Neural Blockade of the Eye
Kathryn E. McGoldrick
Marianne E. Feitl
Orbital injection of local anesthetic was first performed in 1884, with cocaine, for enucleation of the globe (1). However, it was not until the 1930s, when the less toxic procaine and, later, the amide anesthetics became available, that orbital block was commonly used for ophthalmic surgery. Atkinson’s 1936 paper (2) popularized a technique that became firmly entrenched as the traditional retrobulbar block. Over the next five decades, anesthesiologists and ophthalmologists reported serious complications of retrobulbar blockade that were vision-threatening or life-threatening. Attempts to circumvent these complications led to the development in the mid-1980s of peribulbar block. However, peribulbar block is also associated with serious adverse sequelae. Advances in cataract surgery have enabled faster surgery with greater control and less trauma and allowed ophthalmologists to reexamine the use of topical anesthesia for this procedure, obviating, in many cases, the need for injection techniques.
Clearly, the requirements and complications of local anesthesia for intraocular and specialized extraocular surgical procedures are unique. A thorough understanding of the mechanism of neural blockade in ophthalmologic surgery is inextricably linked to detailed knowledge of the relevant anatomy. This chapter outlines the anatomy and techniques germane to the successful administration of local anesthesia for ophthalmic surgery. Moreover, in-depth discussion is presented of associated potential complications and their prevention.
Topical Anesthesia
Agents and Complications
Topical anesthesia frequently is used to eliminate corneal and conjunctival reflexes, in order to obtain ocular measurements. In addition, these agents are used for removal of superficial foreign bodies, suture removal, and irrigation of the lacrimal system. Cocaine is frequently administered during dacryocystorhinostomy to provide analgesia, vasoconstriction, and shrinkage of the nasal mucous membranes.
Although many agents that cause surface analgesia are available for topical use (Table 19-1), the most widely used agents are proparacaine and tetracaine. All agents have a rapid onset of action, within 30 to 60 seconds, and a duration of action from 10 to 20 minutes.
Ocular or serious systemic reactions to topical anesthetic agents are almost nonexistent, with the exception of cocaine. Cocaine penetrates the eye, where it blocks the reuptake of catecholamines at the nerve terminal and has a sympathetic potentiating effect. Pupillary dilation occurs.
Although 1 g of cocaine is considered to be the usual lethal dose for an adult, considerable variation occurs. The usual maximum dose of cocaine employed in clinical practice is 200 mg for a 70-kg adult, or 3 mg/kg. However, systemic reactions may occur with as little as 20 mg. Meticulous attention must be paid to the volume and concentration used because a narrow range spans safety to toxicity to death. Meyers (3) described two cases of cocaine toxicity during dacryocystorhinostomy, emphasizing that cocaine is contraindicated in hypertensive patients or in patients receiving adrenergic-modifying drugs such as guanethidine, tricyclic antidepressants, or monoamine oxidase inhibitors. Additionally, sympathomimetics, such as epinephrine hydrochloride or phenylephrine hydrochloride, should not be administered with cocaine.
Signs of cocaine toxicity can be evident in the respiratory, cardiovascular, and central nervous systems (CNS). Cocaine’s effect on the CNS is biphasic: Initial stimulation is followed by depression as inhibitory synapses are stimulated (4). The patient rapidly becomes excited, anxious, garrulous, and confused. Initially, reflexes are augmented. Headache is common. The pulse becomes rapid, and hypertension develops. Respiration becomes erratic. A chill may herald the sudden onset of hyperthermia. The pupils become dilated, and exophthalmos occurs. Nausea, vomiting, and abdominal pain are common. The patient may complain of something crawling on his or her skin. Delirium, Cheyne-Stokes breathing, convulsions, and, finally, unconsciousness ensues. Indeed, death may be very rapid following acute cocaine overdosage, and resuscitative maneuvers must be initiated quickly.
