Neural Blockade for Pediatric Surgery
Bernard J. Dalens
René Truchon
Regional anesthetic techniques have become major tools in the management of surgical and nonsurgical pain in pediatric patients. During the last decade, many improvements have occurred, especially regarding technologic aspects such as nerve location, devices specifically designed for pediatric use, and availability of less toxic local anesthetics, but also in terms of the conceptualization of pain management. Currently, ultrasonography is revolutionizing the whole field of regional anesthesia, as nerve stimulation did a few decades ago.
An abundant literature has extensively evaluated the techniques, their indications, the benefits and adverse effects to be expected, and the way to avoid complications. Some anesthesiologists, including pediatric anesthesiologists, are still reluctant to use regional techniques in children, especially in infants, or—worse—they are prone to misuse them. For example, caudal anesthesia is still overused, ignoring the fact that it is a neuraxial block—a major anesthetic procedure, the use of which should be restricted to major surgery only. In this chapter, we summarize the specific factors that differentiate between neonates, infants, children, and adults, and then consider the different block procedures suitable to the pediatric population, focusing on safety aspects. Some indications or therapeutic strategies remain controversial, such as the potential dangers of combined regional and general anesthesia, advantages and hazards of adjuvants, and the risk of masking symptoms of compartment syndromes. These issues will be addressed and, although they will remain controversial, a logical and scientifically founded approach to the individual situations will be proposed, taking also into consideration the relevant medicolegal precautions.
General Considerations
Differences in Anatomy Between Children and Adults
Overall Influence of Growth Process
The body of a child must be seen as a developing structure that is not anatomically and physiologically completed before the end of puberty and even in the early years of adulthood. The most evident differences pertain to the changes in size, from a length of about 50 cm and a weight of 3 to 3.5 kg at birth to more than 150 cm and 50 kg (often significantly more) at the end of the pediatric period. These considerable increases in size are neither continuous nor proportional; the relative growth of the trunk and limbs is greater than that of the head. This has marked implications in regional anesthesia because the anatomic landmarks, especially surface landmarks, are constantly changing and must be precisely identified before any block procedure is undertaken. Ultrasound technique may help considerably in locating bony structures and nerve paths (1,2). The use of surface electrolocation techniques, such as surface mapping (3) and percutaneous electrode guidance (4,5), might help in overcoming difficult identification of anatomic landmarks, especially in infants and disabled children with limb and joint malformations.
Another distinctive feature of the pediatric period is the presence of congenital malformations, genetic disorders, and the consequences of fetal/neonatal asphyxia. These conditions impair normal development, and many deformities of bone and joint, as well as neurologic structures, worsen during childhood. These patients usually require repeated orthopedic surgeries; regional block procedures are particularly useful in these indications but anatomic deformities can make the techniques particularly difficult and might expose the patient to complications not usually seen in “normal children,” especially in regard to pressure point complications at unusual locations and postoperative monitoring of neurologic functions.
Differences in Growth of Spinal Cord and Spinal Canal
Before birth, during the embryonic period, the spinal cord entirely occupies the spinal canal. During fetal life, the growth of the vertebral column exceeds that of neural structures; thus, the spinal cord and roots are encased within the spinal canal, and the lower end of the spinal cord, as well as that of the dural sac, project at progressively higher levels, L4 and S3/S4, respectively, up to the ninth month of life. By the end of the second year, adult levels are reached, namely T12–L1 and S2, respectively. As a consequence, approaches to the epidural space above L4 should be avoided in small infants to prevent any potential damage to the underlying spinal cord. Also, it should be remembered that, in infancy, the dural sac ending is close to the sacral hiatus; cephalad introduction of the block needle during caudal anesthesia must but limited to 2 to 3 mm to avoid accidental dural puncture.
Delayed Myelinization of Nerve Fibers
Myelinization begins during the fetal period in cervical neuromeres and extends progressively both cephalad and caudad (6,7). This process is not fully completed until the end of the twelfth year of life. This lack of myelin in young patients makes the penetration of local anesthetic easier within the nerve fibers. Diluted solutions of local anesthetic can produce consistent
nerve blockade since the nerve fibers are thinner and shorter, which reduces the distance between successive nodes of Ranvier.
nerve blockade since the nerve fibers are thinner and shorter, which reduces the distance between successive nodes of Ranvier.
Incomplete Ossification of Skeleton
At birth, the skeleton is mostly cartilaginous. This has three practical implications: (a) cartilaginous structures are poorly seen on x-ray films, but ultrasonography can identify cartilage and differentiate it from bone, and visualize underlying neuraxial structures; (b) cartilage offers little resistance to sharp needles; and (c) undamaged ossification nuclei are necessary to allow normal bone growth and ossification. As a consequence, attempts to make boney contact should be avoided as often as possible in children, especially in infants, and use of sharp needles should be avoided; they may severely damage ossification nuclei and adjacent organs or vessels because they may not be halted by weak cartilaginous bone.
Development of the spine is not complete at the time of birth. It is gender-related, developing earlier in female than in male fetuses (8). The vertebral arches fuse posteriorly during the first year of life, and their junction with the vertebral body becomes ossified from the third to the sixth year (9,10). This posterior fusion remains incomplete at the level of S5 and also S4 most of the time, demarcating a V-shaped aperture, the sacral hiatus, which is covered by the sacrococcygeal membrane, a continuation of the ligamentum flavum, which is tightly adherent to different sacral ligaments. This strong membrane gives immediate access to the epidural space and can easily be penetrated by a block needle to provide caudal anesthesia.
Another peculiarity of the pediatric spine is that the sacrum is still made up of separate vertebrae, the fusion and ossification of which will not be achieved before adulthood (around the twenty-fifth year of life). Consequently, the epidural space can be approached at sacral levels in the same way as it can be at lumbar levels. On the other hand, since the sacrum is cartilaginous, it can be easily traversed by sharp needles, which can damage ossification nuclei (traumatic and infectious lesions) and pelvic/retroperitoneal organs. It should also be remembered that walking is necessary to the normal fusion of the sacral pieces. Patients confined to bed, especially those suffering from cerebral palsy, keep their intervertebral sacral discs unfused.
Delayed ossification of the iliac crest results in lowering of the crystal line (Tuffier line), which is used to locate the L4–L5 interspace to perform a lumbar epidural. In neonates and infants, this line passes one interspace lower; that is, at the level of the L5–S1 interspace (Fig. 27-1).
