Neural Blockade for Obstetrics and Gynecologic Surgery
David O. Gorman
David J. Birnbach
Pain management in the parturient is a challenging clinical situation that requires knowledge of maternal and fetal physiology in addition to the technical skills required to achieve rapid, effective analgesia on demand. Because of the dynamic nature of parturition, the ability to rapidly achieve surgical anesthesia to facilitate cesarean delivery is also required. The capacity to work in a multidisciplinary environment is essential, as clinical situations in obstetrics can change instantly and frequently, requiring changes in the plan of anesthetic management.
Gynecologic surgery is ideally suited to the use of regional anesthesia alone or in combination with general anesthesia. For example, developments in the surgical and oncologic management of gynecologic cancer have resulted in an increased incidence of intra-abdominal lymph node sampling, which often requires higher levels of regional anesthesia and even modification of anesthetic technique.
Management of Pain in Labor
Labor pain is a subjective, multidimensional experience that is unique to each individual (1). The International Association for the Study of Pain (IASP) has defined pain as “an unpleasant sensory and emotional experience, associated with actual or potential tissue damage and described in terms of such damage” (2). Given the sensory and affective component involved in any painful process, pain assessment with appropriate institution of therapy is difficult to perform with precision in the parturient. Demographic, social, and psychological factors have all been shown to predict maximum pain during labor (3). Pain increases as labor advances and is greater when labor is induced or augmented (Fig. 24-1).
Labor pain is real, with subjective and objective manifestations. It has been described as severe, very severe, and intolerable in both parous (46%) and nulliparous women (61%). Use of the McGill Pain questionnaire in labor has shown comparisons between various painful conditions in multiparas and nulliparas (4,5) (Figs. 24-2 and 24-3). Although questionnaires such as the McGill Pain Questionnaire are valid and reliable, their interpretation can be limited by a patient’s previous experience of pain, and researchers continue to look for an accurate, reliable, and reproducible tool for pain measurement. The combination of muscle electromyography combined with visual analogue scales (VAS) has been used to assess the adequacy of pain management in labor (6). Attempts to find diagnostic markers in the measurement of pain, such as cerebrospinal levels of the proteinase inhibitor cystatin C, showed no difference between laboring and nonlaboring women (7). The development of functional magnetic resonance imaging (fMRI), positron emission tomography (PET), and neurophysiologic techniques have allowed researchers to examine cortical representation of painful stimuli and to establish the role of the brainstem, descending pathways, and multiple locations in pain processing (8,9,10). However, the use of these in the parturient has not been established.
Consequences of Labor Pain
Successful management of labor pain is essential to minimize physiologic perturbations that may have a potentially deleterious effect on the fetus. Pregnancy itself is associated with alterations in the maternal physiology due to anatomic and neurohumoral factors. Many of these physiologic changes can be exacerbated by the presence of untreated labor pain and, independently, can have an impact on the technique and effective management of anesthesia and analgesia in the parturient.
Respiratory System
Because of increased metabolism in pregnancy, maternal oxygen consumption can be increased by up to 60% (11) (Table 24-1). Respiratory changes in pregnancy mean that, despite the anatomic changes of increased diaphragmatic and reduced chest wall excursion, vital capacity, total lung capacity, and inspiratory reserve volume remain essentially unchanged. The reduced expiratory reserve volume (–25%) and functional residual capacity (FRC) (–20%) can result in airway closure in 50% of mothers at term (12,13). This situation can be further aggravated by concomitant obesity or in the assumption of a recumbent or lithotomy position. The supine FRC in the parturient has been shown to be only 70% of that in the upright position (14). Pain-associated hyperventilation can be associated with increases in minute ventilation of up to 300% in the second stage of labor (15). This can lead to hypocarbia and alkalosis with hypoventilation between painful contractions, resulting in maternal and fetal hypoxemia and altered maternal neurologic status (16). Studies have shown that administration of effective regional anesthesia is associated with reduced maternal hyperventilation and oxygen consumption (17,18).
Cardiovascular
Increases in stroke volume (25%) and heart rate (25%) can lead to a concomitant increase in cardiac output in early
pregnancy, resulting in increases of up to 35% to 40% of baseline values, and peaking in the early to mid third trimester (12,19,20) (Table 24-2). Uterine contractions can be associated with increases in both cardiac output (up to 25%) and blood pressure (5%–20%) (21). Effective labor analgesia is associated with attenuation of these related cardiovascular changes, with little detrimental effect on uteroplacental perfusion (22,23,24,25). Pregnancy imposes dynamic changes on circulation, with dramatic increases in cardiac output and decreases in systemic resistance. Because uteroplacental perfusion is very dependent on maternal blood pressure, profound hypotension is poorly tolerated and should be treated rapidly. Aortocaval compression is a common cause of hypotension and should be avoided in the term parturient, especially one who has received a neuraxial block. Although most pregnant women can tolerate the supine position without developing hypotension, some will develop supine hypotensive syndrome, which may be associated with profound hypotension, nausea, sweating, and loss of consciousness (Fig. 24-4). Left uterine displacement reduces aortocaval compression (Fig. 24-5).
pregnancy, resulting in increases of up to 35% to 40% of baseline values, and peaking in the early to mid third trimester (12,19,20) (Table 24-2). Uterine contractions can be associated with increases in both cardiac output (up to 25%) and blood pressure (5%–20%) (21). Effective labor analgesia is associated with attenuation of these related cardiovascular changes, with little detrimental effect on uteroplacental perfusion (22,23,24,25). Pregnancy imposes dynamic changes on circulation, with dramatic increases in cardiac output and decreases in systemic resistance. Because uteroplacental perfusion is very dependent on maternal blood pressure, profound hypotension is poorly tolerated and should be treated rapidly. Aortocaval compression is a common cause of hypotension and should be avoided in the term parturient, especially one who has received a neuraxial block. Although most pregnant women can tolerate the supine position without developing hypotension, some will develop supine hypotensive syndrome, which may be associated with profound hypotension, nausea, sweating, and loss of consciousness (Fig. 24-4). Left uterine displacement reduces aortocaval compression (Fig. 24-5).
![]() Figure 24-1. Likelihood of severe pain during labor. From Hardy JD, Javert CT. Studies on pain: Measurements of pain intensity in childbirth. J Clin Invest 1949;28:153–162, with permission. |
![]() Figure 24-2. Comparison of pain scores, using the McGill Pain Questionnaire, obtained from women during labor (5), from patients in the general hospital pain clinic (Melzack R. The short form McGill Pain Questionnaire. Pain. 1987;30:191) and an emergency department (Melzack et al. Acute pain in an emergency clinic: latency of onset and descriptor patterns. Pain. 1982;14:33). |
Table 24-1 Changes in Pulmonary Mechanics in Pregnancy | ||||||||||||||||||||||||||||||||||||||||||
---|---|---|---|---|---|---|---|---|---|---|---|---|---|---|---|---|---|---|---|---|---|---|---|---|---|---|---|---|---|---|---|---|---|---|---|---|---|---|---|---|---|---|
|
Table 24-2 Cardiovascular Changes in Pregnancy | ||||||||||||||||||||||||||||||||||||
---|---|---|---|---|---|---|---|---|---|---|---|---|---|---|---|---|---|---|---|---|---|---|---|---|---|---|---|---|---|---|---|---|---|---|---|---|
|
Neuroendocrine Effects
Interestingly, population-based studies suggest that marked gender variations occur in the reporting of pain. Some suggest that women report symptoms of pain more frequently than do men. In addition, both preclinical and clinical models of acute pain in humans suggest that females are more sensitive to certain noxious stimuli, and report a lower pain threshold than do men (26,27,28,29). (Although these data are of scientific interest, it would probably be inappropriate to mention these results in a busy labor and delivery ward.) Pregnancy itself is associated with an endogenous antinociception that is multifactorial in origin. Peripheral processes (ovarian sex steroids), visceral afferent activity, and spinal opioid antinociceptive pathways are all thought to play a role in pain modulation (30). Animal and human studies have demonstrated an increase in pain threshold in pregnancy, possibly due to increased secretion and reduced degradation of endorphins. This mechanism may be a developmentally induced endorphin-mediated response to counteract the pain of parturition, as pain has also been shown to be reduced by administration of opioid antagonists (31,32,33).
