transient MMP-9 upregulation after nerve injury in the early phase of development of neuropathic pain, (2) steady MMP-2 upregulation leading to maintenance of neuropathic pain, and (3) MMP-9/MMP-1-induced cleavage of IL-1β in the early and late phases of nerve injury, respectively. There is also some thought that upregulation of MMP-9 in the spinal cord is implicated in chronic opioid-induced withdrawal syndrome.
Peripheral AntiHyperalgesic Mechanisms: In contrast to the mediators discussed previously, there are also numerous mediators released into inflamed or injured tissue that act to limit pain transmission.
Opioids are also a component in inflammatory soup (see Stein for review). The peripheral terminals of afferent fibers contain receptors for opioids, but the number of receptors present is upregulated during inflammation. Furthermore, inflammatory cells such as macrophages, monocytes, and lymphocytes may be induced to increase the amount of endogenous opioids released by IL-1β and corticotropin-releasing hormone (CRH) originating from the inflamed tissue. Peripheral endogenous opioids may also be activated by endothelin-1 (ET-1), which is a potent vasoactive peptide, synthesized and released by epithelia after tissue injury. Paradoxically, ET-1 can trigger pain by activating ET A receptors on nociceptors or analgesia through its actions on ET B receptors. Activation of ET B receptors on keratinocytes by ET-1 results in release of β-endorphins and analgesia that is mediated via peripheral μ- and κ-opioid receptors that are linked to G protein–coupled inward rectifying potassium channels (GIRKs).
Acetylcholine (Ach) is released into injured tissue from nonneuronal sources and modulates pain via its effects on nicotinic or muscarinic receptors. Nonspecific nicotinic agonists have weak excitatory effects on C-nociceptors and induce a mild sensitization to heat but no alterations in mechanical responsiveness, but more recently selective agonist for the alpha 5 and 9 subunits have been reported to produce analgesia. In contrast, muscarinic agonists desensitizes C-nociceptors to mechanical and heat stimuli. Mice with targeted deletions of the M2 receptor show enhanced responsiveness of nociceptive fibers to noxious stimuli (see Fiorino and Garcia-Guzman for review), indicating a tonic inhibitory role for this mediator.
Gamma-aminobutyric acid (GABA) may have a peripheral role in pain transmission similar to the bimodal actions of Ach. GABA type A (GABA A ) receptors are located on unmyelinated primary afferents, and activation of these receptors by low doses of the agonist muscimol decreases pain, whereas high doses potentiate pain. GABA A receptors have also been found in DRG cells and on their central terminals in the dorsal horn, and direct application of GABA antagonists to DRG cells decreases hypersensitivity in an animal model of neuropathic pain.
Somatostatin (SST) is a peptide commonly associated with the gastrointestinal (GI) system that may also serve as an antinociceptive agent. Type 2a receptors (SSTR2a) are present in approximately 10% of unmyelinated primary afferent fibers innervating the glabrous skin of the rat, and intraplantar administration of an SST receptor agonist, octreotide, reduces the phase II response after formalin injection (see Malcangio for review). In addition, octreotide reduces the response of CMHs to heat stimuli and attenuates the thermal responses of nociceptors sensitized by bradykinin. SST also inhibits the release of cholecystokinin (CCK), which has been shown to have nociceptive properties. The peripheral effects of SST agonists may be mediated by a direct effect on primary afferents or by its antiinflammatory effects. The SSTR4 has also been implicated as a possible therapeutic target. Studies indicate that an SSTR4–δ-opioid receptor complex exists with varying functions. In neuronal cells, activation of this complex led to greater inhibition of cAMP/protein kinase A (PKA)-induced nociception.
Angiotensin II type 2 receptor (AT 2 R) is a relatively newly reported potential analgesic target (see Rice and Smith and Smith and Muralidharan for reviews). Angiotensin II is the primary effector in the renin-angiotensin system and is produced from angiotensin I in the lungs by angiotensin-converting enzyme, whereas angiotensin I is produced from angiotensinogen by the renal enzyme renin. Angiotensin II has well-known effects in the regulation of blood pressure acting at the type 1 receptor, whereas a physiologic role for the AT 2 R has remained elusive. The discovery of both angiotensin II and the AT 2 R in DRG neurons suggested a potential role in the modulation of sensory information and additional studies indicated an excitatory role on both peptidergic and larger DRG neurons. AT 2 R antagonists subsequently were shown to have antinociceptive properties in experimental animals, and this has subsequently been validated in a phase IIa proof-of-concept double-blind, placebo-controlled study in postherpetic neuralgia patients.