Flexures of the Spine
At birth, the spine has a single regular flexure. With the acquisition of head support, the cervical lordosis develops progressively by the sixth month, while the lumbar lordosis appears with the acquisition of stable sitting position between 9 and 12 months of life. This has implications for the performance of an epidural block depending on the spinal level. At birth, the orientation of the epidural needle is the same regardless of the intervertebral space. With the development of the spinal flexures, the orientation of the epidural needle must be adapted accordingly. At age 1, the spine has acquired its definitive shape, and the orientation of epidural needles should be the same as that suitable in adult patients at the same intervertebral space. However, these flexures can be easily emended by forced flexion almost throughout childhood due to the long-lasting persistence of spine flexibility, a major benefit of the pediatric period (in addition to the absence of osteophytes).
![]() Figure 27-1. Variation in size of the pelvic girdle, with subsequent changes in the relative position of the anterior superior iliac crests. |
Ability to walk is necessary to acquire normal flexures of the spine. Patients with cerebral palsy usually develop severe deformities of the spine, mainly scoliosis, which often necessitate surgical treatment and make epidural anesthesia particularly challenging, occasionally impossible.
Loose Attachment of Sheaths and Fluidity of Epidural Fat
An important feature of the pediatric period is the loose attachment of fascias and sheaths to nerve trunks and muscles. This allows extended spread of local anesthetic, resulting in high-quality nerve blockade whatever the technique used. This extended diffusion can occasionally result in unwanted or even undesirable distant nerve blocks. This also applies to the sheaths surrounding the spinal roots. The younger the patient, the greater the distal spread of the local anesthetic injected epidurally (caudal or lumbar epidural approach) along the spinal roots. Due to this extended spread, in addition to the incomplete myelination of nerve fibers, the quality of blockade is improved in relevant dermatomes and allows the use of lower concentration of local anesthetics, thus reducing the danger of systemic toxicity. However, leakage along spinal roots has also negative consequences: comparatively larger volumes of local anesthetics are necessary in infants to achieve the same cephalad level as in adults. This may be an incitation to administering potentially toxic amounts of local anesthetics or to attempting hazardous techniques such as distant insertion of epidural catheters (especially via the caudal route) to achieve satisfactory blockade.
Another factor affecting the spread of epidural solution is the consistency of the epidural fat. Up to 6 to 8 years of age, this fat is very fluid. After that age, it becomes more densely packed, with an increased proportion of elastic and collagenous fibers that can significantly impede the distribution of the local anesthetic and the progression of epidural catheters. Caudal anesthesia becomes less reliable after age 6, and the insertion of a distant epidural catheter becomes very hazardous and often impossible.
Not only is the longitudinal spread of local anesthetic increased in children, the diffusion process across nerve sheaths is increased too. In young patients, the structure of the endoneurium is loose, which makes it easily traversed in both directions (“fast in, fast out”). As the child grows, the
endoneurium becomes enriched with connective fibers, thus less permeable. The latency and also the duration of nerve blockade increase with age. The most significant characteristics of the pediatric patient that can influence the selection or performance of a regional block procedure are summarized in Table 27-1.
endoneurium becomes enriched with connective fibers, thus less permeable. The latency and also the duration of nerve blockade increase with age. The most significant characteristics of the pediatric patient that can influence the selection or performance of a regional block procedure are summarized in Table 27-1.
Differences in Pharmacology
Concomitantly with the growth process, the body surface area (BSA) increases throughout childhood; however, when related to patients’ weight, the BSA constantly decreases up to the end of adolescence. Drug prescriptions should ideally be related to the BSA, because the doses would be the same as (or in simple ratio with) adult doses, whatever the patient’s age (11). However, BSA is not as easy to obtain as body weight and, in practice, dose calculations are usually made according to weight, which requires constant adaptation of doses throughout childhood and could lead to dosage errors.
Local Anesthetics
Although the pharmacodynamics of local anesthetics is similar in children and adults, pharmacokinetic properties differ significantly, especially in neonates and infants (12).
Absorption from the Site of Injection
Nonionized local anesthetics cross almost freely the endothelium of capillaries surrounding the injection site. As the cardiac output and local blood flow of infants are two to three times greater than in adults, systemic absorption of local anesthetics is increased accordingly and vasoactive agents, such as epinephrine, are very effective in slowing down this systemic uptake, especially for short-acting agents.
Mucosal Topical Anesthesia
The absorption of local anesthetics from mucous membranes is increased in infants, and mucosal topical anesthesia has long been contraindicated. However, the technique can be used safely with certain precautions in young children, either by using specific transmucosal patches (13) or sprays of diluted solutions of local anesthetics, mainly lidocaine (14,15). However, it should be remembered that topical lidocaine exaggerates laryngomalacia, thus being a potential cause of increased morbidity in neonates and young infants (16).
Compartment Blocks
Injection of local anesthetics within closed fascial compartments allows easy blockade of nerves running through those compartments. A large compartment requires that a rather large dose be injected to produce satisfactory blockade, and it offers an extended surface of absorption, thus favoring early and high peak plasma concentrations. During intercostal nerve blocks with ropivacaine, the Tmax is reached 10 (venous blood) and 16 minutes (arterial blood) after injection, with Cmax values ranging from 0.3 (lowest values with 0.2% ropivacaine) to 5.6 μg/mL (highest values with 1% ropivacaine) (17).
Conduction Blocks (Including Epidural Anesthesia)
Amino amides are lipid-soluble and their absorption follows a biphasic curve. Lidocaine undergoes rapid absorption, and its blood concentration increases rapidly following repeat injections. Conversely, bupivacaine and ropivacaine are more efficiently retained at the injection site, and their systemic absorption is slower. In adults, this slow absorption process modifies the pharmacokinetic profile of long-acting agents after repeat injections or continuous infusion; the decrease in plasma concentration is slower than that expected from single-injection studies, and both the elimination half-life and distribution volume increase.
In children, the pharmacokinetic profiles of bupivacaine, levobupivacaine, and ropivacaine are consistently different. After a single injection in small infants, the Tmax of bupivacaine is around 30 minutes—the same as in adults—whereas that of levobupivacaine is approximately 50 minutes (18), and that of ropivacaine still longer: 115 minutes in infants and 62 minutes in children ages 3 to 5 years. Only children older than 5 years have Tmax values similar to those in adults (30 minutes) (19,20). Additionally, several studies also reported a concomitant increase of Cmax values of ropivacaine in infants and children up to 8 years of age (19), probably due to liver immaturity. This has clinical implications, as the Cmax of the most recent and supposedly safer local anesthetic could be reached after the infant had left the recovery room following short outpatient surgeries.
Plasma Protein Binding
Two main plasma proteins are involved in local anesthetic binding: human serum albumin (HSA) and α1-acid glycoprotein (AAG). Human serum albumin has a low affinity for local anesthetic, and many pharmacologic agents can compete at available binding sites. During the first months of life, plasma levels of HSA are low, especially in fasted infants; thus, the protection offered by HSA against systemic local anesthetic toxicity is low and decreases postoperatively (see also Chapter 3).
