Neonatal Resuscitation
Emily J. Baird
Richard C. Month
Valerie A. Arkoosh
It is estimated that over 100 million babies are born annually worldwide. The successful transition from fetus to neonate hinges on the ability of the newborn to adapt to extrauterine life; this transition is characterized by numerous physiologic changes that must rapidly transpire following delivery. Despite the complexity of this process, only 10% of newborns require assistance with ventilation at birth and 1% require extensive resuscitative measures (Fig. 16-1) (1). Nonetheless, this translates to an estimated 5 to 10 million interventions at birth annually. In order to anticipate the need for resuscitation, and to have both the provisions and knowledge to respond appropriately, it is imperative that all providers in the labor and delivery suite understand neonatal adaptation to extrauterine life, the changes that occur during transition from fetal to adult circulation, and the physiology of fetal asphyxia.
Neonatal Adaptations to Extrauterine Life
Fetal Cardiovascular and Pulmonary Physiology
Unlike in the adult, where pulmonary and systemic circulations operate in parallel, the fetal circulation operates in series (Fig. 16-2) (2). In utero, oxygenated blood from the placenta returns via the umbilical vein to the portal vein and is shunted through the ductus venosus into the inferior vena cava (IVC). Nearly 40% of IVC return is directed across the foramen ovale, through the left heart and ascending aorta, to perfuse the heart and brain with maximally oxygenated blood (3). Deoxygenated blood from the head and upper extremities enters the heart through the superior vena cava (SVC). This blood is preferentially directed into the right ventricle, where it mixes with the remainder of the oxygenated IVC return. Due to high pulmonary vascular resistance (PVR), 90% of right ventricular outflow shunts across the ductus arteriosus to the descending aorta to perfuse the abdomen, pelvis, and lower extremities (4). 40% of cardiac output traverses the low-resistance placenta; this contributes to overall low systemic vascular resistance (SVR).
Fetal lung development takes place throughout pregnancy and continues into the neonatal period. In fact, the newborn lung contains only one-third the number of alveoli present in the mature lung, and the process of alveolar development continues through the first 18 months of life (5).
The development of surfactant is also a late occurrence in fetal lung development. Pulmonary surfactant is responsible for reducing alveolar surface tension and allowing for successful ventilation and gas exchange. It is produced by type II pneumocytes in the distal airways, and is typically measurable in fetal lung tissue by 20 weeks of gestation. However, it is not present in the airway lumen until 28 to 32 weeks of gestation, and it is not present in adequate quantity until 34 to 38 weeks of gestation (5).
Normal Peripartum Transition to Extrauterine Life
Parturition leads to rapid and profound changes in neonatal hemodynamics and pulmonary mechanics. During development, the fetal airways contain approximately 30 mL/kg of fluid; during labor, this ultrafiltrate of plasma begins to reabsorb (3). During vaginal delivery, compression of the infant thorax expels further fluid from the mouth and upper airways (2). With the first breath, the lungs fill with air, surfactant is released, and oxygenation is increased (6). This increase in oxygen tension and blood flow has been shown to increase the release of nitric oxide in the pulmonary vasculature, resulting in pulmonary vasodilation and a substantial decrease in PVR (7). Simultaneously, clamping of the umbilical cord removes the low-resistance placenta from the systemic circulation substantially increasing SVR, left atrial pressure increases, and flow through the foramen ovale ceases. Functional closure of the foramen ovale occurs rapidly after birth as left atrial pressures begin to exceed right atrial pressures. The net result is a dramatic reduction, and eventual cessation, of the fetal right-to-left shunt. Right-to-left shunt across the ductus arteriosus reduces substantially within the first few minutes of life. However, anatomic closure of the foramen ovale takes several months; therefore, any circulatory disturbance that elevates right atrial pressure may cause the foramen to remain open, shunting venous flow from right to left and leading to cyanosis.