Peripheral Second Messenger Pathways: Inflammation is associated with the release of a host of chemical mediators. These agents may mediate pain by directly activating nociceptors, such as is primarily discussed previously. However, they may also produce more enduring changes in the sensory neuron, such as early posttranslational changes or even longer-lasting transcription-dependent changes in effector genes in DRG cells. The early posttranslational changes include phosphorylation of transducer molecules (e.g., TRPV1 receptor) and voltage-gated ion channels (e.g., sodium channels) in the peripheral terminals of nociceptors (peripheral sensitization). A classic example of these changes is seen in a VR, TRPV1 (also known as VR1). This receptor is present on a subpopulation of primary afferent fibers that are activated by capsaicin, heat, and protons. Inflammatory mediators, such as bradykinin and NGF, lower the threshold of TRPV1-mediated heat-induced currents in DRG neurons and increase the proportion of DRG cells that respond to capsaicin. These changes occur by PLC-dependent phosphorylation by PKC, by phosphorylation by PKA, and by hydrolysis of the membrane phospholipid, phosphatidylinosital-4-5-biphosphate (PIP 2 ). PKA and PKC also induce a short-term sensitization of nociceptors to heat by modulating the activity of tetrodotoxin-resistant sodium currents. In addition, increases in the activity of various transcription factors, including cAMP response element–binding protein (CREB) and the mitogen-activated protein kinase (MAPK), most especially the extracellular signal–regulated kinases (ERKs), the c-Jun amino-terminal kinases (JNKs), and the p38 enzymes produce even longer-term changes in TRPV1 following inflammation in primary afferent fibers. All of these signal cascades may be engaged by activation of Toll-like receptors on dorsal root ganglion neurons in mediating chemotherapy– and peripheral inflammation–related hyperalgesia.
Neurochemistry of Pain Transmission
As reviewed in the previous chapter, the anterolateral and dorsal column-medial lemniscal pathways transmit nociceptive signals from spinal cord to supraspinal sites, with differences between these paths being determined by anatomy and physiology of constituent neurons. However, unlike the differences in anatomy and physiology between the anterolateral and dorsal column-medial lemniscal systems, the neurochemistry of somatosensory processing in both is very similar. Both systems involve three classes of transmitter compounds, excitatory neurotransmitters, inhibitory neurotransmitters, and neuropeptides, that are found in three anatomic compartments: sensory afferent terminals, local circuit terminals, and descending (or ascending) modulatory circuit terminals. A graphical summary is found in Fig. 2.2 .

Excitatory Neurotransmitters: The amino acids glutamate and aspartate constitute the main excitatory neurotransmitters found at synapses throughout the somatosensory system. Thus transmission between primary afferent fibers and spinal neurons, between spinal neurons and thalamic neurons, and so on are dependent on the four receptor types for glutamate and aspartate in the somatosensory system. These receptors are named for the synthetic agonists that best activate them and include the N-methyl- d -aspartate (NMDA), the kainate, the AMPA ([R,S]-α-amino-3-hydroxy-5-methlyisoxazole-4-propionic acid) receptor and the metabotropic receptors. The latter three are often collectively referred to as the non-NMDA receptors. The AMPA and kainate receptors gate sodium channels and mediate the majority of the fast synaptic afferent signaling for all modalities and intensities of stimuli. The NMDA receptor is recruited only by intense and/or prolonged somatosensory stimuli that are sufficient to remove the magnesium ion that normally blocks the channel pore. Persistent activation of NMDA receptors leads to sensitization of dorsal horn neurons that includes an increase in receptive field size, decreased activation threshold, and prolonged depolarization. Multiple factors influence NMDA receptor-related sensitization. For instance, the release of bradykinin leads to increases in spinal glutamate released by astrocytes and neurons. This glutamate activates NMDA receptors, augmenting central sensitization.
In addition to the release of glutamate from neurons, activated glial cells can release glutamate. In certain pain conditions, such as chemotherapy-induced neuropathy, glial glutamate transporters GLAST and GLT-1 are downregulated, leading to decreased reuptake of spinal glutamate and subsequent spillover of glutamate to extrasynaptic receptor sites.