α1-Acid glycoprotein has an affinity for local anesthetics of five to 10,000 times greater than that of HSA, thus being very effective in preventing systemic toxicity, as only the nonbound free form is pharmacologically active. However, the plasma concentration of AAG is very low at birth (0.2–0.3 g/L) and does not reach adult levels (0.7–1.0 g/L) until the first year (21,22,23). Consequently, the free fraction of all local anesthetics is increased in infants. The maximum doses that can be safely administered must be significantly reduced in this age group, even though the plasma concentration of AAG increases postoperatively except in case of liver insufficiency (23).
Red Cell Storage
After their systemic absorption, local anesthetics also distribute in red blood cells, which retain 20% to 30% of the total dose, depending on the specific agent and the hematocrit. Usually, red cell storage does not affect significantly local anesthetic pharmacology except in the following conditions:
In neonates, due to high hematocrit values (which may exceed 70%) and enlargement of erythrocytes (physiologic macrocytosis), a significantly greater amount of local anesthetic can be entrapped in red blood (decreasing their Cmax after a single injection) and released secondarily (thus increasing the half-life at this age).
In infants, red cell storage is decreased due to physiologic anemia, thus increasing the dangers of systemic toxicity when the plasma protein binding sites are saturated (i.e., close to toxic blood concentrations) (24).
Distribution
Distribution of local anesthetics to organs and tissues depends on body fluid content and varies enormously with patient age (Table 27-2): This considerably affects the pharmacokinetics (Table 27-3). During the first 2 years of life, the peak plasma concentration and clearance of all amino amides is decreased after a single injection, while their elimination
half-life is consistently prolonged (25,26). During childhood, the clearance increases progressively and becomes higher than that measured in adults before returning to adult levels during adolescence.
half-life is consistently prolonged (25,26). During childhood, the clearance increases progressively and becomes higher than that measured in adults before returning to adult levels during adolescence.
Table 27-1 Characteristics that may affect selection and/or performance of regional block procedure in children | ||||||||||||||||||||||||||||||||||||||||||||||||||||||
---|---|---|---|---|---|---|---|---|---|---|---|---|---|---|---|---|---|---|---|---|---|---|---|---|---|---|---|---|---|---|---|---|---|---|---|---|---|---|---|---|---|---|---|---|---|---|---|---|---|---|---|---|---|---|
|
Table 27-2 Variation of body fluid distribution according to patient’s age | ||||||||||||||||||||||||
---|---|---|---|---|---|---|---|---|---|---|---|---|---|---|---|---|---|---|---|---|---|---|---|---|
|
If young patients (excluding the neonatal period) are comparatively less prone to develop systemic toxicity after a single local anesthetic injection, the same does not apply in cases of repeat injections or continuous infusion, because of progressive accumulation (26,27). During continuous infusion, the distribution volume and clearance of bupivacaine decrease consistently while the plasma concentration continuously increases, without reaching a plateau. In the same conditions, the distribution volume and clearance of ropivacaine tend to increase while plasma concentration of the unbound (toxic) form reaches a plateau 24 hours following the injection, with no further increase later (28). Little data are yet available on continuous infusion of levobupivacaine but, as for racemic bupivacaine, plasma concentrations do not reach a plateau and continue to increase with time even when a diluted solution (0.0625%) of levobupivacaine is infused (29).
Metabolism
Amino Esters
Amino amides
Amino amides mainly undergo hepatic microsomal metabolism by the P450 cytochrome enzyme superfamily, the activity of which is reduced during the first months of life (31,32,33). In adults, bupivacaine is mainly metabolized by the CYP3A4 enzyme (34), the activity of which is low during the first year; however, CYP3A7, a major enzyme in the fetus, is very active at birth and allows bupivacaine metabolism almost as efficiently as CYP3A4 in adults. The metabolism of both ropivacaine (35,36,37) and levobupivacaine (18) depends mainly on CYP1A2, which is not fully functional before the third year of life, and, to a minor extent, on CYP3A4. This enzyme immaturity is clinically relevant, but to a limited extent (low clearance, delayed Tmax and, for ropivacaine only, increased Cmax but within clinically acceptable levels); it does not preclude administering these local anesthetics in neonates and infants.
Table 27-3 Influence of age on pharmacokinetic parameters of amino amides | |||||||||||||||||||||||||||||||||||||||||||||||||||||||||||||||
---|---|---|---|---|---|---|---|---|---|---|---|---|---|---|---|---|---|---|---|---|---|---|---|---|---|---|---|---|---|---|---|---|---|---|---|---|---|---|---|---|---|---|---|---|---|---|---|---|---|---|---|---|---|---|---|---|---|---|---|---|---|---|---|
|
Clearance
Lidocaine
The hepatic extraction ratio of lidocaine varies from 0.65 to 0.75. The clearance is flow-limited and decreases when cardiac output decreases (12). Continuous infusion of lidocaine also results in considerable decrease in intrinsic clearance, which is further worsened by the fact that lidocaine metabolism is impaired by its own metabolites. Consequently, lidocaine should not be used for continuous infusion, especially in infants (see also Chapter 3).
Bupivacaine and Ropivacaine
The hepatic extraction ratio is low (0.35–0.45), and the clearance is rate-limited for both agents. Protein binding is the key factor influencing clearance. After a single injection, both local anesthetics have a low
clearance at birth, which progressively increases during the first years (38,39). During continuous infusion, the clearance of bupivacaine decreases by more than 40%, whereas that of ropivacaine remains unchanged; ropivacaine therefore represents the safest drug for continuous infusion, especially in infants (23).
clearance at birth, which progressively increases during the first years (38,39). During continuous infusion, the clearance of bupivacaine decreases by more than 40%, whereas that of ropivacaine remains unchanged; ropivacaine therefore represents the safest drug for continuous infusion, especially in infants (23).
Systemic Toxicity
Understanding systemic toxicity is not simple because only total plasma concentration of local anesthetics is easily measurable, whereas only the unbound form is pharmacologically active. Even the dosage of the unbound form in plasma is a poor reflection of the basically unknown concentration at the site of action (intraneuronal concentration of the ionized form, which is the form that blocks sodium channels).
Clinical signs of neurologic toxicity have been reported with plasma concentrations ranging from 7 to 10 μg/mL for lidocaine or mepivacaine, and from 1.5 to 2 μg/mL (intraoperatively) to 2 to 2.5 μg/mL (postoperatively) with bupivacaine. However, plasma concentrations of bupivacaine exceeding 4 μg/mL have been frequently reported without any evidence of clinical toxicity. From studies in adult volunteers (40,41,42), the following thresholds of toxicity of the unbound form of local anesthetics have been retained: 0.3 μg/mL for unbound bupivacaine; 0.6 μg/mL for unbound levobupivacaine or ropivacaine.