Before administering cocaine, the physician should thoroughly evaluate for possible contraindications, including the use of concurrent medications. To avoid toxic levels, dosages of dilute solutions should be calculated meticulously and administered carefully. Intravenous (IV) labetalol may be administered to treat serious cardiovascular complications (5). Initially, propranolol had been used to control cocaine-induced hypertension (6). However, a lethal hypertensive exacerbation has been ascribed to unopposed α-adrenergic stimulation (7). Thus, labetalol offers the advantage of both α- and β-blockade. To combat the CNS symptoms of cocaine toxicity, IV barbiturates should be given. Cooling measures may be required to treat hyperthermia, including a cooling blanket and ice water or alcohol sponging. In the event of cardiopulmonary arrest, the usual resuscitative measures must be attempted.
All of the topical agents can be toxic to the corneal epithelium with frequent repeated administrations and may delay the healing of corneal epithelial defects by inhibiting cell division
and migration (8). Therefore, topical anesthetics should be used for surgery or diagnostic tests and not for repeated symptomatic relief. Cocaine has the added disadvantage of causing loosening of the epithelium, which can result in large corneal erosions. Thus, cocaine has been replaced in many clinics by one of the alternatives shown in Table 19-1.
and migration (8). Therefore, topical anesthetics should be used for surgery or diagnostic tests and not for repeated symptomatic relief. Cocaine has the added disadvantage of causing loosening of the epithelium, which can result in large corneal erosions. Thus, cocaine has been replaced in many clinics by one of the alternatives shown in Table 19-1.
Table 19-1 Topical ophthalmologic anesthetic agents | ||||||||||||||||
---|---|---|---|---|---|---|---|---|---|---|---|---|---|---|---|---|
|
Topical Anesthesia for Cataract Surgery
Recent refinements in cataract surgery have been extremely impressive and have enabled the use of topical analgesia for these procedures. During the last few decades, the transition from intracapsular surgery to planned extracapsular and then phacoemulsification has enabled surgeons to have greater control of the intraocular environment. Small incisions, scleral tunnel incisions, sutureless wounds, and now clear corneal incisions have minimized surgical trauma and accelerated healing and visual recovery. Moreover, the implantation of intraocular lenses through small incisions became possible with the development of continuous circular capsulorrhexis and foldable implants.
Although Tomas Morena y Maiz of Peru first suggested the medical use of cocaine as a topical anesthetic in 1868, it was in 1884 that Koller and Freud in Vienna first instilled cocaine into the conjunctival sac for local ophthalmic analgesia. Then, in 1910, Julius Hirschberg reported on his extensive, favorable experience administering 2% cocaine chloride for topical anesthesia in cataract surgery (9). The advances in cataract surgery previously mentioned have allowed ophthalmologists to reexamine the use of topical anesthesia for this procedure. Phacoemulsification, with its small incisions, is the procedure of choice in using topical anesthesia. Although planned extracapsular procedures may be performed under topical anesthesia alone, it is frequently advisable to supplement with 1 to 2 mL of subconjunctival 1% lidocaine superiorly. If surgery is prolonged, topical analgesia may need to be supplemented with sub-Tenon’s lidocaine. This may be administered via a cannula for greater safety.
Topical anesthesia circumvents potential complications of peribulbar or retrobulbar block that can result in blindness or death. It also allows the patient to see well almost immediately after the surgery. Potential disadvantages of topical anesthesia include eye movement during surgery, patient anxiety, and (rarely) allergic reactions. Immediate allergic reactions are manifested by hyperemia, stinging, itching, and chemosis of the conjunctiva. Delayed hypersensitivity can occur as well. Proparacaine and especially cocaine have the greatest toxicity to the epithelium. Tetracaine, although less toxic, purportedly does not produce adequate deep anesthesia. Lidocaine has minimal epithelial toxicity and produces satisfactory deep analgesia of sufficient duration (8).