Endorphin levels in labor are related to the frequency and duration of uterine contractions and thus are considered to reflect the stress state of labor (34). The combination of pain, stress, and anxiety associated with parturition lead to an increase in maternal plasma catecholamines. The effect of increased plasma catecholamines on the maternal–placental unit has been demonstrated in animal studies, with reductions of up to 50% in uterine blood flow (35). Human studies have similarly shown increases in plasma catecholamines in pregnancy of between four and seven times that of nonpregnant controls following modest exercise (36). Plasma catecholamine levels are increased in labor by a factor of up to five times normal values, with a higher urinary level of catecholamine excretion. This leads to increases in maternal peripheral vascular resistance and blood pressure. Increased sympathetic activity with release of gastrin leads to gastric hyperacidity, which, combined with pain-related gastric stasis and reduced parasympathetic activity, increases the risk of aspiration should general anesthesia be required.
Pain in labor can have a marked physiologic effect on both mother and fetus. Increased epinephrine release from the adrenal medulla due to pain and anxiety leads to increased glucagon formation and increases in maternal free fatty acids and lactate levels. These hormonal responses to the increases in catecholamine levels, combined with maternal hyperventilation and compensatory metabolic changes during painful labor, lead to a state of maternal catabolism. The resulting stress on the maternal physiologic system leads to stimulation of the hypothalamus, with both adrenergic and pituitary responses. This leads to an increase in systemic cortisol, β-endorphins, and lipotropins and a corresponding increase in serum antidiuretic hormone (ADH) release. Increased sympathetic stimulation leads to increased gluconeogenesis
in the medulla and peripheral norepinephrine effects in the periphery.
in the medulla and peripheral norepinephrine effects in the periphery.
High maternal concentrations of catecholamines may have a deleterious effect on both mother and fetus in terms of uterine blood flow, and effective analgesia in labor is essential to reduce this effect (Figs. 24-6 and 24-7). Researchers have demonstrated a reduction of more than 50% in maternal plasma epinephrine levels associated with epidural and intrathecal analgesia (37,38,39). However, studies have shown that epidural analgesia in labor has not been associated with reductions in fetal and neonatal levels of catecholamines and β-endorphins, which are thought to play a central role in the mediation of several adaptive processes in the fetus after delivery, including surfactant synthesis, nonshivering thermogenesis, glucose homeostasis, and water metabolism (40).
Concomitant Illnesses
With improvements in reproductive medicine and increases in the average maternal age in pregnancy, the impact of increased sympathetic activity on cardiac output and maternal blood pressure may be associated with increased morbidity. This is illustrated by the increasing number of high-risk pregnancies in which maternal cardiac disease is a factor.
Psychological Effects of Pain in Labor
Perception of pain in labor can be modulated by many factors. The psychological effects of painful labor are not clearly understood. Anxiety and fear of pain may occur in the nulliparous patient, possibly in association with other ongoing psychological issues. Multiparous patients may also have a morbid fear of pain, especially if they have had a previously bad experience with medical care (41).
Negative outlook, low levels of education, and neuroendocrine factors all correlate with increased perception of pain. It is likely that these factors could impact on long-term psychological issues, including bonding, long-term emotional stress, and postpartum mood dysfunction (Fig. 24-8).
Regional Analgesia and Maternal–Fetal Circulation
The circulations of mother and fetus merge in the placenta and allow for the exchange of many physiologic substrates. In addition, the placenta serves a variety of functions, including the ongoing endocrine support of pregnancy. Fetal blood travels from the fetal heart to the placenta by way of two umbilical arteries and returns (nutrient-enriched and waste free) to the fetus by means of a single umbilical vein.
The placenta is composed of both maternal and fetal tissues that form a basal and chorionic plate. Essentially, the placenta is a semipermeable membrane that provides an interface for maternal and fetal circulation. The intervillous space separates the
plates and is subdivided by decidual tissue. Chorionic villi and spiral arteries project extensively into the intervillous space. Maternal blood flows into the intervillous space from the spiral artery and, at this site, maternofetal placental transfer occurs. It is estimated that up to 80% of the uterine blood flow passes through the intervillous space.
plates and is subdivided by decidual tissue. Chorionic villi and spiral arteries project extensively into the intervillous space. Maternal blood flows into the intervillous space from the spiral artery and, at this site, maternofetal placental transfer occurs. It is estimated that up to 80% of the uterine blood flow passes through the intervillous space.
Between 40% and 50% of the fetal cardiac output goes to the placenta, and a similar amount returns to the heart via the umbilical vein. Fetal blood enters the placenta via the two umbilical arteries, which arise from the internal iliac arteries. These arteries subdivide and eventually form umbilical capillaries that traverse the chorionic villi. The fetal blood flow is approximately 75 mL/kg/min. Although fetal and maternal blood pressures are uneven, placental transfer occurs rapidly for most drugs.
The umbilical–placental circulation is regulated by physiologic reflex changes and is also modified by neuroendocrine effects. Prostaglandins, endorphins, catecholamines, vasopressins, and other systemic factors modulate umbilical–placental perfusion.
Uterine Blood Flow
Uterine blood flow increases progressively throughout pregnancy and reaches a mean value of 500 to 700 mL/min at term. Uterine vessel blood flow is high, with a low vascular resistance (42). Pain may play a significant role, as uterine artery flow is dependent on maternal blood pressure and cardiac output. The uterine vessels are maximally dilated during pregnancy, thus no autoregulation is present. Therefore, any factor that interferes with blood flow through the uterus can potentially adversely affect fetal blood flow. Uterine blood flow is determined by the following relationship:

Factors that reduce uterine blood flow include maternal hypotension, hypovolemia, hemorrhage, aortocaval compression, and sympathectomy. Similarly, conditions that increase the frequency or duration of uterine contractions (uterine hypercontractility/tetany) and changes in hypertension-induced increases in uterine vascular tone may also adversely affect blood flow. Both general and regional anesthesia can have a marked influence on uterine blood flow, causing alterations in perfusion pressure and/or changes in uterine vascular resistance. Sympathetic blockade following neuraxial techniques, especially as practiced prior to 1990 using higher concentrations of local anesthetics, can produce maternal hypotension and thus reduce uterine blood flow. This can be marked in a fasting and potentially dehydrated parturient with ongoing insensible fluid losses. Fluid preloading prior to proceeding with regional anesthetic technique may reduce the impact of the local anesthetic–induced sympathectomy and resulting hypotension. This becomes more important as the concentration of local anesthetic increases and neuraxial analgesia progresses to neuraxial anesthesia. Appropriate fluid preloading, with maintenance of maternal cardiac output, has a beneficial effect on uteroplacental blood flow (43). Studies have demonstrated that maternal cardiac output correlates with the uterine artery pulsatility index and umbilical artery pH; therefore, these can be used as a surrogate index of fetal homeostasis (44,45). Volatile agents used in the maintenance of general anesthesia are potent myocardial depressants and can also lead to systemic vasodilatation. Aortocaval compression can further exacerbate this situation, and the parturient should, whenever possible, be positioned with left uterine displacement to optimize fetal oxygenation.
Hypotension as a result of regional anesthesia for labor and cesarean delivery can have a potentially deleterious affect on the fetus. Intravenous (IV) fluid therapy and avoidance of aortocaval compression are essential, but often must be combined with pharmacologic management. Ephedrine is extensively used in obstetric anesthesia for treatment of the effects of sympathectomy, as it has a low incidence of uteroplacental vasoconstriction. However, ephedrine can produce tachycardia and has been shown to produce depression of fetal pH and base excess (46). Ephedrine has mixed α- and β-adrenergic effects that may lead to an increase in blood pressure secondary to increased cardiac output and increased peripheral vascular resistance. Studies in pregnant ewes demonstrated that ephedrine was superior to metaraminol and methoxamine in maintaining uteroplacental blood flow (47).