Because of low protein binding, infants are considered at greater risk of systemic toxicity. A noticeable difference with adults is that cardiac toxicity seems to occur concomitantly with central nervous toxicity, instead of being preceded by neurologic symptoms including convulsions (see Chapter 5).
Clinical Implications in Daily Practice
Short-acting agents (lidocaine and mepivacaine) should be restricted to single-injection techniques. For longer duration procedures, bupivacaine, ropivacaine, and levobupivacaine are suitable, either for single-injection blocks or for continuous infusion. In infants, use of ropivacaine or levobupivacaine for single-injection procedures does not represent a significant improvement over racemic bupivacaine in terms of safety; infants given these agents require a longer stay in the recovery room, as their plasma peak concentration is comparatively delayed. Conversely, for continuous infusion, ropivacaine represents a safer choice, as its clearance remains unchanged postoperatively, whereas that of either racemic bupivacaine or levobupivacaine decreases dramatically.
Table 27-4 Commonly used adjuvants and recommended doses | ||||||||||||||||||
---|---|---|---|---|---|---|---|---|---|---|---|---|---|---|---|---|---|---|
|
Opioids
The main pharmacokinetic difference between infants and older patients in regard to opioids (mainly morphine) pertains to the elimination half-life, which is consistently increased in infants. After lumbar epidural injection, peak plasma concentration of morphine is reached within 10 minutes. However, following therapeutic epidural or spinal doses, this plasma concentration is far too low to play a significant role in the development of analgesia, which depends only on the drug crossing the dura mater (43,44). The elimination half-life from cerebrospinal fluid (CSF) is similar to that of plasma and follows the same monoexponential elimination curve. As peak CSF concentration of morphine is very high shortly after an epidural injection, it takes 12 to 24 hours before the concentration falls below the minimal effective concentration (10 ng/mL). Therefore, adverse effects, including respiratory depression, can be observed more than 12 hours after an epidural injection, thus requiring close monitoring of vital parameters 24 hours after the last injection. Maximum recommended doses are 30 μg/kg for epidural morphine and 10 μg/kg for intrathecal morphine (Table 27-4). Patients given neuraxial morphine are not eligible for outpatient surgery (see also Chapter 40).
Short-acting lipid-soluble opioids such as fentanyl (1–2 μg/kg) and sufentanil (0.5 μg/kg) can be administered epidurally instead of morphine. They improve the quality of intraoperative analgesia, especially when coadministered with diluted local anesthetic solution. However, they do not prolong significantly the duration of postoperative pain relief unless a continuous infusion or repeat injections are made. Part, if not all, of their action is related to their plasma concentration, which can lead to early and acute respiratory depression (sudden apnea). This condition is very different from the progressive and delayed respiratory depression, preceded by generalized pruritus, sedation, and progressive bradypnea, which is observed following excessive doses of epidural morphine.
Another limitation must be considered before prescribing opiates in pediatrics. Experimental administration of a few doses—even a single dose—of opiates can induce tolerance in newly born animals (45,46,47); this tolerance similarly develops in human neonates and occurs more rapidly in preterm than in term neonates (48,49). Gender plays a consistent role, both in animals (50) and in human (51), with males developing a more intense tolerance than females. The underlying mechanisms of this early tolerance are essentially unknown but should encourage the practitioner to limit exposure of neonates to opiates as often as possible, using alternate medications (continuous local anesthetic infusion and/or other additives).
Other Additives
Epinephrine
Epinephrine is the most commonly used additive (5 mg/L or 1:200,000). Coadministered with local anesthetics (mainly short-acting agents such as lidocaine and mepivacaine), it decreases the plasma peak concentration (52,53) and increases local anesthetic duration of action, especially in children younger than 4 years old (54,55). Its vasoconstrictive properties have raised questions about possible spinal cord ischemia, but this proved to be unfounded (56). Nevertheless, many anesthesiologists prefer using a lower concentration of epinephrine in neonates and infants (2.5 mg/L or 1:400,000). At this concentration, the absorption rate of caudal bupivacaine is decreased by 25% (55), which consistently improves the safety of the technique. Another benefit of using local anesthetics with epinephrine is related to the hypersensitivity of children to IV epinephrine. In most infants and children, an accidental intravascular injection of a solution containing epinephrine would result in early (within 20 seconds) and readily evident ST segment elevation, T wave change (57), and hypertension (58) (see also Chapters 3, 4, 5).
Clonidine
Clonidine is another α2-adrenergic agonist (like epinephrine) that is extensively used neuraxially (59,60) and peripherally (61) (Table 27-4) at doses of 1 to 2 μg/kg. It increases (by approximately a factor of 2) the duration of blockade with no hemodynamic effects (in children) and decreases peak plasma concentration of the local anesthetic. Clonidine also produces slight sedation for 1 to 3 hours postoperatively, which is more beneficial than detrimental, as it does not preclude hospital discharge provided no more than 2 μg/kg has been administered. The addition of clonidine can often make unnecessary the placement of a catheter and a continuous infusion in many pediatric procedures, thus reducing the overall morbidity and costs of the technique. However, there are some respiratory concerns in neonates (62,63).
Ketamine
Ketamine, especially S-ketamine, has gained considerable interest as a local anesthetic adjuvant due to its blocking effects on N-methyl-D-aspartate (NMDA) receptors and its interaction with sodium channels in a local anesthetic–like fashion (including sharing a binding site with commonly used clinical local anesthetics) (64). Coadministered at a dose of 0.25 to 0.5 mg/kg, ketamine prolongs the duration of analgesia for many hours (65,66) with no significant, especially behavioral, adverse effects (see also Chapter 40).
Other Adjuvants
Many other additives have been occasionally (and questionably from an ethical point of view) administered along with local anesthetics (67). Some may be of interest (corticosteroids, buprenorphine, neostigmine, tramadol, midazolam, and biodegradable bupivacaine/polyester microspheres). However, many of them produce significant adverse effects. Furthermore, they have no approval for pediatric use.
Immaturity and Perception of Pain
Pain pathways and perception of pain are not mature at birth. They evolve throughout childhood. Furthermore, since the ability of children to communicate is limited both for anatomic (inability to speak, lack of vocabulary) and psychological/emotional reasons, assessment of pain is therefore difficult. The younger is the patient, the more difficult is the evaluation (see Chapters 5, 30, and 47).
Common Applications
Regional techniques are suitable for anesthetic, analgesic, and also a few nonanalgesic purposes.