Patient selection is critical and should be restricted to individuals who are alert, able to follow instructions, and can control their eye movements. Patients who are demented, photophobic, or are unable to communicate are inappropriate candidates. Similarly, patients with small pupils, which may require significant iris manipulation, those requiring large scleral incisions, or those with an inflamed eye may be contraindicated for topical anesthesia.
In 1992, Charles Williamson developed a technique using topical 4% lidocaine for phacoemulsification surgery through a stepped clear corneal wound. Williamson described his technique, beginning with the instillation of two drops of 0.5% tetracaine into the operative eye prior to the application of dilating drops (10). (Tetracaine prevents the stinging associated with mydriatics.) Then, before the patient is brought to the operating room, two sets of topical 4% lidocaine, four drops every 5 to 10 minutes, are administered. After the patient is positioned on the operating room table, four more drops of topical 4% lidocaine are instilled. A well-dilated pupil is necessary to provide adequate visualization of the lens and to prevent the need for excessive iris manipulation. Orbital decompression devices are not used because they are uncomfortable and unnecessary; there is no solution in the orbit to cause external pressure. Minimal, if any, sedation is given because it is crucial that the patient be able to cooperate with the surgeon’s instructions. Some analgesic supplementation, however, is often required because topical application of local anesthetic in this setting does not provide the dense and profound analgesia associated with retrobulbar blockade (11,12). Moreover, supplementation of topical anesthesia with preservative free intraocular lidocaine may help to improve the comfort of both the patient and surgeon during cataract surgery using topical analgesia. After preoperative instillation of topical local anesthetic, preservative-free 1% lidocaine (0.3 mL) is injected into the anterior chamber after a corneal stab incision is performed. Viscoelastic is then instilled to fill the anterior chamber, and the remainder of the surgery is conducted using the standard technique.
Local Anesthetics and Adjuvants for Ophthalmologic Neural Blockade
Agents injected for regional anesthesia in ophthalmic surgery (Table 19-2) are the same as those used in other peripheral nerve blocks (see Chapter 4). Lidocaine was traditionally the commonly used agent. In the last two decades, however, there has been a tendency to use local anesthetics of longer duration, such as bupivacaine, that reduce the need for postoperative analgesics and minimize eye movements immediately after surgery. These agents can be used alone or in combination with lidocaine, to take advantage of the rapid onset of lidocaine and the long duration of bupivacaine. In addition, the longer-duration agents can provide adequate anesthesia for the more lengthy and complex procedures such as combined vitrectomy and retinal reattachment surgery.
Epinephrine frequently is added to the injection solution for ophthalmologic neural blockade to counteract the vasodilator action of the anesthetic agent and to reduce bleeding. Epinephrine can also serve as a marker of accidental IV
injection and to facilitate augmentation and longer duration of action of lidocaine, mepivacaine, or bupivacaine. Dilute concentrations of 1:200,000 (1 mg/200 mL) should be used to avoid tissue injury secondary to ischemia. The use in the orbit of premixed anesthetic solutions with epinephrine is discouraged, because of their high concentration of metabisulfite, an allergenic and neurotoxic substance. Rather, the addition of 0.1 mL of 1:1,000 epinephrine at time of use to 20 mL ampules or vials, or 0.15 mL of 1:1,000 to 30 mL vials, to produce 1:200,000 solutions is recommended. Importantly, retrobulbar epinephrine may reduce blood flow to the optic nerve and is best avoided in patients with glaucomatous optic nerve damage.
injection and to facilitate augmentation and longer duration of action of lidocaine, mepivacaine, or bupivacaine. Dilute concentrations of 1:200,000 (1 mg/200 mL) should be used to avoid tissue injury secondary to ischemia. The use in the orbit of premixed anesthetic solutions with epinephrine is discouraged, because of their high concentration of metabisulfite, an allergenic and neurotoxic substance. Rather, the addition of 0.1 mL of 1:1,000 epinephrine at time of use to 20 mL ampules or vials, or 0.15 mL of 1:1,000 to 30 mL vials, to produce 1:200,000 solutions is recommended. Importantly, retrobulbar epinephrine may reduce blood flow to the optic nerve and is best avoided in patients with glaucomatous optic nerve damage.