Prolonged Hypoxia/Acidosis and Failure of Transition: Asphyxia in the Newborn
Transient hypoxemia or acidosis is typically well tolerated by a normal newborn and prompt resuscitation usually prevents permanent physiologic alteration. However, prolonged neonatal
hypoxemia or acidosis impedes the transition from fetal to neonatal physiology. Hypoxemia and acidosis promote pulmonary hypertension through hypoxic pulmonary vasoconstriction; this encourages continued flow across the ductus arteriosus. Hypoxia also directly promotes the patency of the ductus arteriosus, as ductal smooth muscle constriction is dependent on arterial oxygen tension. Pulmonary hypertension causes elevated right heart pressures, maintaining right-to-left shunt across the foramen ovale. Blood flowing through the patent ductus arteriosus (PDA) and foramen ovale is not oxygenated, contributing to increasing hypoxemia (4). Progressive hypoxemia and acidosis ultimately results in myocardial failure and brain damage.
hypoxemia or acidosis impedes the transition from fetal to neonatal physiology. Hypoxemia and acidosis promote pulmonary hypertension through hypoxic pulmonary vasoconstriction; this encourages continued flow across the ductus arteriosus. Hypoxia also directly promotes the patency of the ductus arteriosus, as ductal smooth muscle constriction is dependent on arterial oxygen tension. Pulmonary hypertension causes elevated right heart pressures, maintaining right-to-left shunt across the foramen ovale. Blood flowing through the patent ductus arteriosus (PDA) and foramen ovale is not oxygenated, contributing to increasing hypoxemia (4). Progressive hypoxemia and acidosis ultimately results in myocardial failure and brain damage.
Both the fetus and neonate respond to hypoxemia with a “diving” reflex (known as such due to its similarity to seal physiology during a dive). Blood flow is diverted centrally to
the heart, brain, and adrenal glands, and tissue oxygen extraction maximally increases. Although initially a hypertensive response, as oxygen extraction reaches its maximum, myocardial contractility and cardiac output decrease, leading to systemic hypotension (4).
the heart, brain, and adrenal glands, and tissue oxygen extraction maximally increases. Although initially a hypertensive response, as oxygen extraction reaches its maximum, myocardial contractility and cardiac output decrease, leading to systemic hypotension (4).
Neonatal ventilation during hypoxia is initially rapid and regular. However, as hypoxia continues, respiration stops in a stage termed primary apnea. Stimulation of the neonate during primary apnea will reinitiate respiratory effort. If hypoxia continues, the neonate will begin to attempt irregular, grunting respirations, followed by another cessation of respiration called secondary or terminal apnea. Stimulation will not reverse secondary apnea, since respiratory drive has been reduced by both central nervous system (CNS) depression and direct diaphragmatic depression (8). The net result of these physiologic responses is a neonate with persistent pulmonary hypertension and little or no ventilatory drive. Ideally, prompt resuscitation prevents these physiologic perturbations.
Anticipating the Depressed Newborn
Approximately 80% of neonates requiring resuscitation can be anticipated (1,9). Many of the antepartum and intrapartum factors related to increased likelihood for neonatal resuscitation are listed in Table 16-1.
Antepartum Evaluation
The contraction stress test (CST), originally called the oxytocin challenge test (OCT), was one of the first antepartum tests aimed at assessing fetal well-being (10). Intravenous oxytocin or maternal nipple stimulation is used to induce at least three moderate to strong uterine contractions per 10-minute period for at least 20 minutes. The outcomes of the test are summarized in Table 16-2 (10). Indeterminate or suspicious tests are usually repeated within 24 hours. Positive CST has been shown to correlate well with intrauterine growth restriction, increased incidence of late decelerations in labor, and depressed 5-minute Apgar score (11). The CST has been proven to have a high negative predictive value, with false-negative rates less than 0.4/1,000 patients (12). However, as with most antenatal testing modalities, the false-positive rate remains high at approximately 30% (11).