Phenylephrine is a potent vasoconstrictor that is easy to titrate and has minimal associated fetal acidosis (Fig. 24-9). However, phenylephrine may decrease maternal heart rate and cardiac output and, although safe in the healthy parturient, few data are available on its use in high-risk cases. Vasopressors such as phenylephrine have direct α-adrenergic receptor activity and have been shown in animal studies to increase intrinsic vascular resistance and thus reduce uterine blood flow (48). There has been renewed interest and popularity in the use of phenylephrine as a vasopressor in routine clinical practice; studies have demonstrated that it is as efficacious as ephedrine in maintaining maternal blood pressure and umbilical artery pH values (49,50,51). Although a recent study has suggested that combination therapy with ephedrine and phenylephrine may be the optimal technique for maintaining normotension, ephedrine is still considered the vasopressor of choice by many anesthesiologists (52,53).
Relative to the high-risk parturient, animal studies of compromised maternal–fetal physiology with maternal hypoxemia and hypotension found that phenylephrine administration was associated with impaired uterine and placental hemodynamics and increased fetal lactate concentrations, when compared to ephedrine (54). Despite the more favorable effects on uterine and placental circulations of ephedrine over phenylephrine, no significant differences in fetal acid–base status or lactate concentrations were observed in other similar investigations (55). At Jackson Memorial Hospital, we have changed our practice so that mild hypotension is still treated with 5 mg of IV ephedrine. If this dose does not correct the hypotension, combinations of ephedrine and phenylephrine are subsequently administered.
Furthermore, in studies to evaluate the impact of both vasopressors in the maintenance of maternal–fetal parameters during ritodrine tocolysis and epidural-induced hypotension, both agents were shown to provide similar restoration of maternal mean arterial pressure. However, ephedrine was shown to be superior to phenylephrine in restoring uterine blood flow and fetal oxygenation (56). Comparison of both agents in the treatment of hypotension following anesthesia for cesarean delivery suggested that, unlike ephedrine, phenylephrine had a greater impact on uterine and placental flow indices as measured using Doppler velocimetry. These findings suggest that, although both agents have been well described in the treatment of maternal hypotension, caution should be exercised with phenylephrine in the presence of a potentially compromised fetus (57).
Regional Anesthesia and the Placenta
The potential transfer of anesthetic agents across the placenta is a concern in the management of pain in the parturient. Drugs cross the placenta by three main processes: simple diffusion, active transport, or pinocytosis. The extent of drug transfer is dependent on numerous factors including lipid solubility, molecular weight, protein binding, concentration gradient, and maternal and fetal pH. The Fick principle governs the rate of transfer of a drug across a membrane:

where Q/t is the rate of diffusion, K is the diffusion coefficient, A is the surface area of membrane available for exchange, Cm-Cf is the concentration gradient between the maternal and fetal circulations, and D is the thickness of the membrane.
The potency and duration of action of local anesthetic agents is determined by their lipid solubility. This leads to the binding of a drug close to its target of action and also to reduced metabolism by liver enzymes and plasma esterases. Local anesthetic agents are weak bases and are poorly water soluble. Structurally, most local anesthetic agents are composed of a benzene ring (lipid-soluble/hydrophobic) and an amine group (water-soluble/hydrophilic), which is ionizable. These components are linked by a chemical chain, the structure of which is either ester (–CO) or amide (–HNC). The uncharged hydrophobic fraction of drug crosses the lipid membrane and initiates blockade of the hydrophobic sodium (Na+) channel. The extent of hydrophobicity of a drug may also increase its risk of toxicity. Placental transfer is thus more active for lipid-soluble anesthetic agents.
Local anesthetics agents bind systemically to tissue and plasma proteins (albumin and α1-acid glycoproteins [AAGs]). The protein-bound fraction is pharmacologically inactive, thus increased protein binding leads to reduced transfer of local anesthetic agent across the placenta. High-molecular-weight molecules are less likely to cross the placenta, whereas molecules with weights under 500 daltons will cross easily. Most drugs administered to the parturient in labor have low molecular weight and therefore transfer easily to the fetus.
Highly ionized substances with low lipid solubility (such as non-depolarizing muscle relaxants) have very limited transfer. In fact, non-depolarizing muscle relaxants have been directly administered to the fetus during fetal surgery, with no impact on the mother. Fetal pH and serum protein binding directly affect drug disposition in the fetal circulation (58).
The degree of ionization greatly influences drug transfer because only nonionized portions of the drug can cross the placenta. The degree of ionization of a drug is determined by the Henderson-Hasselbalch equation:

where pKa is the negative logarithm of the acid dissociation constant. The pKa of a drug is the pH at which it is 50% ionized and 50% nonionized.
The pKa of a local anesthetic agent determines the ratio of ionized to uncharged (base) form of the drug. The pKa for local anesthetic agents ranges from 7.6 to 9.2. The pKa generally correlates with the speed of onset of most local anesthetics agents. The closer the pKa is to physiologic pH, the faster its onset of action. Bupivacaine has a pKa of 8.1 and is only 15% nonionized at physiologic pH. Lidocaine has a more rapid onset, with a pKa of 7.7, and with 25% of drug nonionized at physiologic pH. However, 2-chloroprocaine is an exception, exhibiting a rapid onset of action, possibly due to increased tissue penetrability, despite having a pKa of 9.0. Similarly, agents with a pKa closer to physiologic pH have a higher degree of placental transfer. The umbilical vein-to-maternal artery (UV:MA) ratio for mepivacaine (pKa 7.6) is 0.8, compared to 0.3 for bupivacaine (pKa 8.1) (see also Chapters 3 and 4).
Pain thresholds may be increased in pregnancy, with a possible corresponding increased sensitivity to local anesthetic agents (32,59). Therefore, changes in maternal and fetal acid–base status, combined with altered protein binding, can have a major impact on the management and technique of regional anesthesia. Fetal acidosis leads to increased ionization of local anesthetic agents that have crossed the placenta into the fetal
circulation. These ionized agents are unable to transfer back (ion trapping) across the placenta into the maternal circulation. Fetal acidosis and systemic insult can lead to increased perfusion of the heart and brain, thus increasing the delivery of drug to these important organs. This can lead to further accumulation of drugs in an already compromised fetus (60). Although this is a major theoretical concern, the clinical significance of this phenomenon is unclear (see Chapter 3).
circulation. These ionized agents are unable to transfer back (ion trapping) across the placenta into the maternal circulation. Fetal acidosis and systemic insult can lead to increased perfusion of the heart and brain, thus increasing the delivery of drug to these important organs. This can lead to further accumulation of drugs in an already compromised fetus (60). Although this is a major theoretical concern, the clinical significance of this phenomenon is unclear (see Chapter 3).
The fetal circulation can have a major impact on drug distribution within the fetus. As previously described, drugs enter the fetal circulation via the umbilical vein following their passage across the placenta. The liver is perfused by umbilical venous blood, and significant hepatic uptake can occur, which may have a protective effect on the fetus (61). Approximately 40% of the umbilical venous blood bypasses the liver via the ductus venosus (62). Further dilution of umbilical venous blood across the foramen ovale and ductus arteriosus can also occur.
Parturition and Stages of Labor
The delivery of the fetus (parturition) is achieved by the intermittent involuntary contraction of smooth muscle, facilitated by maternal bearing down. When stretched, the uterus has the ability to expel any foreign body within its cavity. The precise mechanism of maintenance of pregnancy and onset of labor is unknown, although numerous hypotheses have been suggested. Declines in serum progesterone, increasing levels of estrogen, increased prostaglandin production, increases in oxytocin receptors, structural changes in the myometrium, and many fetal factors are all thought to play a role in the onset of labor (63).
Labor is divided into three stages, each stage of which has a particular type and nature of pain. The first stage begins with maternal awareness of regular and painful uterine contractions and ends with complete dilatation of the cervix. This discomfort usually reflects a minimal intrauterine pressure of approximately 25 mm Hg and reflects stretching and distension during contraction (64).
During the first stage of labor, the pain experienced is mostly visceral in nature, arising from afferents in the uterus and its adnexa during contractions. The painful afferents pass through the superior hypogastric plexus and lumbar sympathetic chain and enter the spinal cord through the posterior segments of T10–T12 (Fig. 24-10). The intensity of pain is related to the contraction strength and subsequent pressure generated against the cervix and perineum (65). Chemical metabolites resulting from neurohumoral pathways or contraction-induced ischemia may also lead to local stimulation of painful chemoreceptors by several chemical mediators including prostaglandins, serotonin, substance P, and lactic acid (64,66).