Table 19-2 Local anesthetic agents used in ophthalmology | ||||||||||||||||||||||||||||||
---|---|---|---|---|---|---|---|---|---|---|---|---|---|---|---|---|---|---|---|---|---|---|---|---|---|---|---|---|---|---|
|
The enzyme hyaluronidase is often added to the anesthetic solution to enhance solution diffusion through the tissues; this action is accomplished by hydrolysis of extracellular hyaluronic acid. For ophthalmic solutions, 7.5 to 15 turbidity-reducing units (TRU) per mL are used. The addition of hyaluronidase allows a more complete, reliable block with the use of less anesthetic solution, and thus less tissue distortion. Additionally, improved dispersal of the anesthetic agent may reduce the incidence of myotoxicity and subsequent postoperative diplopia.
The myotoxic effects of local anesthetics on muscle fibers of humans and animals have been well-established (13,14,15). Rainin and Carlson (16) reported three cases of permanent and one case of temporary vertical muscle paresis after injection of 0.75% bupivacaine directly into the extraocular muscles of four patients. Subsequently, Carlson and associates (17) showed that only 1 mL of 0.75% bupivacaine, 2% lidocaine, or 2% mepivacaine, injected retrobulbarly into rhesus monkeys, led to damage of the extraocular muscles closest to the site of injection, usually the inferior recti and oblique muscles. The same substances injected into human extraocular muscles caused massive lesions, which often occupied the bulk of the cross-section of the muscle. Within such lesions, all of the muscle fibers were uniformly destroyed (17). Lidocaine appears preferentially to destroy white fibers, whereas bupivacaine is more toxic to red fibers. However, at higher concentrations of local anesthetics, this specificity is abolished, and all striated muscle is injured. Interestingly, other tissues such as smooth muscle, neurons, and connective tissue are unaffected by the same concentration of local anesthetic.
Clearly, intramuscular injection of local anesthetics above a threshold concentration (18) causes myonecrosis with loss of myotubules. Damaged fibers become hyalinized and are invaded by macrophages. However, cell nuclei appear to be spared. Regeneration (16,19) can begin after several days and is often complete in 6 weeks if the microcirculation of the nerve and muscle is intact. In addition to being concentration-dependent, myotoxicity is also dependent on the volume of muscle into which the local anesthetic is injected and on limited epimysial or perimysial spread. Because damage is more likely to be irreversible if the blood supply to the muscle is damaged, injection into the extraocular muscles in the area of the hilum should be assiduously avoided. Early recognition of myotoxicity and selective patching of the eye during regeneration are important.
Ptosis following cataract surgery is not uncommon, and multiple factors have been implicated in its etiology (20,21). These include the presence of a preexisting ptosis, injection of anesthetic solution into the upper lid when performing facial nerve block, retrobulbar injection, injection of peribulbar anesthesia through the upper eyelid at the 12-o’clock position, ocular compression or massage, the eyelid speculum, placement of a superior rectus bridle suture with traction on the superior rectus-levator complex, creation of a large conjunctival flap, prolonged or tight patching in the postoperative period, and postoperative eyelid edema. Direct intramuscular injection of local anesthetics may also account for many cases of transient and permanent ptosis (16). A recent magnetic resonance imaging (MRI) study performed immediately after the diagnosis of diplopia in four patients who received peribulbar blockade disclosed inflammatory edema of the paralyzed extraocular muscle consistent with direct local anesthetic–induced myotoxicity after presumed inadvertent intramuscular injection (22).
Feibel and colleagues (20) believe that the development of postcataract ptosis is multifaceted and that no single aspect of cataract surgery is the sole contributor. That the local anesthetic injection cannot be isolated as a primary factor is underscored by the observation that postsurgical ptosis is seen in patients undergoing surgery with general anesthesia.