Anesthetic Indications
Emergency Surgery and Patients at Risk of Severe Complications under General Anesthesia
After halothane had become available, regional anesthetic techniques fell almost completely into disuse. Some regional techniques survived only in a limited number of surgical indications in pediatrics including: (a) emergency procedures on the extremities and lower part of the abdomen and limbs in nonfasted children, and (b) elective surgery in children with severe medical conditions that could be dramatically aggravated either by the general anesthetic itself or the preparation of the patient, such as poor tolerance of fasting in certain metabolic diseases, facial deformities at risk of difficult intubation, malignant hyperthermia–susceptible patients, pulmonary cystic fibrosis, or severe asthma.
Many advances have occurred over the years, including the availability of new anesthetic agents, safer general anesthetic techniques, and new equipment for intra- and postoperative monitoring. Emergency surgery, per se, is no longer an indication for regional anesthesia, even though in many instances, when applicable, it could represent the first choice due to its lower morbidity rate.
Currently, regional blocks are particularly useful in trauma patients with extremity lesions, because they improve the comfort of the child, and allow pain-free clinical and radiologic examinations, wound dressings, even reduction of limb fractures without compromising the vital functions or affecting the evaluation of the status of the central nervous system. In a number of cases, nerve injury (such as median nerve rupture following a supracondylar fracture of the humerus) has resulted from the trauma itself. To avoid irrelevant claims, it is important to make a complete neurologic evaluation before any treatment is undertaken, preferably with the help of an external consultant, and to document the results in the medical files and to the parents. Then, after discussing with the parents and the child the different options for pain relief, a regional block with or without catheter placement will be mutually agreed to or denied.
Patients at risk of compartment syndrome are of special concern (see following discussion), but they should not be deprived of adequate pain relief, including by use of regional procedures (67). Some emergency conditions involving parietal disorders such as torsion of the testis, incarcerated hernia, or paraphimosis in nonfasted patients might benefit greatly from immediate surgery under a regional block procedure (e.g., caudal anesthesia, penile block)
without the hazards of either a crash induction or a delayed surgical repair under general anesthesia.
without the hazards of either a crash induction or a delayed surgical repair under general anesthesia.
Improvement in the management of diabetic patients and most metabolic disorders does not preclude the performance of a general anesthetic even though regional anesthetic techniques might represent a better choice in many instances. This is especially the case in patients presenting with the following disorders:
Nonstabilized acute or chronic respiratory insufficiency (status asthmaticus, cystic fibrosis)
Uncompensated metabolic or endocrine disorders
Neuromuscular diseases including myopathies, myotonic syndromes, myasthenia, some porphyrias, and some neurologic diseases with demyelinating disorders
Certain major skeletal deformities and polymalformative syndromes
Instability of the cervical spine is present in patients with Chiari syndrome, and frequent in Down syndrome or achondroplasia. Tracheal intubation in these patients is hazardous and might result in tetraplegia due to mobilization of the cervical spine. Many pediatric syndromes with facial deformities, microstomia, and palatal division are often a cause of difficult or impossible intubation even with the help of a fiberscope (and other devices); using a technique that avoids tracheal intubation is beneficial in these children.
Former preterm infants less than 60 weeks of post gestational age are prone to develop postoperative apnea. Inguinal hernia repair, a common procedure in these patients, is more safely achieved under regional anesthesia alone (spinal, caudal, or sacral/lumbar epidural anesthesia) than with any other technique of anesthesia, provided no sedative agent has been coadministered.
Anesthetic management is extremely difficult to provide to patients suffering from epidermolysis bullosa. Like severely burned patients, these infants are prone to suffer from intense and prolonged pain; regional block procedures may occasionally represent an effective and low-morbidity option, allowing surgery without tracheal intubation and providing long-lasting postoperative pain relief when a catheter is placed.
Elective Surgery
If based on medical indications alone, regional procedures would be extensively used for surgery on the extremities and lower part of the abdomen in children, as it is in adults. This is common practice in a few countries, especially in Northern Europe, where blocks are culturally well established and accepted both by adults and children. However, in the vast majority of countries, children usually fear needle sticks, and regional block procedures have to be performed in anesthetized children, a practice well-established and accepted in this age group (68,69).
Indications for Acute Pain Relief
Analgesia is currently the main indication for regional anesthesia in children, especially in elective surgery involving the extremities, abdomen and, to a lesser extent, the thorax, neck, and head. Intra- and postoperative pain is easily and efficiently prevented by single-injection or continuous techniques depending on the expected duration and intensity of postoperative pain (70,71,72). All pediatric patients can benefit from these techniques including neonates, preterm infants, and critically ill children (73,74,75). In some institutions, regional techniques are used in children undergoing cardiac surgery (76,77,78), but this application remains controversial. Outpatient surgery currently represents a major and cost-effective indication of regional blocks, mainly peripheral nerve blocks, with a very low morbidity provided that appropriate precautions are taken.
In recent years, catheter techniques allowing intermittent, on-demand, or continuous infusion of local anesthetics have become increasingly popular. Originally restricted to neuraxial techniques (28,79,80), catheter placement along a peripheral nerve path or a fascial plane is becoming routine practice in many institutions to provide long-lasting pain relief both in hospitalized and nonhospitalized patients (81,82,83). Regional blockade is commonly performed to provide analgesia for cancer pain and chronic pain relief. These techniques are detailed in Chapter 47 and are not considered in this chapter.
Nonanalgesic Indications
Occasionally, a regional block procedure may be performed in children for whom analgesia is not the first objective. Examples of these mainly nonanalgesic indications include vasodilation in patients presenting with ischemic disorders (84,85,86) and maintenance of a motor block throughout the recovery period in the patient with an unstable fracture, fragile nerve suture, or tendon repair.
Contraindications
Contraindications to regional block procedures are basically the same as in adult patients. Regardless of anesthetic technique, the patient’s full consent (and parental agreement for children) must be obtained preoperatively. Parent/child refusal should be considered a contraindication. Patients with severe psychoneurotic disorders should also be considered with the greatest attention, and most anesthesiologists would deny regional procedures in these patients.
Contraindications to Neuraxial Blocks
Absolute contraindications to neuraxial blockade include (87,88): infection at the puncture site, septicemia and meningitis; bleeding disorders, either constitutional or secondary to anticoagulant therapy; true allergy to local anesthetic, a very rare event (even with amino esters); uncorrected hypovolemia; and major vertebral anomalies (not spina bifida occulta—for many but not all authors, these include tethered cord syndromes and ventriculoperitoneal shunts).
Patients with mild seizure disorders should not be denied a regional anesthesia if they do not suffer any additional disorder prone to increase the morbidity of the procedure. Preexisting central nervous disorders and degenerative axonal diseases have long been considered as contraindications, at least relatively, even though few data support the hypothesis that a regional block worsens their course. A recent pediatric study involving 139 patients showed that preexisting neurologic disorders were not associated with bad neurologic outcome (the reverse was observed) after central block procedures (89).