The metabotropic glutamate receptors (mGluRs) are a family of G protein–linked sites involved in more long-term cellular changes. The group I mGluRs when activated are coupled to G q/11 that activate PLC-liberating inositol phosphate, which in turn results in the release of cytosolic calcium and activation of PKC. The group II and III metabotropic receptors are negatively coupled by G i /G o to adenylyl cyclase and so reduce intracellular cyclic AMP and PKA activity. Given the complexity of these receptor transduction mechanisms, it should come as no surprise that activation of mGluRs can result in the modulation of multiple cellular kinases, receptors, ion channels, and transcription factors and so have complex and sometimes variable effects on somatosensory and pain processing. However, as a general rule the group I mGluRs have cooperative effects with NMDA receptors in promoting cellular excitability and signaling, whereas the group II and III mGluRs most often have inhibitory effects on transmission.
ATP also modulates somatosensory transmission. The primary receptor for ATP is the P2X family of receptors, which is composed of seven subunits expressed in six homomeric and at least four heteromeric subtypes. These receptors are present on the central terminals of primary afferent fibers innervating neurons in lamina V and II of the dorsal horn, where they function to increase the release of glutamate. The P2 class of receptors, both the ionotropic P2X and the GPCR P2Y classes, further play a unique role in glial-mediated pain sensitivity. The binding of ATP to P2 receptors on microglia changes the phenotype of these cells to include increased expression of P2 and cytokine receptors. These now-activated microglia begin to secrete inflammatory mediators, such as cytokines, NGF, and NO. These factors serve to sustain pain and inflammation. In support of these findings, researchers have found that mice lacking either P2X 4 or P2X 7 showed decreased sensitivity to mechanical and thermal stimulation in an animal model of pain. ATP may play a central role in pain perception in head and neck cancers as a result of expression of P2X 2 /P2X 3 on nerve fibers supplying these cancers.
Inhibitory Neurotransmitters: The amino acids glycine and GABA are the chief inhibitory neurotransmitters in the somatosensory system (for review see Prescott ). Glycine is the chief inhibitory amino acid at spinal levels, whereas GABA predominates at higher levels. There are two receptor sites for glycine, a chloride-linked strychnine-sensitive receptor and a strychnine-insensitive regulatory site on the NMDA glutamate receptors. GABA is found in local circuit neurons of spinal laminae I, II, and III. Three types of GABA receptors have been identified. The GABA A receptor is linked to a chloride channel and modulated by barbiturates, benzodiazepines, and alcohol. Selective GABA A agonists include muscimol, and selective antagonists include gabazine. A GABA A -mediated link between large myelinated fibers and C-fiber nociceptors has been proposed as a mechanism for the development of allodynia following intradermal injection of the irritant capsaicin. In addition, a selective loss of inhibitory interneurons at both spinal and thalamic levels has been suggested as contributing to some neuropathic pain conditions; and alternatively a shift in the chloride potential such that GABA A channels assume an excitatory function has also been proposed. The GABA B receptor has been associated with both a potassium ionophore and with a G protein–linked complex. Baclofen is a selective GABA B receptor agonist and phaclofen is a selective antagonist. Finally, the newly described GABA C receptor has also been described as associated with a potassium channel ionophore. Cis-4-aminocrotonic acid (CACA) is a selective agonist for this site, but there is currently no selective antagonist for GABA C receptors. GABA C receptors do not appear to have any role in the modulation of somatosensory information. Application of CACA has been shown to induce an antinociceptive effect that is blocked by 1,2,5,6–tetrahydropyridine-4-yl methylphosphinic acid (TPMPA). It was previously thought that these receptors did not appear to have any role in the modulation of somatosensory information, but this recent study indicates a potential role at this receptor site. Decreased spinal cord GABAergic inhibition is thought to contribute to neuropathic pain following peripheral nerve injury. Reports suggest that restoring spinal cord GABAergic signaling by intraspinal transplantation of cortical precursors of GABAergic interneurons can reverse neuropathic pain behavior in the mouse.