Anatomy of the Orbit and the Eye
A thorough knowledge of the anatomy of the orbit and eye (23), especially of the innervation of the orbital structures, is essential to obtain effective neural blockade. Anatomy related to specific anesthetic blocks is detailed under the description of the regional block.
Orbit
The bony orbit has the shape of a pear with the stem directed toward the optic canal (Fig. 19-1). The orbit is covered by the outer periosteal layer of the dura mater (periorbita). The orbit contains the muscles, nerves, and vessels that enable the eye to function properly. A number of blood vessels and nerves
supplying areas of the face around the orbital aperture pass through the orbit. Important surrounding anatomic structures include the anterior cranial fossa above, the maxillary sinus below, and the nasal cavity and ethmoidal air cells medially. There are nine canals and fissures in the orbit, the most important being the optic foramen, the superior and inferior orbital fissures, and the supraorbital and infraorbital foramina (Fig. 19-2).
supplying areas of the face around the orbital aperture pass through the orbit. Important surrounding anatomic structures include the anterior cranial fossa above, the maxillary sinus below, and the nasal cavity and ethmoidal air cells medially. There are nine canals and fissures in the orbit, the most important being the optic foramen, the superior and inferior orbital fissures, and the supraorbital and infraorbital foramina (Fig. 19-2).
Eye
The normal globe is about 24 mm in the anteroposterior diameter. This dimension is increased in myopic (nearsighted) eyes. The eyeball has three concentric layers: an outer fibrous layer (the cornea and sclera), a middle vascular layer (the iris, ciliary body, and choroid), and an inner neural layer (the retina). Intraocular contents are: aqueous humor in the anterior chamber
(space lined by the cornea, iris, and pupil) and in the posterior chamber (space behind the iris and in front of the vitreous), the crystalline lens, and the vitreous humor (space behind the lens and in front of the retina).
(space lined by the cornea, iris, and pupil) and in the posterior chamber (space behind the iris and in front of the vitreous), the crystalline lens, and the vitreous humor (space behind the lens and in front of the retina).
The optic nerve (cranial nerve II) pierces the globe just above and 3 mm medial to the posterior pole. The nerve has a diameter of 1.5 mm and an intraorbital length of about 30 mm. The nerve exits the orbit by way of the optic foramen. The traditional upward and inward position of the globe during retrobulbar anesthesia (see following) results in stretching, as well as a downward and outward displacement of the optic nerve. This position places the nerve in a vulnerable position for direct traumatization (24,25). Thus, having the patient look in primary gaze should reduce the risk of direct injury.
Six extraocular striated muscles control the movements of the globe. The four rectus muscles (superior, medial, inferior, and lateral) originate from a common tendon ring that encircles the optic foramen (annulus of Zinn) with their tendinous insertion 5.5 to 7.5 mm from the limbus of the cornea. The superior oblique muscle originates above and medial to the optic foramen, runs medially to the trochlea, then bends backward to insert on the globe beneath the superior rectus muscle. The inferior oblique muscle originates medially from the periosteum of the lacrimal bone, runs beneath the inferior rectus muscle, and inserts on the posterolateral aspect of the globe. The seventh striated muscle in the orbit is the levator of the upper eyelid, which originates from the periosteum of the apex of the orbit above the superior oblique muscle. The levator runs forward between the roof of the orbit and superior rectus muscle and spreads out into an aponeurosis that inserts into the skin and tarsal plate of the upper eyelid. Cranial nerves III, IV, and VI innervate these striated muscles (Table 19-3 and Fig. 19-3A,B).