The painful afferents from the lower uterine segment and the endocervix have their cell bodies located in the thoracolumbar dorsal root ganglia. However, the upper vagina and vaginal cervix have cell bodies in the sacral dorsal root ganglia that are mostly comprised of C-fibers. These enter the spinal cord through the dorsal
root ganglion and develop a loose network of synapses in the ventral and dorsal horn (superficial and deep). Because of the significant convergence of the visceral pain pathways, the pain experienced in this stage of labor is often poorly localized and can be referred to the rectum, lower back, and along the abdominal wall (67). As the fetal head descends along the birth canal and into the pelvis, pressure on the pelvic viscera and stimulation of the lumbosacral plexus can lead to perception of pain from L1–S1. This has implications in the provision of neuraxial analgesia, because to achieve effective pain management during this stage, the lumbar and upper sacral nerve roots require effective blockade. Neurons at the level of the dorsal horn transmit afferent information to the contralateral spinothalamic tract and other ascending pathways to the higher centers of the brain responsible for the localization and affective component of pain (see Fig. 31-19).
root ganglion and develop a loose network of synapses in the ventral and dorsal horn (superficial and deep). Because of the significant convergence of the visceral pain pathways, the pain experienced in this stage of labor is often poorly localized and can be referred to the rectum, lower back, and along the abdominal wall (67). As the fetal head descends along the birth canal and into the pelvis, pressure on the pelvic viscera and stimulation of the lumbosacral plexus can lead to perception of pain from L1–S1. This has implications in the provision of neuraxial analgesia, because to achieve effective pain management during this stage, the lumbar and upper sacral nerve roots require effective blockade. Neurons at the level of the dorsal horn transmit afferent information to the contralateral spinothalamic tract and other ascending pathways to the higher centers of the brain responsible for the localization and affective component of pain (see Fig. 31-19).
The second stage of labor begins with full dilatation of the cervix and ends with the delivery of the fetus. Ongoing pain stimuli from contractions of the uterine body continue, in addition to pain from distension of the lower uterine segment. The contribution of pain from cervical dilatation slowly diminishes. However, the presenting fetus, as it presses on pelvic structures, leads to stimulation of superficial somatic structures and their afferents via the pudendal nerve (S2–S4); pain arises from tearing of ligaments, and pressure on fascia, muscles, bladder, urethra, and rectum. The pudendal nerve also supplies the motor fibers to the skeletal muscles of the pelvic floor and perineum. The anterior perineum also receives fibers from the genital branch of the genitofemoral nerve (L1–L2) and the ilioinguinal nerve (L1). The lateral aspect of the perineum is also supplied by the posterior femoral cutaneous nerve (S1–S3) (68,69,70) (Fig. 24-11). Thus, pain in the second stage is often sharply localized. This somatic pain is transmitted via C- and Aδ-fibers that enter the spinal cord through the dorsal roots and terminate in synapses in the ipsilateral superficial laminae of the dorsal horn. Provision of appropriate analgesia during the second stage of labor requires analgesia from a level of T10 extending caudally to include the somatic nerves of the perineum (S2–S4). This may not always be blocked by a continuous epidural using low-dose anesthetic, and increased dosing (top-offs) may be necessary at this stage.
![]() Figure 24-10. Peripheral pain pathways during labor (see also Fig. 31-23). |
The third stage of labor commences with the completed delivery of the fetus and ends with the completed delivery of the placenta and attached membranes. The analgesia requirements for the third stage of labor are usually less than that of the first and second stages. However, if there is delay in the delivery of the placenta or if manual removal by the obstetrician is required, increased doses of analgesia or anesthesia of the perineum and lower uterus are usually required.
Management of Pain in Labor
Comprehensive patient education programs regarding labor pain and its management options form a key part of antenatal care. Pain management in labor ideally should be multidisciplinary in nature. Appropriate psychological preparation and support is essential whenever possible. This can be accomplished by high levels of antenatal care and patient education prior to onset of labor, thus enabling the patient to make decisions in advance in relation to her pain management and also increasing the likelihood that, despite the pains of labor, the parturient has made an informed decision and gives vital consent.
The use of psychological techniques including positive outlook, relaxation therapies, and diversionary techniques may be considered as adjuncts to neuraxial pain management (71). The role of the patient’s partner in this situation cannot be underestimated; relatives and caregivers who provide an appropriately relaxing and supportive environment are clearly beneficial. Use of hypnosis in labor has been limited by refractory patients, whereas the role of nonconventional therapies including aromatherapy and reflexology is unclear. Transcutaneous electrical nerve stimulation (TENS) can be useful in early labor when pain scores are low, but its role in established painful labor has not been supported (72).
Intradermal injections of water have been described in the management of lower back pain in labor. These injections are thought to produce analgesia by counterirritation. Relief of labor back pain has been described for up to 90 minutes following injection (73).
Systemic Analgesia
Systemic analgesia is used when regional techniques are either not available or contraindicated. This may include situations in which no anesthesiologist is available, or in the high-risk parturient in whom neuraxial techniques are contraindicated, such as in a coagulopathic patient. The administration of intramuscular opioids has been a well-established technique that has been widely used historically, despite suggestions that labor pain is insensitive to systemically administered opioids and that the IV route is preferable to intramuscular
injection (74). The associated maternal and fetal neurologic depressant effects, combined with risks of accumulation of excitatory metabolites, have limited the use of parenteral opioids. Lack of established efficacy, combined with nausea, vomiting, and sedation all preclude widespread use and have made this option unpopular with parturients (75). Opioid administration in labor has been shown to produce relief of moderate pain in 70% to 80% of patients, but only in 35% to 60% of those experiencing severe pain (64,65). Of note, the majority of women in labor experience severe pain, as illustrated by the classic studies performed more than 50 years ago (76,77,78,79,80,81,82).
injection (74). The associated maternal and fetal neurologic depressant effects, combined with risks of accumulation of excitatory metabolites, have limited the use of parenteral opioids. Lack of established efficacy, combined with nausea, vomiting, and sedation all preclude widespread use and have made this option unpopular with parturients (75). Opioid administration in labor has been shown to produce relief of moderate pain in 70% to 80% of patients, but only in 35% to 60% of those experiencing severe pain (64,65). Of note, the majority of women in labor experience severe pain, as illustrated by the classic studies performed more than 50 years ago (76,77,78,79,80,81,82).
Insufficient evidence is available to evaluate the comparative efficacy and index of safety among opioids used in labor analgesia. Intramuscular meperidine (Demerol) has been widely used for labor analgesia and is associated with modest analgesia (76), but the potential for maternal, fetal, and neonatal side effects exist. Accumulation of the excitatory metabolite normeperidine has not been widely described in labor. In terms of pain relief, interval to delivery, and instrument/operative delivery, no difference was noted between meperidine and tramadol. However, meperidine was associated with a higher incidence of nausea, vomiting, and drowsiness (77,78).
Use of fentanyl has been considered because of its potency (100 times that of morphine) and rapid onset. Intravenous doses of 50 μg of fentanyl have been reported to be of value in the first stage of labor, although its short duration of action compared to morphine requires frequent administration or use of continuous infusions. In addition, because of its lipid solubility, fentanyl has been shown to cross the placenta easily, and fetal levels of drug can rise rapidly (79). Use of patient-controlled IV remifentanil (0.27–0.93 μg/kg) has been shown to be superior to IV meperidine in terms of pain relief, with a lower incidence of fetal heart rate abnormalities; research is ongoing to find optimal dosing regimens (82). Finally, the potential benefit of combining μ- and κ-receptor agonists has not been borne out by clinical studies (81).
Neural Blockade in Obstetric Anesthesia and Analgesia
Regional blockade provides the least depressant and most effective form of analgesia and anesthesia in obstetrics. Peripheral techniques are commonly used by the surgeon in situations in which regional techniques have not been utilized or to supplement blockade in the presence of sparing of the sacral dermatomes due to an ineffective block.