Norepinephrine is another abundant inhibitory neurotransmitter and is especially important in descending brainstem projections to the dorsal horn. The inhibitory effects of norepinephrine in the spinal cord appear to be twofold by directly activating inhibitory GABAergic interneurons and by also inhibiting excitatory interneurons. The adrenergic receptors include two broad classes termed the alpha and beta receptors, each of which in turn have several subtypes. The alpha2-adrenergic receptor is the primary form found in the spinal dorsal horn that has an inhibitory role on the processing of sensory information. However, it should be noted that the function of norepinephrine following injury to the nervous systems might reverse from an inhibitory, analgesic role into one of promoting and/or sustaining an ongoing chronic pain state.
Serotonin is also expressed in descending pathways to the spinal dorsal horn, predominately in those originating from the midbrain raphe nuclei. There are multiple serotonin (5-HT) receptor subtypes, including 5-HT-1, 2, and 3 receptors, and each of these major types also has several subtypes. Due to controversy concerning which of these subtypes mediates the analgesic properties of serotonin, interest in serotonin as a clinically useful target for the treatment of pain has weaned. In part, this controversy may be because some serotonin receptor subtypes in fact promote nociception, whereas others are inhibitory. If more selective tools are developed with which to dissect this pharmacology, serotonin may regain its former status as a potentially useful clinical target. Serotonin-norepinephrine reuptake inhibitors, such as duloxetine and amitryptiline, have been used to treat assorted pain conditions, with variable rates of success. The inhibitory and antinociceptive nature of norepinephrine and serotonin is further evidenced by the abundance of literature showing that many antidepressants that modulate both of these neurotransmitters, including duloxetine and amitriptyline, have analgesic properties in humans and in animal models of pain. Currently, it is thought that the antinociceptive effects are mediated by activation of α-1adenoreceptors and 5-HT2 receptors, leading to facilitation of descending inhibition.
Adenosine is another important inhibitory neurotransmitter at the spinal level. There are at least two types of adenosine receptors, termed the A1 and A2 sites. Occupation of these sites by adenosine results in G protein–mediated alterations of cAMP levels in target cells. However, both elevations and decreases in cAMP formation have been reported in various conditions. Adenosine may mediate a portion of the analgesia produced by brainstem norepinephrine projections to the spinal cord and appears to have especially robust analgesic properties in neuropathic pain conditions. Further studies have also shown that the adenosine A1 receptor (A1R) plays a role in transmission of pain signals. Activation of this receptor is believed to produce postsynaptic inhibition by activation of potassium (K + ) channels, thus causing hyperpolarization of the neuronal membrane. It has been demonstrated that mice that lack this A1 receptor have increased nociceptive responses to different pain stimuli.
Ach is yet another neurotransmitter that mediates antinociception at the level of the spinal dorsal horn. Stimulation of the vagus nerve results in inhibition of pain transmission, and it is likely that this effect is mediated by Ach. Ach may also contribute to the analgesia produced by the alpha2-adrenergic receptor agonist clonidine. It was previously thought that the antinociceptive effects of Ach were mediated by the muscarinic and not by the nicotinic Ach receptor subtypes. However, findings have shown that several nicotinic receptor subtypes play a role in a variety of pain states, their efficacy often limited by adverse effects. It was shown that coadministration of ABT-594 (an antiallodynic nicotinic Ach receptor agonist) with NS-9283 (a positive allosteric modulator of the α4β2 nicotinic Ach receptor subtype) resulted in potentiation of the analgesic effect without concomitant potentiation of adverse effects. Many other nicotinic receptor subtypes are being identified as potential therapeutic targets.
Neuropeptides: In addition to the excitatory and inhibitory neurotransmitters discussed previously, there are multiple neuropeptides that contribute to signaling of somatosensory information. Although some of these could be classified as excitatory compounds and others as inhibitory, we have separated these into a section of their own because of the distinct profile of action of these compounds as opposed to the neurotransmitters. Unlike the very rapid onset and termination of action of the transmitters, neuropeptides tend to have a more gradual onset and a more prolonged duration of action once released.