![]() Figure 19-3. A: Orbital anatomy as seen from the lateral approach. B: Orbital anatomy as seen from above. |
Table 19-3 Motor innervation to ocular adnexal muscles | ||||||||||||||
---|---|---|---|---|---|---|---|---|---|---|---|---|---|---|
|
Innervation of the Eye and Orbital Contents
The sensory supply to the eye and its adnexa is derived from the trigeminal nerve (cranial nerve V). This is a mixed nerve
that comprises a large sensory part and a small motor part. The sensory portion of the nerve divides at the trigeminal ganglion into three branches: ophthalmic, maxillary, and mandibular (Table 19-4, Figs. 19-3 and 19-4).
that comprises a large sensory part and a small motor part. The sensory portion of the nerve divides at the trigeminal ganglion into three branches: ophthalmic, maxillary, and mandibular (Table 19-4, Figs. 19-3 and 19-4).
The motor supply to the extraocular muscles and levator palpebrae muscle of the upper eyelid is provided by cranial nerves III, IV, and VI (Table 19-3). The facial nerve (cranial nerve VII) supplies all the muscles of expression, including the orbicularis oculi in the eyelids. The nerve emerges through the stylomastoid foramen, just below the osseous part of the outer ear. The facial nerve then turns forward and enters the parotid gland superficial to the neck of the mandible, where it divides into five branches: temporal, zygomatic, buccal, mandibular, and cervical. The temporal division supplies the upper part of the orbicularis oculi, the corrugator supercilii, and the frontalis muscle. The zygomatic branch supplies the lower part of the orbicularis oculi muscle (Fig. 19-5).
The autonomic nervous system supplies the eye through the sympathetic and parasympathetic systems (Table 19-5, Fig. 19-4).
Table 19-4 Trigeminal (V cranial nerve) sensory innervation of the eye and adnexal structures | ||||||||||||||||||||||||||||||||||||||||
---|---|---|---|---|---|---|---|---|---|---|---|---|---|---|---|---|---|---|---|---|---|---|---|---|---|---|---|---|---|---|---|---|---|---|---|---|---|---|---|---|
|
Akinesia of the Orbicularis Oculi Muscle
Akinesia of the eyelids is important for intraocular surgery to prevent compression of the lids and expulsion of intraocular contents. Paralysis of the orbicularis oculi muscle may be achieved either by local infiltration of the muscle or proximal infiltration of the branches of the facial nerve that supply it. Although a separate block to provide akinesia of the orbicularis oculi muscle is typically performed in conjunction with retrobulbar blockade, this additional maneuver is unnecessary with peribulbar blockade owing to diffusion of the local anesthetic to the targeted area.
Van Lint Method
In 1914, van Lint was the first to describe akinesia of the orbicularis oculi for cataract extraction (26). The classic van Lint technique involves inserting the needle at the lateral orbital rim and making a small intradermal wheal. The needle is advanced into the deep tissues along the inferolateral orbital margin. As the needle is withdrawn, 2 to 4 mL of anesthetic is injected. The needle is then redirected along the supertemporal orbital margin. Again, anesthetic is injected as the needle is withdrawn. Pressure is applied to the eye to promote diffusion of the anesthetic. This method has the side effect of producing lid edema. Therefore, the technique has been modified by placing the injections more laterally to block the facial nerve as it crosses the periosteum of the orbital rim, and is followed by inferior and superior injections (Fig. 19-6).