Contraindications to Peripheral Nerve Blocks
Peripheral nerve blocks have few contraindications. Although infection close to the insertion site and allergy to local anesthetics are absolute contraindications, bleeding disorders may not contraindicate the performance of most peripheral blocks. Only procedures at risk of damaging large arteries (such as a supraclavicular block) or requiring deep needle insertion in closed muscular compartments (such as psoas compartment blocks or sciatic blocks at the thigh)
should be considered with caution.
should be considered with caution.
Patients at Risk of Compartment Syndrome
Compartment syndromes are (very) infrequent but can result in devastating complications. Certain trauma lesions and surgeries are more often concerned, such as displaced humeral supracondylar fractures or tibial shaft and forearm fractures treated by intramedullary nail fixation.
The extremities are divided into distinct muscular compartments by inextensible fascias, within which run nerves and vessels. The intracompartmental pressure must remain low to allow effective venous return. Any expanding lesion or disorder including muscle necrosis, edema, hematoma, injection of a large volume of fluid, or external compression by a cast (90) or a wound dressing inevitably increases the intracompartmental pressure, which may interrupt blood flow. Depending on the local lesions and state of consciousness, this interruption produces “tourniquet” pain, hypoesthesia, or a combination of both, typically summarized by the “5 P’s” eponym: pain, pallor, paresthesia, paralysis, and pulselessness. This severe condition must be recognized early, in order to perform extended fasciotomies before irreversible lesions have occurred.
Since pain is one of the cardinal symptoms, any technique aimed at attenuating pain, especially regional blockade, is often claimed to be contraindicated because of the possibility of hiding this presenting symptom and thus delaying the rescuing surgery. However, avoidance of analgesic strategies is not acceptable medically or ethically (91). Intense pain is often associated with bone fractures. Although fractures are very frequent in the pediatric population, compartment syndromes are very rare. Therefore, children should not be deliberately allowed to experience pain because of fear of compartment syndrome, which could be easily and precociously diagnosed using appropriate and easily available monitoring.
Adequate pain management, including continuous epidural analgesia (92), does not preclude early diagnosis but, on the contrary, favors it. In effect, the most sensitive clinical indicator of compartment syndrome is the increase in pain medication requirements, both in dosage and frequency, and this indicator precedes other clinical symptoms of compartment syndrome by an average of 7.3 hours (93). Therefore, adequate pain management, either by the IV route or by using regional procedures with low concentrations of local anesthetics (0.1%–0.15% bupivacaine, levobupivacaine, or ropivacaine) and avoiding motor blockade may considerably facilitate early diagnosis when significant changes happen: (a) because pain is no longer relieved and the child asks for additional analgesia; (b) less often, because the child becomes oversedated with the usual “normal” dose of parenteral narcotics; or (c) because the intensity and distribution of anesthesia is changing (progressive loss of any sensory perception and motor function).
The development of excruciating pain in a child deprived of analgesic medications should no longer be considered the “presenting” symptom of a compartment syndrome. Rather, patients at risk should not have a closed plaster cast and should be monitored by serial clinical evaluation of the distal perfusion and tissue oxygenation of the limb and by noninvasive monitoring of the intracompartmental pressure using, for example, near infrared spectroscopy (94), noninvasive “hardness” measuring devices (95), or ultrasound technique (96), even though these noninvasive techniques are not fully reliable. If the risk is considered high (e.g., displaced humeral fractures, intramedullary nail fixation of tibia or radius, obtunded patients), the intracompartmental pressure close to the fracture site should be invasively monitored because the technique is easy and inexpensive, requiring only a venous cannula, an IV line, and a pressure gauge (as for central venous pressure measurement) (93,97,98,99).
Selection of the technique, Equipment, and Anesthetic Solution
Selection of the Most Appropriate Block Procedure
Among the pediatric population, regional anesthesia is mostly used for analgesia, rather than anesthesia (as in adults). Therefore, it must offer a risk–benefit ratio superior to alternative analgesic approaches. In making a selection, the following points must be considered:
The distribution of anesthesia should cover not only the operative field but also the different areas involved in the surgery (e.g., sites of tourniquet placement, skin or bone graft taking).
The duration of sensory blockade should approximate that of postoperative pain.
The performance of the block should not aggravate the physical condition of the patient (e.g., risk of bacterial contamination, patient’s positioning prone to worsen trauma lesions).
The anesthetic and surgical techniques should be of the same magnitude (neuraxial blocks should be avoided for minor surgery).
The anesthesiologist must be familiar with the regional technique planned.
It is recommended that an alternative strategy be selected and explained to patients and family, in case of failure of the primary technique.
Techniques of Nerve Location
As most block procedures are performed on anesthetized children, a reliable technique of nerve localization independent of the patient’s cooperation is necessary (nerve stimulation, ultrasound guidance, or both); the overall morbidity of the two combined techniques, regional and general, should be lower than that of any other technique of anesthesia care, meaning usually that general anesthesia should be light (no muscle relaxants and no/minimal narcotics).
Basically, these techniques are the same as in adults; namely, loss-of-resistance technique for epidural anesthesia and compartment blocks, CSF aspiration for spinal anesthesia, and nerve stimulation for peripheral nerves and plexuses.
Ultrasound guidance is opening a new era, one that is particularly exciting in pediatric patients, especially in infants, whose nerves are very superficial and easily identified. However, the size of the probe can be difficult to handle and its application on the skin can change the anatomic landmarks and displace the block needle. The sterility of the procedure can occasionally be difficult to maintain throughout the procedure. Currently, block needles can only be reliably seen and their tip clearly identified when the major axis of the probe is in line with the shaft of the needle. If the probe is perpendicular to the shaft, only a cross-section of the needle can be seen (not always) and no information is obtained regarding the position of the needle tip. Two “tricks” can be helpful (personal data): (a) inject a small amount of 5% dextrose while the ultrasound machine is in Doppler mode—the stream produced at the tip of the needle will give a conical colored signal (dextrose will not compromise
further neurostimulation); (b) inject the local anesthetic after dispersing microbubbles in the solution.
further neurostimulation); (b) inject the local anesthetic after dispersing microbubbles in the solution.