Infiltration of local anesthetic along the perineum is performed in a fan-shaped pattern along the ischiorectal fossa prior to episiotomy or for suture of same post delivery. Lidocaine 0.5% to 1.0% with epinephrine (1:200,000) can be used, up to a total dose of 7 mg/kg. Local anesthetic infiltration has been described for emergency use for cesarean delivery, although central neuraxial blockade has largely replaced this practice. The technique may still have a place in the situation of profound fetal bradycardia with no anesthesia provider present (83).
Paracervical and Pudendal Blocks
The paracervical block is an alternate technique for the parturient who does not want or cannot receive central neuraxial block for the first stage of labor. It is a relatively simple procedure to perform and provides relief of pain in the first stage of labor without adversely affecting the progress of labor. Local anesthetic is injected submucosally into the fornix of the vagina lateral to the cervix. Blockade of neural transmission through the paracervical ganglion is achieved, which lies lateral and posterior to the junction of the cervix and uterus. Paracervical blockade does not affect somatic sensory fibers from the perineum and thus offers no pain relief for the second stage of labor. Currently, this technique is predominantly performed by obstetricians for nonobstetric surgery, such as dilatation and curettage. Its use in obstetric practice has profoundly decreased over the past two decades as neuraxial blockade has become safer, more effective, and increasingly more available. Significant adverse reactions to paracervical blockade have been reported including profound fetal bradycardia, systemic local anesthetic toxicity, postpartum neuropathy, and infection (84). It has been suggested that the etiology of fetal bradycardia is related to reductions in uterine blood flow and elevated local anesthetic levels in fetal blood (85,86) (Fig. 24-12).
The pudendal nerves originate from the lower sacral nerve roots (S2–S4). These nerves transmit sensory innervation for the lower vagina, vulva, and perineum, and provide motor innervation to the perineal muscles. Injection of local anesthetic behind the bilateral sacrospinous ligament transvaginally provides excellent anesthesia of these nerves (87). Pudendal nerve block provides satisfactory analgesia for spontaneous and instrumental vaginal delivery at the level of the vaginal outlet. However, pudendal nerve blocks do not provide sufficient analgesia for mid-forceps delivery, repair of vaginal lacerations, or exploration of the uterine cavity (88). Although complications are rare, failure of block, systemic local anesthetic toxicity, infections, and hematoma formation have been described. The proximity of needle placement to the fetal head may also be an issue of great concern (89) (Fig. 24-13).
Lumbar Sympathetic and Thoracolumbar Paravertebral Blocks
Anatomy and Innervation
The autonomic nervous system is divided into the sympathetic and parasympathetic nervous systems. Preganglionic nerves of the sympathetic nervous system arise from the thoracic and lumbar segments of the spinal cord; in the parasympathetic
nervous system, they arise from the brainstem and sacral spinal cord. Most visceral structures (except blood vessels and sweat glands) receive both sympathetic and parasympathetic innervation. The sympathetic chain lies along the anterolateral surface of the lumbar vertebral bodies. The psoas muscle and fascia separate the lumbar sympathetic chain from the somatic nerves. Anatomic studies have shown marked variability in the distribution of the ganglia, but suggest optimum blockade of the ganglia will result from sympathetic blocks at the level of the L3 vertebral body (90). However, caution should be exercised at the level of the middle one-third, where the segmental vessels pass under the dense fascia toward the epidural space, thus creating a potential passageway to the epidural space (90,91).
nervous system, they arise from the brainstem and sacral spinal cord. Most visceral structures (except blood vessels and sweat glands) receive both sympathetic and parasympathetic innervation. The sympathetic chain lies along the anterolateral surface of the lumbar vertebral bodies. The psoas muscle and fascia separate the lumbar sympathetic chain from the somatic nerves. Anatomic studies have shown marked variability in the distribution of the ganglia, but suggest optimum blockade of the ganglia will result from sympathetic blocks at the level of the L3 vertebral body (90). However, caution should be exercised at the level of the middle one-third, where the segmental vessels pass under the dense fascia toward the epidural space, thus creating a potential passageway to the epidural space (90,91).
White rami communicantes are aggregates of preganglionic fibers that leave the spinal cord from the intermediolateral cell column and lateral horn (T1–L2). They leave the spinal canal with the corresponding anterior spinal nerves and join the sympathetic chain. They are white in color, as they are only slightly myelinated. The postganglionic fibers (gray rami communicantes) leave the sympathetic chain in association with the spinal nerves. They are unmyelinated and appear gray in color. The preganglionic fibers (white rami communicantes) may synapse in the sympathetic chain and then travel with the spinal nerve. Some may ascend or descend to another level of the spinal cord and synapse at this level before traveling with the spinal nerve. Some white rami communicantes transverse the sympathetic chain without synapsing. These preganglionic sympathetic fibers form the greater, lesser, and least splanchnic nerves (T6–T12), which synapse in the celiac and superior mesenteric ganglia within the abdomen. The postganglionic sympathetic fibers from these ganglia are distributed to the abdominal organs. The sympathetic nerves are usually accompanied by afferent fibers conducting sensory information from the appropriate viscera (see Figs. 39-1, 39-2, 39-5).
Lumbar Sympathetic Blockade
Early studies have suggested that the lower uterine and cervical afferent sensory fibers join the sympathetic chain at L2–L3. There were some reports of effective blockade of afferent sensory transmission from these structures to the spinal cord using lumbar sympathetic blocks (92,93). Lumbar sympathetic blocks provided effective analgesia of long duration (94). In addition, lumbar sympathetic blocks in nulliparous parturients with induced labor were associated with effective analgesia and rapid cervical dilatation during the first 2 hours of analgesia, and were also associated with a shorter duration of the second stage when compared to epidural analgesia (95). These findings, however, have not widely convinced clinicians to embrace this technique, which remains unpopular.
Blockade of the paravertebral sympathetic chain interrupts visceral afferents and sympathetic efferent transmission. The specificity and sensitivity of this procedure and the role of the sympathetic nervous system in the pain of parturition is unclear (96). The precise mechanism of action of lumbar sympathetic blocks in labor is also unclear, given the close anatomic relationship between the sensory afferents and the sympathetic chain (see Fig. 39-4).
The lumbar sympathetic nerves at the thoracolumbar level are reasonably amenable to non–image guided regional anesthesia techniques. However, the afferent nerves from the distal one-third of colon and all the pelvic viscera are less so. These fibers travel with the sympathetic fibers of the superior hypogastric plexus, which is located retroperitoneally in the pelvis at the level of L5–S1. Needle placement is technically difficult and thus impractical to use during labor (97,98). In addition, use of fluoroscopic guidance to facilitate needle placement in the parturient would be exceptionally rare because of risks of fetal exposure to radiation (see Figs. 45-15, 45-16).
Lumbar sympathetic blockade requires placement of a 22-gauge 10-cm needle approximately 2 cm deep to the transverse process of L2, bilaterally. Incremental local anesthetic injections can be administered. Bilateral doses of 10 mL of bupivacaine 0.5% with 25 μg of fentanyl and 50 μg epinephrine have been used to good effect (95). Complications include bleeding, infection, hypotension, genitofemoral neuralgia, unintentional epidural/spinal injection, and perforation of viscera
or blood vessels (91,99). As previously mentioned, these complications, combined with the potential for block failure, have limited the clinical usefulness of these blocks.
or blood vessels (91,99). As previously mentioned, these complications, combined with the potential for block failure, have limited the clinical usefulness of these blocks.
Thoracolumbar Paravertebral Blockade
The paravertebral space is a narrow triangular space lateral to the vertebral column. The boundaries of the paravertebral space are formed by the iliopsoas anterolaterally; vertebral body, intervertebral disc, and foramen medially; and by the costotransverse ligament posteriorly. The space contains the sympathetic chain, dorsal and ventral nerve roots, and rami communicantes. Analgesia is achieved by deposition of local anesthesia in the distribution of the lateral aspect of the intervertebral foramen, within the paravertebral space, resulting in blockade of the ventral nerve roots and accompanying sympathetic nerves (see Figs. 16-4 and 16-15,16-16,16-17,16-18).