Substance P and neurokinin A serve as excitatory neuropeptides in the somatosensory system. The receptors for these peptides include the neurokinin 1 and 2 sites, each of which have been associated with elevation of intracellular calcium levels, perhaps through liberation of inositol phosphate. These two peptides may be present in intrinsic neurons of the spinal dorsal horn and thalamus but are especially concentrated in primary afferent fibers. At the spinal level, these peptides are only released following application of noxious stimuli that are sufficient to produce sustained discharges in C-nociceptors, although some small myelinated (Aδ) fibers may also contain substance P. Instead of signaling as synaptic transmitters, these peptides tend to spread throughout the dorsal horn, potentially acting on multiple synapses some distance from their point of release. It has been suggested that stimuli of particular modalities (e.g., mechanical vs. thermal) are associated with selective release of one peptide versus another; however, this suggestion has not been corroborated. Activation of neurokinin 1 and/or 2 receptors by substance P and/or neurokinin A is regarded as a key step needed for the induction of sensitization and hence the expression of hyperalgesia following cutaneous injury. It has been further proposed that the mechanism of neurokinin receptor involvement in the expression of sensitization is through facilitation of the synaptic actions of the EAA neurotransmitters.
CGRP, like substance P, is expressed predominately by small, unmyelinated primary afferent fibers, and it is also found in DRG cells and in the superficial layers of the spinal cord. Both CGRP and substance P synthesis and release are increased by another excitatory peptide, neuropeptide Y. Spinal release of CGRP has an excitatory effect on wide dynamic range neurons, and administration of the CGRP antagonist CGRP8-37 reverses this activity. Intrathecal administration of CGRP has been shown by some researchers to produce mechanical hypersensitivity, although it should be noted that others have failed to replicate this finding. Interestingly, the function of CGRP released within the brain seems to be antithetic to the peripheral and spinal effects, with release of this peptide within the PAG producing antinociceptive results.
CCK is a hormone peptide normally involved in digestion; however, it is also involved in the maintenance of pain. Some researchers contend that this effect is achieved via descending facilitation of nociceptive output from the rostral ventromedial medulla, whereas others propose that CCK blocks the descending antinociceptive effects of endogenous opioids within the periaqueductal grey. Coadministration of a CCK antagonist along with traditional exogenous opioids results in augmented analgesia and even opioid tolerance reversal. CCK also caused release of both prostaglandin E2 (PGE2) and 5-HT in the lumbar spinal cord that was attenuated by naproxen and ondansetron, respectively. Continued work is needed to better understand the mechanisms and therapeutic uses of CCK antagonists.
SST, the enkephalins, and possibly dynorphin are included as inhibitory neuropeptides at the spinal level. These peptides are contained in both intrinsic neurons of the dorsal horn and in the fibers descending to the dorsal horn from various brainstem nuclei. The endorphins are another class of inhibitory neuropeptides. The receptor types for the opioid peptides include the μ, δ, and κ receptor subtypes, and these receptors are found at all levels of the somatosensory system. These receptors are associated with modulation of both intracellular cAMP and potassium levels. There is also an important cooperative functional link between μ-opioid and alpha2-adrenergic receptors that have yet to be fully exploited for clinical applications.
Cannabinoids are present in the peripheral and central nervous systems and play a role in inhibiting pain. At this point, the CB1 receptor within the central nervous system seems to be a likely target for pharmacologic interventions. The CB1 receptor agonist Sativex is very effective at decreasing neuropathic pain but has sedative side effects. CB1 receptors are highly expressed in the DRG, and their activation at this level decreases the release of neurotransmitters involved in pain transmission and attenuates mechanical and heat hypersensitivity. Agarwal et al. showed that specific loss of CB1 receptors in peripheral nociceptive neurons leads to a major reduction in the antihyperalgesic effects of endocannabinoids and systemically administered cannabinoids. CT3 has decreased central nervous system bioavailability, and therefore fewer side effects, and yet is still efficient at producing analgesia. Lately, research has focused on a potential role of the CB2 receptor because activation of this receptor is associated with fewer adverse effects. Research has demonstrated that AM1710, an agonist at the CB2 receptor, decreased allodynia induced by paclitaxel in a mouse model. Furthermore, this same effect was not seen in mice in which the CB2 receptor was knocked out. A separate study showed that the CB2 agonist, JWH133, was able to attenuate pain responses in a model of osteoarthritis-associated pain.