Table 27-5 Pediatric doses and clinical characteristics of commonly used local anesthetics | |||||||||||||||||||||||||||||||||||||||||||||||||||||||||||||||||||||||||||||
---|---|---|---|---|---|---|---|---|---|---|---|---|---|---|---|---|---|---|---|---|---|---|---|---|---|---|---|---|---|---|---|---|---|---|---|---|---|---|---|---|---|---|---|---|---|---|---|---|---|---|---|---|---|---|---|---|---|---|---|---|---|---|---|---|---|---|---|---|---|---|---|---|---|---|---|---|---|
|
Selection of the Anesthetic Solution
Selection of the most appropriate local anesthetic depends on the surgical indication, the expected duration of intense postoperative pain, and the postoperative location of the patient. Commonly administered local anesthetics and their usual doses are displayed in Table 27-5. Short-acting local anesthetics (lidocaine and mepivacaine) are preferred for outpatient surgery. Longer-lasting agents do not preclude hospital discharge, provided motor functions have been restored at least in part and on the condition that the familial environment is competent (i.e., caring and able to make sound judgments). Levobupivacaine and ropivacaine are preferred for continuous infusion or repeat/on-demand injections after a catheter has been inserted along a peripheral nerve or neuraxially to provide long-lasting pain relief. Additives may consistently improve the quality and duration of blockade. Nonopioid additives (clonidine, ketamine) are particularly useful because they have few (and minor) adverse effects, thus not precluding early hospital discharge, and they prolong postoperative pain relief without requiring catheter placement.
For decades, long-lasting postoperative pain relief depended exclusively on continuous epidurals and patient hospitalization. Recently, the development of peripheral catheter techniques has simplified the management of postoperative pain, offering a better risk–benefit ratio than continuous neuraxial blockade, and even allowing hospital discharge and management at home in selected patients. For these techniques, continuous infusion (2–5 mL/h) or on-demand injections (2–5 mL) of low-concentration levobupivacaine or ropivacaine (0.1%–0.2%) represent the best and safest choice.
Equipment
The required equipment to perform regional block in children is basically the same as in adults (see Chapter 8), and this holds particularly true for peripheral nerve blockade (single-injection as well as catheter techniques).
For neuraxial blocks, some notable differences must be considered. For obvious reasons, shorter and thinner needles must be used in infants. For epidural anesthesia, the Tuohy bevel is virtually the only bevel used in children. The recommended medium for the loss-of-resistance technique has elicited many controversies (100). Basically, in children over 6 to 7 years of age, use of normal saline offers the same advantages as in adults. Conversely, in infants and, particularly in neonates, air is the most dependable technique of identification of the epidural space. For spinal blocks, the bevel/tip of the needle does not have the same importance as in adults; the incidence of postdural puncture headache remains (very) low and is not influenced by the design of the needle tip (101,102). Most critical is the distance from the tip of the needle to the distal orifice of the lumen; this distance must be as short as possible to avoid leakage outside the dura mater if the needle is not advanced enough past the dura mater, and to avoid potential damage to the cauda equina if the needle is introduced too deeply. Consequently, pencil-point needles do not improve the overall quality of the procedure and are even suspected of decreasing the success rate by favoring subdural spread of the local anesthetic. The most commonly used devices for regional block procedures in the pediatric patient are listed in Table 27-6.
Technique of Injection
The technique of injection is a critical factor of safety. Meticulous technique must be systematically followed:
Perform an aspiration test prior to any injection to verify the absence of blood reflux (or CSF reflux during an epidural).
Check cardiac monitoring during and after the injection of a test dose (0.1 mL/kg up to 3 mL) of a solution containing 0.5 to 1 μg/kg of epinephrine for 30 to 60 seconds; any elevation of ST segment or increase in T-wave amplitude (103,104,105,106,107), followed by an increase in blood pressure (108), but only inconstantly by tachycardia, suggests accidental IV injection and requires cessation of the procedure. When epinephrine is contraindicated, isoproterenol (0.05–0.1 μg/kg) should be used instead.
Inject the local anesthetic slowly (<10 mL/min) (109) to prevent high peak plasma concentration in case of accidental IV injection.
Stop any injection if unusual resistance is felt, to prevent potential intraneural injection.
Repeat aspiration tests during injection (every 5 mL) and prior to any catheter reinjection to help verify that the tip of the needle/catheter has not moved into a vessel (or through the dura mater).
Table 27-6 Recommended devices for most regional block procedures in pediatric patients | |||||||||||||||||||||||||||
---|---|---|---|---|---|---|---|---|---|---|---|---|---|---|---|---|---|---|---|---|---|---|---|---|---|---|---|
|
Patient Monitoring during the Block Procedure
Regional anesthesia is an anesthetic procedure. As such, it must be performed by anesthesiologists in an operating room environment with the usual monitoring recommended for any general anesthesia—monitoring of electrocardiogram (ECG), blood pressure, temperature, respiratory rate, oxygen saturation by pulse oximetry and, in patients (especially infants) under assisted ventilation, evaluation of tidal volume and end-tidal CO2. An IV line must be established prior to any injection of local anesthetic (110), and vitals parameters are to be reported on the anesthesia chart (see Chapter 8).
Assessment of the Block
The quality and distribution of blockade must be evaluated prior to surgery, whatever the patient’s age. This evaluation is not easy even in conscious children in the absence of a motor block. It requires that the anesthesiologist has gained the confidence of the child, prevents the child from seeing what he is doing (especially handling of needles), and makes comparative tests in nonblocked areas. Gentle skin pinching is the most dependable technique of sensory testing, especially in lightly anesthetized patients. Electrical stimulation using a nerve stimulator proved to be suitable in healthy volunteers (111,112) but little data are available in children.
Awake versus Asleep Block Placement
Regional blocks can be performed without sedation on cooperative and unpremedicated children (113). However, for elective surgery, children usually should be unconscious during the block procedure. If general anesthesia is not contraindicated for medical reasons, light general anesthesia can be safely used to perform a regional block and is widely accepted in pediatrics (69). In a large multicenter prospective study, only 23 rather minor adverse effects out of 24,000 regional procedures in children were noted, thus confirming the safety of combined regional and general anesthesia (68). A parallel study conducted during the same period in fully awake adult patients reported a similar rate of complications, the severity of which was, however, considerably greater, with several cases of permanent neurologic damage and deaths (114).
Performing a regional block in an anesthetized patient is usually considered unsafe in adults based on case reports (115,116,117), mainly related to thoracic epidural anesthesia (118). A study involving placement under general anesthesia of a lumbar epidural catheter in 4,298 consecutive adult patients did not report any severe complication (119), thus showing that, in adults too, the combined technique is an acceptable option. However, the real question does not pertain to the safety of the technique but to the benefits expected (relative to the risks) from such a combination (120).