In the sitting position, the iliac crests (corresponding to L3–L4 ± 1 interspace) and spinous processes (midline) are identified and marked (see Chapter 16, Fig. 16-20). A line is drawn cephalad/caudad 2.5 cm lateral to midline, parallel to the spinous process of the thoracolumbar spine. Needle placement is along this line at the level of those nerve roots requiring blockade (T9–L1). Contact is made with the transverse process at each level required, and the needle is then angled 10 degrees cephalad/caudad and advanced 1 cm beyond the transverse process. Some anesthesiologists use a loss-of-resistance technique to identify the paravertebral space, although this can be quite subjective. This technique results in dermatomal analgesia at the level of the nerve roots blocked. The block is volume-dependent, and 20 mL of local anesthetic administered via a single needle should provide analgesia to approximately five somatic dermatomes and eight sympathetic levels. This volume of anesthetic agent may pose risks to the parturient in the event of accidental intravascular or intrathecal injection. Catheter techniques may be used to facilitate prolonged analgesia through the use of local anesthetic infusions and bolus doses as required. The absorption of local anaesthetic is difficult to predict, with analgesia of between 1 and 10 hours reported following a single injection (100,101) (see also Chapter 16).
The failure rate for paravertebral blocks performed in surgical patients is approximately 10%, with side effects including hypotension (4.6%), vascular puncture (3.8%), pleural puncture (1.1%), and pneumothorax (0.5%). The incidence of urinary complications and hypotension was found to be lower than with epidural analgesia (102,103). Total spinal anesthesia has also been reported as a complication (104). Paravertebral hematoma formation may also occur, although cadaveric studies suggest that fluid spread in the paravertebral space is caudal and lateral, thus away from the central neuraxis (105).
Bilateral lumbar sympathetic and paravertebral blocks have been described as effective techniques that can be used as an alternative to epidural labor analgesia (95,106). These, however, are non–image guided techniques (in the parturient), and the needle entry point is chosen on the basis of anatomic landmarks. The needle end-point is based on varying distances beyond contact with the transverse processes. This presents quite a large margin for error, and the distinction between the benefits of sympathectomy and nerve root block may be difficult to make for either procedure. These techniques have been suggested as suitable alternatives for epidural analgesia when central neuraxial blockade is deemed inappropriate for anatomic or medical reasons, but further investigation in the high-risk parturient is necessary. Use of short-acting IV opioid infusions may prove to be a safer and more flexible option.
Central Neuraxial Blockade
Epidural analgesia resulting in blockade of the lumbosacral plexus has been demonstrated to be a safe and effective technique for the management of labor pain in the parturient (107,108,109).
Epidural placement should be performed in an area with appropriate staffing, and familiarity with central neuraxial blockade is essential. Appropriate resuscitation equipment must be readily available. In addition, ongoing maternal and fetal monitoring should be maintained, and provision must be made for the placement of the epidural catheter in a sterile environment. Appropriate facilities for ongoing epidural management, including physiologic monitoring and post epidural management protocols, must be in place. There has been much discussion regarding both the proximity and levels of anesthesiology staffing required to provide safe and effective care using epidural analgesia in labor. International practice varies widely and, in the future, it is likely that a combination of quality assurance, financial constraints, and medicolegal issues may ultimately establish a more uniform standard of care.
Full preprocedure evaluation and preparation of the parturient should be performed. In addition, anesthesiologists are obliged to obtain consent and inform their patients of the risks associated with regional anesthesia and analgesia. The timing, effectiveness, and extent of this consent have been the subject of much debate (110). No clear consensus exists as to what the appropriate levels of informed consent are. Some anesthesiologists believe that the forum for obtaining and documenting consent for labor analgesia should be during the antenatal preparation of the parturient, as acute pain might have a marked impact on the understanding and acceptance of clinical risks (111). However, the consensus of opinion is that most women are capable of giving informed consent even during active labor. Theoretically, similar ethical problems may arise should a mother fail to give consent for epidural analgesia in the antenatal period, and then change this decision on experiencing acute pain refractory to parenteral opioids. The previous administration of parenteral opioids does not necessarily preclude subsequent placement of an epidural. However, each case must be decided individually. Anesthesiologists have differing views regarding the extent of inclusiveness of both risks and their associated incidences, and may limit patient discussion regarding rare complications such as epidural hematoma (112).
Patient Position
The technique of epidural insertion in labor differs in some ways from standard epidural placement in the nonpregnant patient. Pregnancy-related anatomic changes can make positioning and identification of landmarks problematic. In addition, ongoing acute pain and associated distress during contractions can lead to difficulty in positioning the patient and can lead to ongoing physical and emotional stress to both patient and medical staff. Epidural insertion can be performed with the patient in the lateral decubitus or sitting position. Some evidence suggests that uteroplacental perfusion may be optimum in the lateral decubitus position (113). Likewise, the potential for the reduction of venous congestion by adopting the lateral recumbent head-down position may be associated with a reduction in the incidence of lumbar epidural venous puncture (114). However, the incidence of successful epidural placement may be higher in the sitting position, especially in the obese parturient. In the management of obese and morbidly obese patients, the sitting position may be associated with easier identification
of the midline and possibly improved respiratory parameters (115). To complicate the issue further, a decreased incidence of aortocaval compression during the identification of the epidural space was demonstrated in the sitting position compared to the left lateral decubitus position (116). Taking all these factors into account, we believe that positioning of the patient for epidural placement should be done taking user experience and preference into account. In addition, patient factors and preferences should also be considered. One report even suggested that use of the prone (knee-chest) position had a role in epidural placement (117). There is no doubt that the dynamic nature of acute pain medicine requires that each practitioner should be familiar with a broad range of procedures and positions in the performance of epidural, spinal, and combined spinal–epidural techniques.
of the midline and possibly improved respiratory parameters (115). To complicate the issue further, a decreased incidence of aortocaval compression during the identification of the epidural space was demonstrated in the sitting position compared to the left lateral decubitus position (116). Taking all these factors into account, we believe that positioning of the patient for epidural placement should be done taking user experience and preference into account. In addition, patient factors and preferences should also be considered. One report even suggested that use of the prone (knee-chest) position had a role in epidural placement (117). There is no doubt that the dynamic nature of acute pain medicine requires that each practitioner should be familiar with a broad range of procedures and positions in the performance of epidural, spinal, and combined spinal–epidural techniques.
Aseptic Technique
Strict asepsis is essential in the performance of neuraxial blocks, especially those involving epidural catheter insertion. Several case reports have identified iatrogenic causes of meningitis during central neuraxial procedures (118,119,120,121). The routine use of face masks in the prevention of iatrogenic contamination by anesthesiologists has shown wide user variation. Surveys of United Kingdom obstetric anesthesiologists and of fellows of the Australia and New Zealand College of Anaesthetists with a special interest in obstetric practice showed a marked variation in practice standards. In the United Kingdom, more than 50% of anesthesiologists did not wear face masks for central neuraxial procedures, and this precaution was not seen to be essential in 29% of the latter group (122,123). The infectious disease community however, is united in their view that aseptic techniques, including use of face masks, is essential.
Disinfection of the skin with chlorhexidine, povidone iodine, or similar agent is strongly recommended. Standard aseptic precautions, including use of sealed bottles or single-use packets of povidone iodine, have proved to be more effective than multiuse bottles (124,125). In vitro studies have shown the effectiveness of iodine products for asepsis, although recent clinical evidence suggests that chlorhexidine in alcohol solution is more efficient as an antimicrobial agent (126). This combination agent has been extensively evaluated for use in placing central lines; however, its use in epidural or spinal placement has not been fully established. Although there is some worry about neurotoxicity based on preliminary animal research, clinical evidence in Europe suggests that chlorhexidine is both efficacious and safe for use as a disinfectant for neuraxial blocks (127,128).
Identification of the Epidural Space
Following appropriate antiseptic preparation of the lumbosacral spine, the skin is draped and local anaesthesia is administered to the skin and interspinal ligament. Use of clear plastic drapes offers the advantage of providing a sterile field while allowing better visualization of landmarks. Placement of the epidural needle at the L3–L4 ±1 intervertebral level should provide appropriate coverage of the lumbar and sacral nerve roots required for analgesia during labor and delivery. Use of loss-of-resistance techniques to both saline and air have been extensively described. Studies have assessed the quality of analgesia in women randomized to either technique (129,130): Beilin and co-workers found that patients who had epidural placement using a loss-of-resistance technique to air had a higher requirement for rescue medications following initial analgesia (129). The merits of a saline technique include avoidance of pneumocephalus-induced headache, nonuniform spread of local anesthetic, and nerve root irritation, all of which have been described following injection of epidural air (131,132) (see Figs. 11-12,11-13,11-14,11-15).