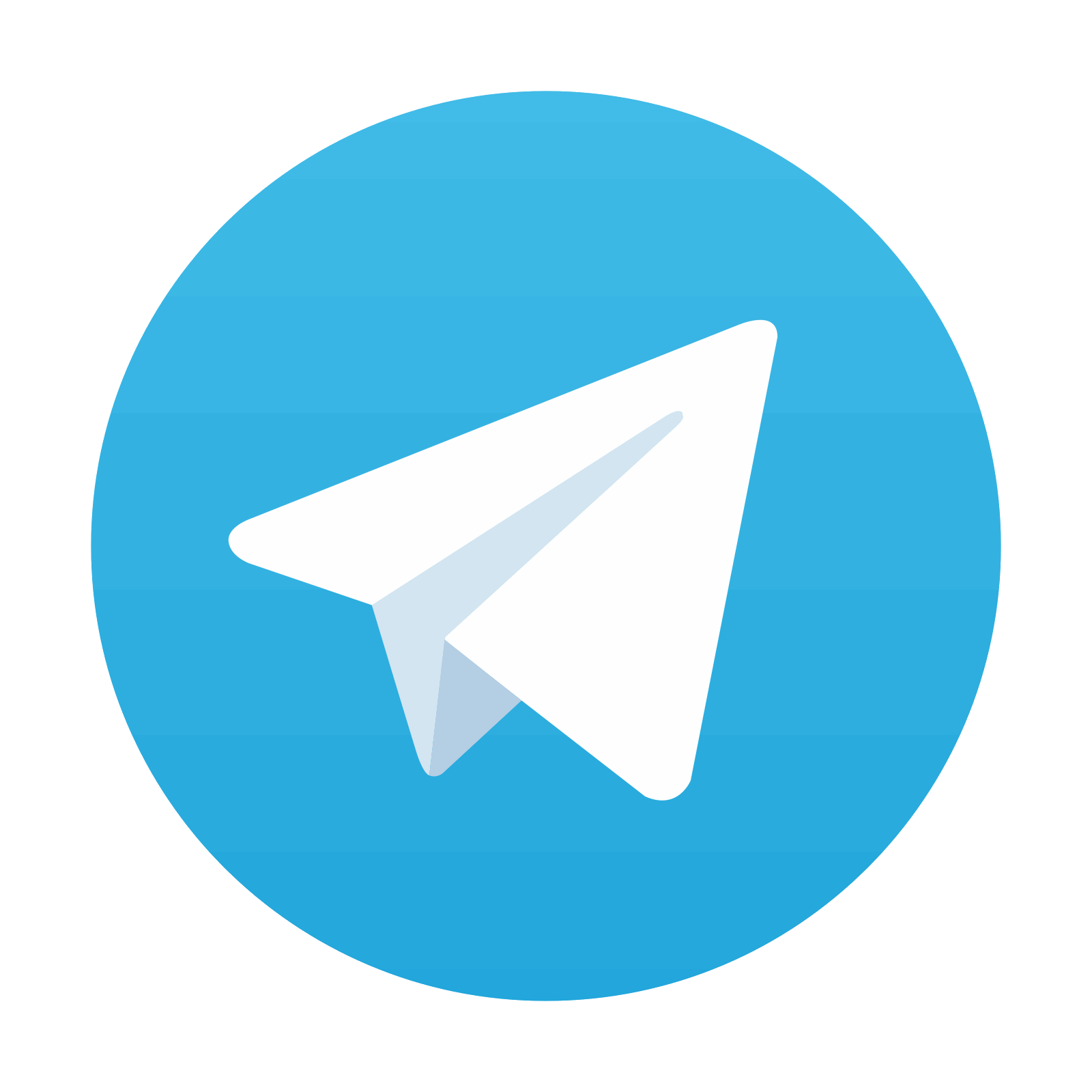
Stay updated, free articles. Join our Telegram channel

Full access? Get Clinical Tree
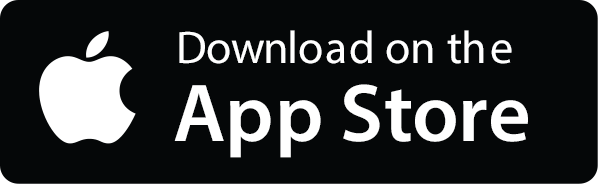
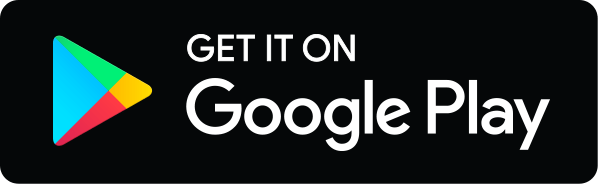