The biophysical profile (BPP) provides further antepartum evaluation of the fetus. The BPP consists of four ultrasound components and one cardiotocographic component (the nonstress test [NST], which can be used by itself as a screening test) as listed in Table 16-3. Each is scored either zero or two for a final maximum score of ten points (13). A BPP score of 8/10, with normal amniotic fluid volume, is considered reassuring (13), while a BPP score of 6/10 (or 8/10 with abnormal amniotic fluid volume) correlates closely with fetal asphyxia.
The BPP accurately identifies both acute hypoxia (manifested by NST, movement, and breathing) and chronic hypoxia (manifested by reflex movements and amniotic fluid volume) (14). It identifies asphyxiated fetuses in several different high-risk patient populations, including many of those listed in Table 16-1 (15,16,17). While the test carries a false-negative rate of only 0.7 in 1,000 examinations, the false-positive rate, with a 1-week interval examination period, is approximately 50% (18,19).
The BPP is labor intensive, requiring a continuous 30-minute ultrasound period. In an effort to streamline the test, an indicator of acute hypoxia (NST) and an indicator of chronic hypoxia (amniotic fluid volume) were combined as a first-line test (20). This modified biophysical profile (mBPP) is then followed by a complete BPP if either component is abnormal. This progression of examinations has been a successful compromise for the labor-intensive BPP (12,17).
Table 16-1 Antepartum and Intrapartum Factors Associated with the Need for Neonatal Resuscitation | ||||||||||||||||||||||||||||||||||||||||
---|---|---|---|---|---|---|---|---|---|---|---|---|---|---|---|---|---|---|---|---|---|---|---|---|---|---|---|---|---|---|---|---|---|---|---|---|---|---|---|---|
|
Intrapartum Evaluation
The primary method of intrapartum evaluation of the fetus continues to be fetal heart rate (FHR) monitoring by cardiotocography. Over time, this has proven to be a consistently reliable method of confirming fetal well-being; a reassuring FHR tracing is more than 90% accurate in identifying a neonate with a 5-minute Apgar score of 8 or higher (21). However, FHR monitoring carries a false-negative rate as high as 50% (21). An abnormal FHR tracing may not be indicative of long-term outcomes; however, it is highly predictive of the need for resuscitative efforts in the delivery room (9).
Intrapartum fetal monitoring utilizes FHR measurement (by external Doppler or by fetal scalp electrode) in concert with measurement of contraction pattern (by external tocodynamometry or intrauterine pressure transducer). These two parameters, together, can suggest fetal well-being; they
can also be a harbinger of fetal asphyxia. Four specific parameters are evaluated when assessing the FHR tracing: Baseline, variability, accelerations, and decelerations (22). Definitions of each parameter are in Table 16-4.
can also be a harbinger of fetal asphyxia. Four specific parameters are evaluated when assessing the FHR tracing: Baseline, variability, accelerations, and decelerations (22). Definitions of each parameter are in Table 16-4.
Table 16-2 Outcomes of the Contraction Stress Test | ||||||||||||||
---|---|---|---|---|---|---|---|---|---|---|---|---|---|---|
|
Utilizing these four parameters, FHR tracings can be placed into three categories, as identified in Table 16-5. Category I tracings are classified as normal, and are indicative of fetal well-being. Category II tracings are classified as indeterminate, and are neither indicative of fetal well-being nor predictive of abnormal fetal acid–base status. These tracings require consistent reevaluation and surveillance, taking into account the entire clinical picture. Category III tracings are abnormal and are highly predictive of abnormal fetal acid–base status. Category III tracings should be met with prompt evaluation and intervention (22). In addition, the presence of a sinusoidal FHR pattern, defined as a tracing with a smooth, sine-wave–like pattern with three to five cycles per minute, persisting 20 minutes or more, necessitates prompt intervention (22).