Peroxisome proliferator-activated receptors (PPARs): The receptors discussed up to this point have been limited to those contained on the cell surface; however, the PPARs represent a class of nuclear receptors that serve as transcription factors. Within this family, three different isoforms exist (PPARα, PPARβ/δ, and PPARγ), which are localized to different tissues and serve different functions. Of these, PPARγ has been studied most extensively regarding the role it plays in attenuating inflammation. Rosiglitazone has been shown to possibly modulate neuropathic pain by acting on the PPARγ receptor to reduce expression of MCP-1 and its receptor, CCR2, in the DRG. Based on this line of research, it follows that PPARs may offer a novel means to decrease pain. As previously mentioned, future research attempting to use these receptor agonists as analgesics will have to overcome the serious side effects of increased adiposity and fluid retention. PPARs, such as PPARγ, are located in the brain and spinal cord. Although it is currently unclear how these receptors become activated following injury, once activated they mediate inflammatory substances, such as substance P, CGRP, and cytokines. In turn, mediation of these and other factors allows for inhibition of inflammation and pain.
Central Signal Propagation and Second Messenger Systems: The movement of various ions and the activity of cellular enzymes and metabolites are essential in the propagation of bioelectric signals in the central nervous system. Alterations to these factors can drastically reduce or augment signal propagation and ultimately somatosensory perception. Ion movement relies on proteins that form ion channels and function as second messenger enzymes. The actions of these proteins can be blocked by a number of agents, and many of these have been studied as putative analgesics. However, because ion channels and second messengers are found in all neural elements, the effects of compounds acting at these sites are not specific to pain circuitry. Side effects are therefore often encountered with these drugs, which limit their usefulness. There are four ion channels involved in pain signal propagation in the central nervous system, those for sodium, calcium, potassium, and chloride.
Sodium channels serve as the key to propagation of neural impulses throughout the nervous system because the opening of these channels is the primary event underlying the depolarization of nerve membranes, and sodium currents in dorsal horn neurons are mediated by at least three types of tetrodotoxin-sensitive channels. The local anesthetics lidocaine and bupivacaine physically block sodium channels, preventing the movement of sodium across the membrane. Prolonged infusions of local anesthetics for postoperative pain in humans became widespread in the 1990s, and cancer and chronic nonmalignant pain are treated with continuous infusions of intraspinal local anesthetics outside of the hospital. However, side effects are common and include delayed urinary retention, paresthesia, paresis/gait impairment, periods of orthostatic hypotension, bradypnea, and dyspnea. Advances in the understanding of sodium channel subtypes present novel means of achieving pain relief. Both the Nav1.7 and Nav1.8 subtypes are expressed throughout the central and peripheral nervous system and are critical in action potential generation in peripheral nociceptors. Clinically, patients with absent or nonfunctioning Nav1.7 channels experience congenital insensitivity to pain, and overactivity of this subtype is associated with certain chronic pain conditions. The anticonvulsant carbamazepine produces analgesia, presumably by inhibiting Nav1.7. Furthermore, mice lacking Nav1.8 display decreased pain responding, and the Nav1.8 selective blocker A-803467 alleviates neuropathic and inflammatory pain in animals. The Nav1.9 subtype is also involved in pain transmission. Evidence indicates a possibility that Nav1.9 plays a role in the development of pain secondary to trigeminal neuralgia. Each of these subtypes has been found to have genes that produce gain-of-function mutations leading to transmission of pain signals. As such, current research is focusing on genomics as a basis for possible therapeutic interventions (for reviews see Waxman and Moldovan et al. ).
Potassium channels are the second main cation channels of the neuronal action potential. There are four families of potassium channels, with the voltage-gated channels and the inwardly rectifying channels being strongly implicated to play a role in pain. Opening of voltage-gated potassium channels allows outward positive current flow from neurons, such as during repolarization following an action potential. Blockade of these channels initially prolongs generation of action potentials. However, continued application prevents repolarization and so ultimately produces a failure to generate action potentials. The inwardly rectifying channels establish and regulate the resting membrane potential. Evidence has implicated a potential for potassium channels to serve as targets for the treatment of pain. NO has been found to activate ATP-sensitive potassium channels (K[ATP]) and contribute to the maintenance of neuropathic pain, although the exact mechanisms of this effect are yet to be uncovered. Administration of the potassium channel blocker retigabine reversed surgically induced neuropathic pain in an animal model. Two-pore potassium (K2P) channels have emerged as potential therapeutic targets. This group of potassium channels has many different members. For instance, the TRESK and TREK subtypes have been shown to have a role in nociception. Studies have shown that mice in which the K2P was knocked out had silencing of heat nociceptors. Li and Toyoda also described several K2P channels, their role in pain signaling, and the potential for the development of new therapeutics.