However, the judicious use of air during the loss-of-resistance technique should avoid many of these side effects. In fact, the use of air when performing a combined spinal–epidural (CSE) technique can be advantageous, as it allows clear identification of cerebrospinal fluid (CSF) without introducing any confusion caused by the concomitant use of saline. Because of the unpredictable duration of labor and the ever-present need to facilitate cesarean section delivery should it be necessary, placement of the epidural catheter is performed more commonly than performing a single-injection technique.
Selection of Epidural Catheter
The use of both single-port (uniport) and multiport (multiple-orifice) epidural catheters has been widely described. The proposed advantage of the single-port (open-end) catheter is the delivery of medication to a single anatomic site, which may have safety implications. However, single-port catheters may also be associated with reduced spread of medication, leading to incomplete and/or unilateral blocks. This problem may be reduced through the use of the newly developed flexible-tip single-port catheters, which may also potentially offer the advantage of decreased incidence of paresthesias and intravascular placement (133).
Comparisons between multi- and single-port catheters showed that significantly fewer catheters needed replacement in the multiport group because of inadequate analgesia (defined as unblocked segments or unilateral block) and that paresthesias were less common in this same group (134,135). A comparison of multiport, firm-tipped, close-ended epidural catheters with uniport, open-ended, soft-tipped, wire-reinforced catheters showed the softer uniport to have a lower incidence of paresthesias and vascular puncture (136).
Multiport (closed-end) catheters have the theoretical disadvantage of potentially delivering anesthetic agent to more than one anatomic site, such as to the epidural and subarachnoid spaces at the same time. However, studies have not shown multiport catheters to be associated with multicompartment placement, and the incidence of vascular puncture and dislodgement requiring replacement were similar for both catheter types (single- and multiport). Multiport catheters have consistently been shown to be associated with a reduced incidence of inadequate analgesia and thus require less manipulation, presumably because of a more even distribution of medication (133,135).
A further technique suggested in the prevention of accidental vascular puncture during epidural catheter placement includes injection of 3 to 5 mL of saline through the epidural needle prior to advancing the catheter. This is thought to expand the epidural space, possibly decreasing the likelihood of unintentional IV cannulation (137).
Technical factors may play a major role in determining the effectiveness of labor analgesia. In a study of 100 laboring women, insertion of multiport catheters to a depth of 5 cm was shown to be associated with the highest incidence of satisfactory analgesia and minimal complications, compared to a higher rate of insertion complications at a 7-cm depth (138). Cephalad orientation of the epidural needle at catheter placement has also been shown to be associated with increased effectiveness and reduced complications. Epidural catheters are not fixed in the epidural space however, and their position can vary according to the posture of the parturient. Change of position from sitting to lateral recumbent can be associated with
movement of the catheter of between 1 and 2.5 cm, which can lead to inadequate analgesia (139). To minimize the risk of catheter displacement, especially in obese patients, it has been suggested that multiorifice catheters should be inserted to a depth of more than 4 cm into the epidural space and secured only upon assuming the lateral position (140).
movement of the catheter of between 1 and 2.5 cm, which can lead to inadequate analgesia (139). To minimize the risk of catheter displacement, especially in obese patients, it has been suggested that multiorifice catheters should be inserted to a depth of more than 4 cm into the epidural space and secured only upon assuming the lateral position (140).
Epidural Test Dose
Confirmation of successful catheter placement within the epidural space is often best measured by effective analgesia. Prior to administration of appropriate medications to achieve this degree of analgesia, unanticipated intrathecal or IV catheter placement must be excluded. Although this may not ensure appropriate epidural placement, it does however reduce the cardiorespiratory and neurologic risks associated with administration of a dose intended for epidural use into the CSF or systemic circulation. The use of test doses in labor has also been the subject of much controversy. Use of an appropriate test dose is considered important by some anesthesiologists, especially as many patients may subsequently require larger doses of concentrated local anesthetic administered epidurally in the event of emergency cesarean delivery (141). The sensitivity and specificity of test dosing is critically important in determining the appropriate placement of an epidural catheter. Given the extent of patient movement during labor and the potential for the epidural catheter to move according to patient position, it could be argued that frequent test dosing might be required. In addition, the impact of this epidural test dose on motor function in ambulating patients, and in preeclamptic patients and patients with cardiac disease, should also be considered (142,143,144,145,146). However, in clinical practice, if an epidural catheter has been placed and local anesthesia/opioid solution is infusing uneventfully into a comfortable parturient with minimal motor deficit, the catheter can be considered to be appropriately sited in the epidural space.
Intrathecal injection of 3 mL of lidocaine 1.5% (45 mg) with epinephrine 1:200,000 (5 μg/mL) will result in dense motor blockade within 2 to 4 minutes. Intravascular injection of 15 μg of epinephrine will result in tachycardia. The administration of this dose of local anesthetic systemically can result in lightheadedness and circumoral numbness or tingling within 1 minute of injection (141,142,143). Administration of the test dose should not be performed immediately before or during a uterine contraction, as labor pain itself is associated with increased heart rate variability. Aspiration of epidural catheters should be performed, but does not adequately identify all cases of intravascular or intrathecal catheter placement, especially if a single-port catheter is used (147,148).
Use of the “air test” combined with epidural catheter aspiration has also been described as a method to out rule accidental intravascular catheter placement (149). Injection of 1 mL of epidural air with concomitant precordial Doppler detection has been used successfully to identify intravascular placement. However, the sensitivity for this test appears to be less with use of the newer multiport epidural catheters (150).
Selection of Local Anesthetic Agent and Adjuvant
Bupivacaine
The use of the amide local anesthetic bupivacaine is well established in obstetric anesthesia. Its prolonged duration of action, differential sensory blockade, and relative lack of tachyphylaxis make it an ideal agent for use in epidural and spinal anesthesia (151). The degree of drug ionization at physiologic pH and the extent of protein binding determine the degree of placental transfer. Bupivacaine is highly ionized at physiologic pH (pKa of 8.05) and is 95% protein bound, thus placental transfer is limited. The ratio at delivery of the concentration of local anesthetic in blood or plasma from the umbilical vein to the concentration of local anesthetic in maternal blood (UV:MA ratio) for bupivacaine ranges from 0.31 to 0.44 and is much lower than that of lidocaine (152). Bupivacaine has been the subject of concern in relation to its systemic cardiovascular toxicity (153,154), and the use of bupivacaine 0.75% concentration solution in the epidural space has been prohibited in obstetric practice by the U.S. Food and Drug Administration (FDA). Bupivacaine consists of two stereoisomers S(–) and R(+), and is marketed as a racemic mixture of these. TheR enantiomer was found to contribute to bupivacaine’s unwanted toxicity (155,156). Levobupivacaine and ropivacaine are the pure S(–) enantiomers of N-butyl- and N-propyl-2′,6′-pipercoloxylidide, which were developed as less cardiotoxic alternatives to bupivacaine (157) (see also Chapter 3).
Ropivacaine
Ropivacaine is a homologue of bupivacaine and mepivacaine formulated as a single levo rotary enantiomer. The putative advantages of ropivacaine over bupivacaine are the suggested lower risks of cardiovascular depression and neurologic toxicity (158) (see also Chapter 3).
In addition, sheep studies have shown faster clearance associated with ropivacaine compared to bupivacaine following intravascular administration, which suggests a greater margin of safety after unintentional intravascular injection (159).
Use of the minimum local anesthetic concentration (MLAC) in determining local anesthetic potency has shown that ropivacaine is 40% less potent than bupivacaine when used as a bolus for initiation of epidural analgesia in early labor (158,160). However, dilute ropivacaine (0.08%) with fentanyl (2 μg/mL) produced effective labor analgesia with preservation of spontaneous voiding and ambulation in parturients (161). Comparison between bupivacaine 0.075% and ropivacaine 0.075% using a patient-controlled epidural analgesia (PCEA) technique during labor showed both agents to be equally effective (162). Thus, there appears to be no clear clinical advantage of ropivacaine over bupivacaine for epidural labor analgesia in terms of either obstetric or neonatal outcome. However, cost may become a factor in the selection of any anesthetic technique, and ropivacaine is currently more expensive than racemic bupivacaine (163,164,165).