In pediatrics, many reasons make the performance of regional blocks under light general anesthesia mandatory. Young children who have not acquired complete body image cannot grasp the concept of paresthesia. Additionally, whether peripheral nerve trunks and spinal roots have an intrinsic network of sensory innervation, the spinal cord, as well as the brain, does not have sensory nerve supply: Puncturing the spinal cord or the brain is asymptomatic (which allows stereotactic surgery in awake patients) whether or not the child is awake (this applies to adults patients too) (121); conversely, sticking a root or a nerve trunk elicits excruciating pain resulting in an immediate awakening reaction provided the child is lightly anesthetized with no muscle relaxant. Additionally, the background
of several decades of placement of epidural catheters and performance of regional blocks under general anesthesia meets the criteria of evidence-based medicine for safe practice.
of several decades of placement of epidural catheters and performance of regional blocks under general anesthesia meets the criteria of evidence-based medicine for safe practice.
A further point to be considered is the cooperation of the child, which is not easy to obtain; the occurrence of a panic attack at a critical step of a block procedure can be extremely detrimental. Therefore, all potentially dangerous procedures (interscalene block, thoracic epidural, paravertebral block, etc.) should not be attempted on unanesthetized patients, even if apparently cooperative, because at any time this cooperation may disappear and result in severe damage, as has even been reported in adults (122). In emergency conditions, when it is mandatory to provide pain relief without risking compromising the physical status (especially respiratory and cerebral) of the patient, a regional block offers the best risk–benefit ratio when applicable. In these circumstances, most children experiencing severe pain usually agree to the performance of the block technique while being fully conscious or only lightly sedated.
In conclusion, available data do not show an increase of morbidity when a regional block is performed on an anesthetized child. Conversely, the overall morbidity could be decreased by the combined technique for several reasons: prevention of the potential damages resulting from a loss of behavioral control during the block procedure; and avoidance of the hemodynamic and respiratory complications of deep general anesthesia, provided the associated general anesthetic is light (i.e., without muscle relaxants and with no more than 0.5 MAC of halogenated agents or equivalent IV agents).
The combined technique might also offer psychological benefits, even though difficult to establish, as many children fear needle puncture. In practice, the only pending question pertains to the criteria of “adulthood”: At what age a young patient is still managed as a child or as an adult.
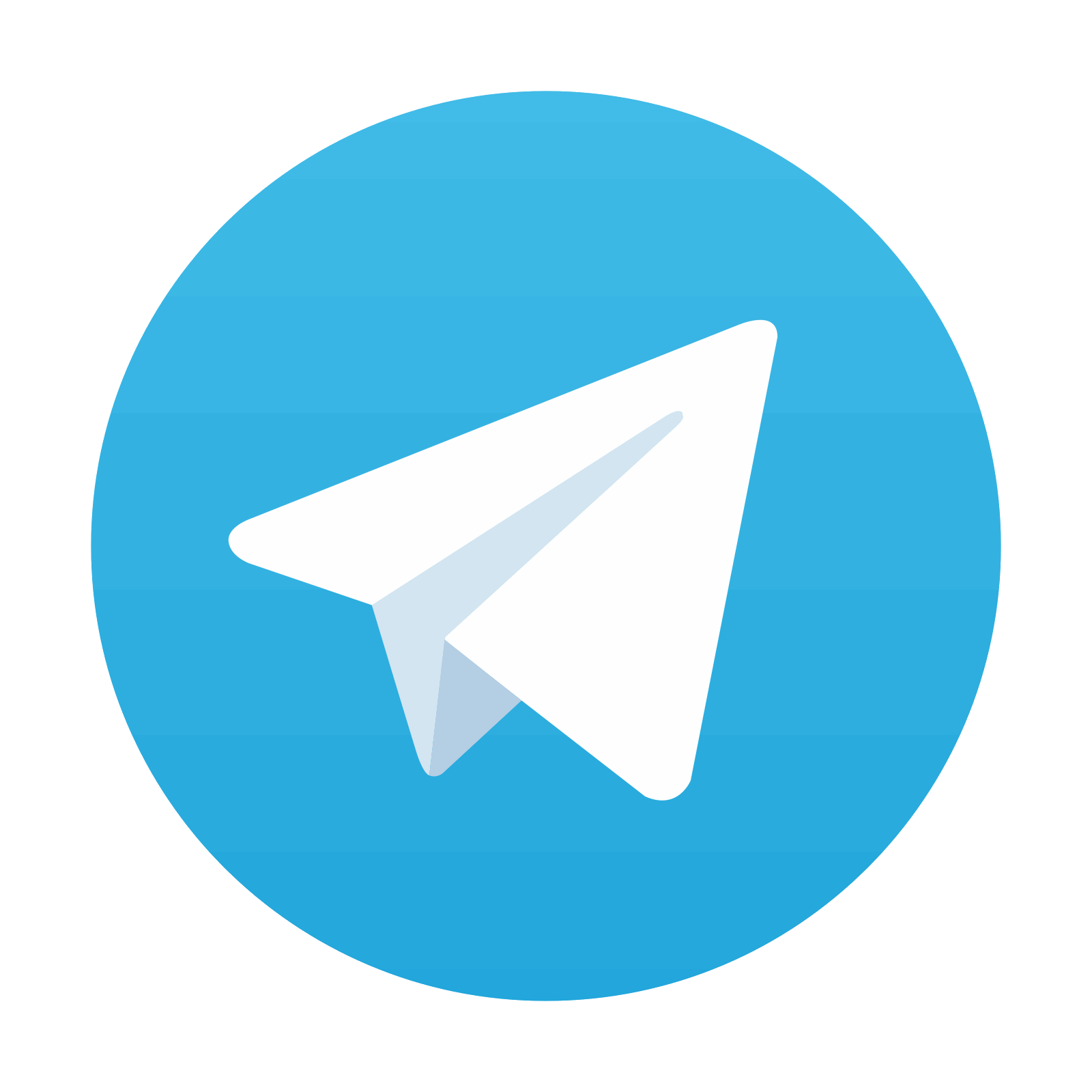
Stay updated, free articles. Join our Telegram channel

Full access? Get Clinical Tree
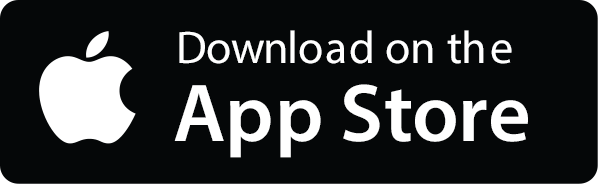
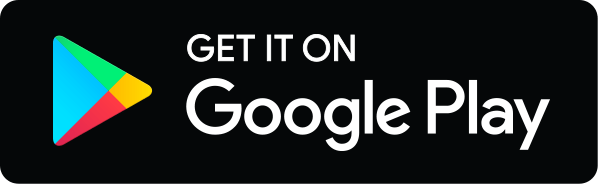