Calcium ions are not directly involved in action potential propagation but instead are essential for the release of neurotransmitters following synaptic depolarization. At least four different types of calcium channels, the L-, N-, T-, and P-types, have been identified in dorsal horn neurons. There are numerous chemical antagonists of L-type calcium channels, whereas N-type calcium channels are blocked using toxins of Conus magus. P-type channels are especially prevalent in Purkinje cells and are sensitive to venom toxins of the funnel web spider (Agelenopsis aperta). T-type channels are involved in the regulation of neuronal excitability and pacemaker activity and are blocked by some omega conotoxins. Antinociceptive effects have been shown for N-, L-, T- and P-type calcium channels in animals and for L- and N-type channels in humans.
Chloride ions are also a major contributor to signal propagation, and three major classes of chloride channels have been identified. The first class identified was the ligand-gated chloride channels, including those of the GABAA and glycine receptors, and these are common in dorsal horn neurons. The second class, also likely common at spinal levels, is the voltage-gated chloride channel. The final chloride channel class is activated by cyclic adenosine monophosphate and may include only the cystic fibrosis transmembrane regulator. Activation of chloride currents usually results in hyperpolarization of neurons, and facilitation of these hyperpolarizing currents underlies the mechanisms of many depressant drugs. However, the GABAA receptors on primary afferent terminals gate a chloride channel that allows efflux, instead of the normal influx, of chloride with a net effect therefore of depolarizing primary afferent terminals. Chloride channel antagonists such as bicuculline and strychnine have not been given to relieve pain but instead to produce an experimental pain state characterized by a pronounced opiate-refractory allodynia. These compounds were also used to exacerbate the anatomic consequences of nerve constriction injury. A group of chloride channels known as calcium-activated chloride channels (CACCs) have been studied more recently. As their name implies, these channels are activated by increases in intracellular calcium, which then leads to an increase in chloride conductance. Pineda-Farias showed that anoctamin-1 and bestrophin-1, CACCs subtypes, may play a role in neuropathic pain. They specifically showed that anoctamin-1 mRNA was increased in rats that underwent L5/L6 spinal nerve ligation. Cho et al. also studied anoctamin-1 activity and demonstrated that it may function as a heat sensor, detecting thermal information and mediating nociceptive information; knockout of Ano-1 resulted in decreased nociception.
Finally, the role of second messenger systems on pain sensitivity has been examined in a number of studies. Increases in the levels of membrane-bound PKC have been found following both nerve injury and intraplantar injection of formalin. Spinal infusion of phorbol esters to activate PKC increases the behavioral response to intraplantar formalin and increases the spontaneous and evoked activity of primate spinothalamic tract neurons. In contrast, antagonists for PKC decrease pain behavior following nerve injury, intraplantar formalin, intraspinal NMDA, and intradermal capsaicin. Similarly, inhibition of PLC or phospholipase A (needed for release of cofactors to PKC) reduced hyperalgesia following intraplantar formalin and zymosan, respectively. Further evidence comes from the finding that animals engineered with defects in PKC had less pain following nerve injury, whereas those engineered with defects in PKA had decreased responses to formalin, capsaicin, and hind paw inflammation.
Based on this abundance of research, many second messenger systems could become targets for clinical pain treatment. However, the current role of these systems in pain management is indirect through the action of various drugs that interact with surface receptors linked to G proteins. Receptors linked to GS (receptors associated with βγα S subunits) include the beta1-adrenergic, dopaminergic type 1, and adenosine type 2 receptors. Those that activate Gq,12 (βγα q,12) include the serotonin 2c, alpha1-adrenergic, histamine, thromboxane A2, metabotropic glutamate, and the muscarinic type 1, 3, and 5 receptors. Finally, GI-(βγα i)-linked receptors include the adenosine 1, serotonin 1B, GABAB, muscarinic 2, μ-, δ- and κ-opioid receptors. Neurotransmitter receptors linked to GS and Gq,12 generally increase pain transmission, whereas GI-linked receptors inhibit pain signaling.