Levobupivacaine
Levobupivacaine is a long-acting local anesthetic with a similar clinical profile to bupivacaine. Studies have shown that the cardiotoxicity of bupivacaine was more pronounced with the R(+) enantiomer, and use of levobupivacaine—the S(-) enantiomer—has gained acceptance in clinical practice (166). The safety of levobupivacaine has been compared to racemic bupivacaine in both animal and human volunteer studies (167,168,169). The lethal dose of levobupivacaine was 1.3- to 1.6-fold higher than that of bupivacaine in several animal studies, supporting the added safety of levobupivacaine should inadvertent intravascular injection occur (170) (see also Chapters 3 and 5).
Levobupivacaine crosses the placenta, with UV:MA ratios for levobupivacaine demonstrated to be 0.3 in women undergoing elective cesarean delivery following the epidural administration of 30 mL 0.5% levobupivacaine (171).
Levobupivacaine has been shown to compare favorably to bupivacaine for epidural use for cesarean delivery (172). Assessment of speed of onset and quality of sensory block between 0.5% bupivacaine and 0.5% levobupivacaine has shown similar results. There appears to be no difference between speed of onset and duration of sensory block when patients received either levobupivacaine 0.5% or bupivacaine 0.5% for cesarean delivery. In addition, the onset and reversal of sensory/motor blockade, quality of anesthesia, and muscle relaxation were comparable between the two groups. Levobupivacaine has also been used extensively to provide epidural analgesia for labor. A recent multicenter study comparing levobupivacaine and bupivacaine reported these drugs as having equivalent analgesic efficacy (173,174). Concomitant use of fentanyl has been shown to have a dose-sparing effect on levobupivacaine requirements. Levobupivacaine is not currently marketed in the United States, but is available in many other countries worldwide.
Levobupivacaine has been shown to compare favorably to bupivacaine for epidural use for cesarean delivery (172). Assessment of speed of onset and quality of sensory block between 0.5% bupivacaine and 0.5% levobupivacaine has shown similar results. There appears to be no difference between speed of onset and duration of sensory block when patients received either levobupivacaine 0.5% or bupivacaine 0.5% for cesarean delivery. In addition, the onset and reversal of sensory/motor blockade, quality of anesthesia, and muscle relaxation were comparable between the two groups. Levobupivacaine has also been used extensively to provide epidural analgesia for labor. A recent multicenter study comparing levobupivacaine and bupivacaine reported these drugs as having equivalent analgesic efficacy (173,174). Concomitant use of fentanyl has been shown to have a dose-sparing effect on levobupivacaine requirements. Levobupivacaine is not currently marketed in the United States, but is available in many other countries worldwide.
Lidocaine
Lidocaine has been widely used in obstetric anesthesia. The rapid onset of action associated with lidocaine and its intermediate duration of action are particularly useful for many obstetric procedures. The UV:MA ratios reported are within the range of 0.4 to 0.6 (175). Epidurally administered lidocaine 2% solution combined with epinephrine is widely used for operative delivery; however, its duration of action and degree of motor blockade make it less ideal for use in facilitation of prolonged labor analgesia. Although early reports suggested that lidocaine compromised neonatal neurobehavioral function, this has not been borne out by further investigation or clinical experience (176,177).
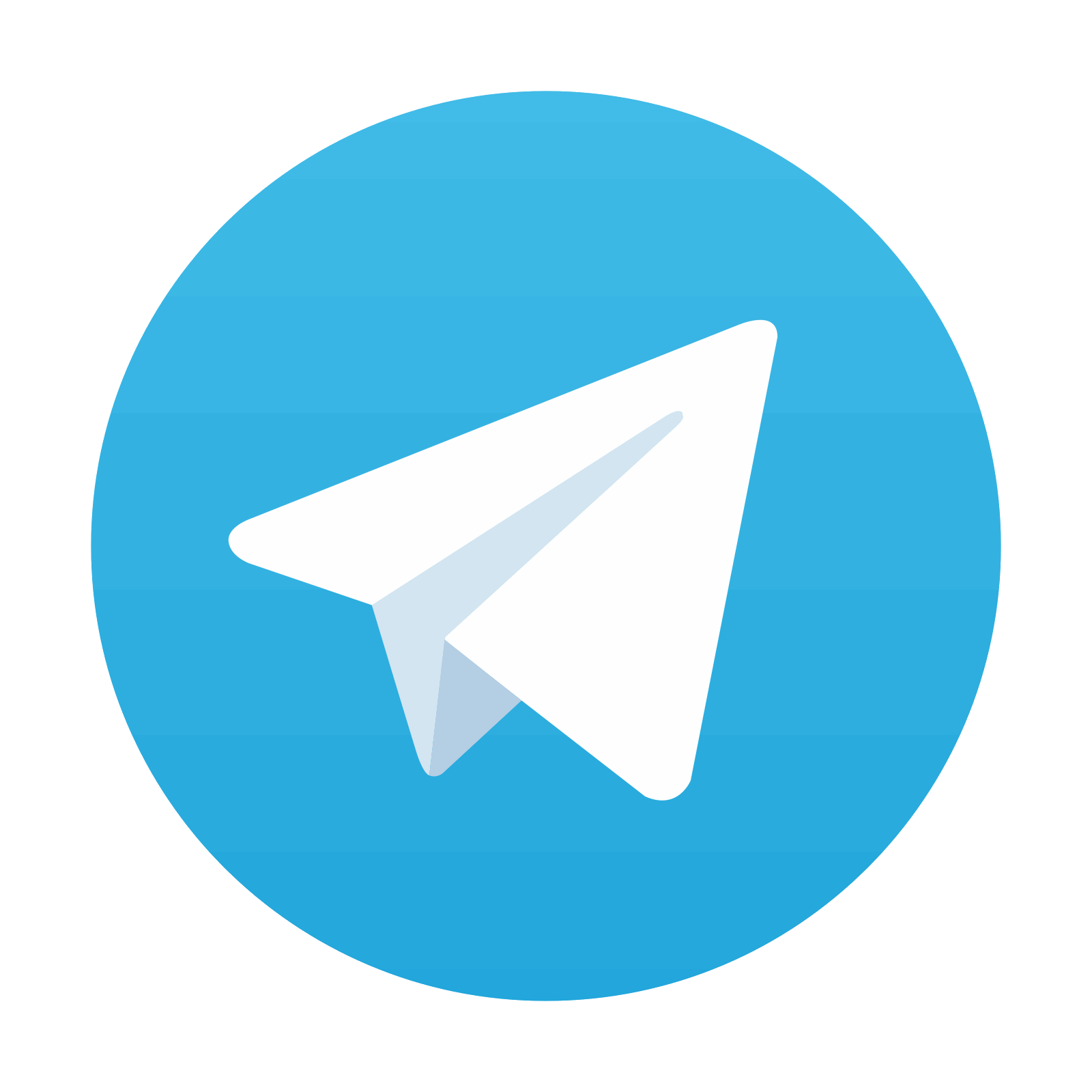
Stay updated, free articles. Join our Telegram channel

Full access? Get Clinical Tree
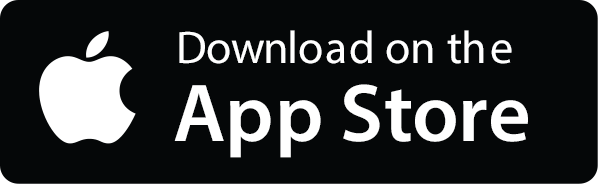
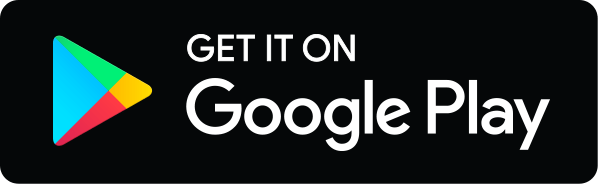