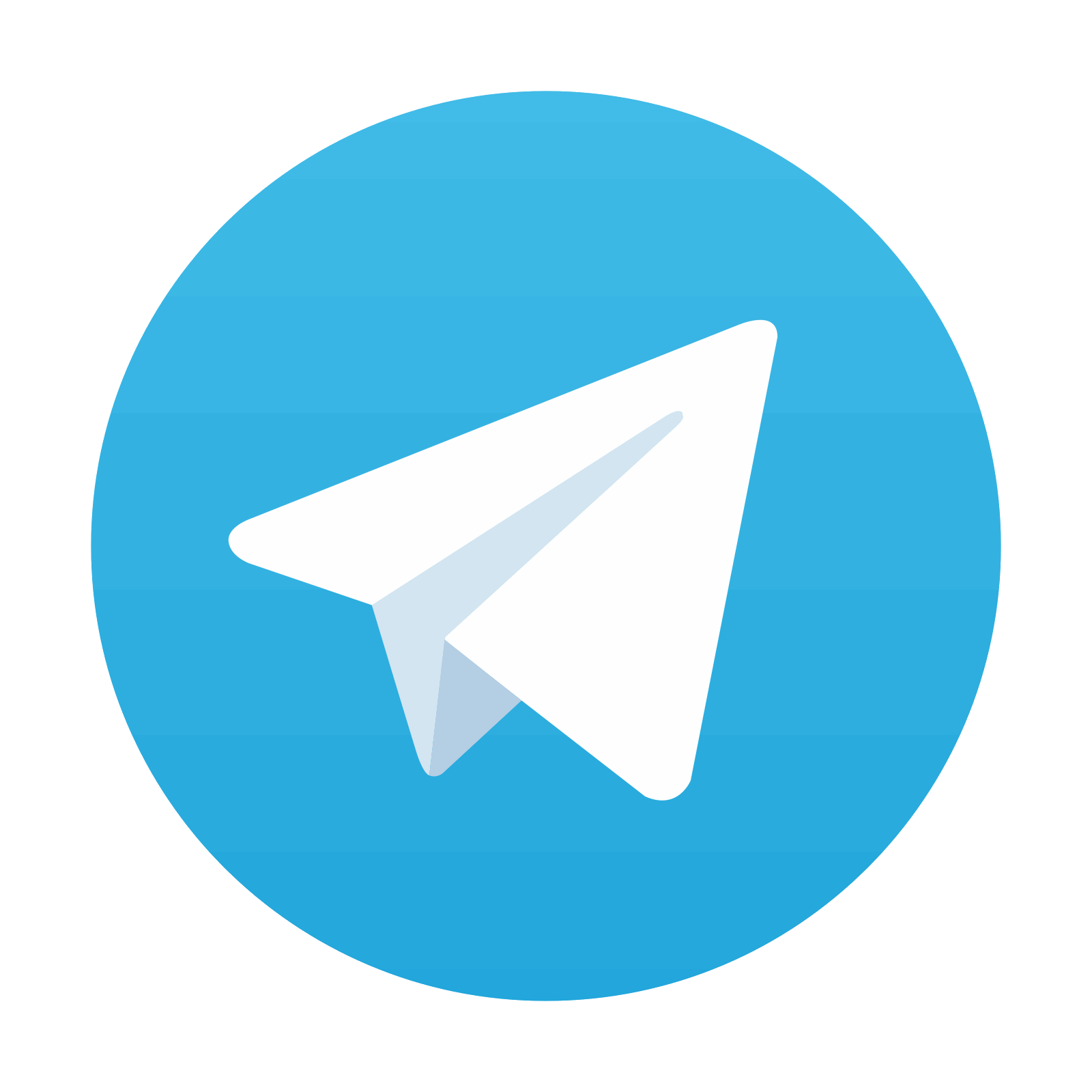
Stay updated, free articles. Join our Telegram channel

Full access? Get Clinical Tree
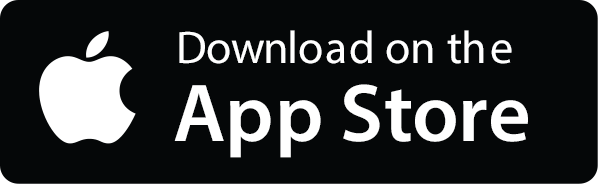
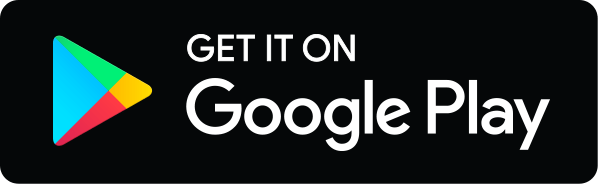