Table 16-3 Biophysical Profile | ||||||||||||
---|---|---|---|---|---|---|---|---|---|---|---|---|
|
In the presence of a Category II or III FHR tracing, a confirmatory test of fetal well-being is often prudent. Fetal pH can be measured directly via scalp puncture and can directly confirm or exclude acidosis (23). A scalp pH below 7.20 is considered abnormal and, if confirmed by a second measurement, may indicate the need for expedient delivery. Fetal scalp sampling is not without its challenges; it is an invasive examination for both mother and fetus and requires ruptured membranes to gain access to the fetus. For these reasons, many labor and delivery units have decreased or discontinued the use of fetal scalp sampling and replaced it with digital scalp stimulation (24).
Digital scalp stimulation is administered by gently rubbing the fetal scalp for 15 seconds during a vaginal examination. A FHR acceleration of 10 bpm for 10 seconds or longer after stimulation is highly predictive of a fetal pH greater than 7.20. A lack of acceleration after scalp stimulation, however, is predictive of fetal acidosis with a likelihood ratio greater than 15.
Evaluating the Neonate
The Apgar Score
The cornerstone of neonatal evaluation for the past 50 years has been the Apgar score. The score, first published by anesthesiologist Virginia Apgar in 1953, uses five signs: Heart rate, respiratory effort, reflex irritability, muscle tone, and color, each on a 0 to 2 point scale, for a maximum score of 10 (25). These scores are typically measured at 1 minute and 5 minutes postpartum. The individual parameters are listed in Table 16-6.
This simple scoring system, specifically the 5-minute Apgar score, has repeatedly proven useful in predicting neonatal mortality: The term infant with a 5-minute Apgar score of 0 to 3 is 59 to 386 times more likely to die in the neonatal period than the term infant with a score of 7 to 10 (26,27).
However, the Apgar score is not without concerns. There is high inter-observer variation in scoring, both in the total score and in each of the subcategories (28). In addition, the scores have been used incorrectly in an attempt to identify long-term outcomes beyond the increased risk of neonatal death (including long-term neurologic function, cerebral palsy, and even future intelligence). Incorrect Apgar scoring has led to issues of increased medicolegal liability. According to the American Academy of Pediatrics (AAP) and the American College of Obstetricians and Gynecologists (ACOG), the Apgar scoring system, while shown to correlate with neonatal death, is not “[a] conclusive marker … of an acute intrapartum hypoxic event” (29).
Umbilical Arterial and Venous Blood Gas Analysis
In addition to the Apgar score, umbilical cord blood gas measurements can further elucidate the intrauterine environment of the fetus. Normal umbilical arterial and venous blood gas measurements are found in Table 16-7; however, wide variation can be found in vigorous neonates with Apgar scores between 7 and 10 (30). Cord gas measurements are extracted from umbilical artery and vein samples from a 20 to 30 cm section of cord immediately after cord clamp (30).
Table 16-4 Interpretation of Fetal Heart Tracing | ||||||||||
---|---|---|---|---|---|---|---|---|---|---|
|
Table 16-5 Categorization of Fetal Heart Rate Tracing | ||||||||
---|---|---|---|---|---|---|---|---|
|
These two measurements provide different information, and together can provide a picture of the peripartum milieu of the fetus, and may be able to help explain reasons for fetal distress. The umbilical venous sample provides an indication of adequacy of placental transfer, in addition to indirect measurements of maternal and fetal status, as this is blood traveling directly from the placenta. Conversely, the umbilical arterial sample provides a direct measurement of fetal oxygenation and acid–base status, and is analogous to a central venous sample in an adult.
While much information can be gleaned from a solitary umbilical arterial sample, and, should only one of the two samples be collected, it should be an arterial sample, much more data can be extracted from a paired sample (31). First, the two components of a paired sample are always identifiable as arterial and venous, respectively, as a venous cord sample will invariably have a higher pH and higher PO2 than the coinciding arterial sample. Second, while a solitary arterial sample can identify fetal acidosis and quantify its degree, a paired sample can assist in identifying the etiology of acidosis. Fetal asphyxia due to maternal hypoxia or uteroplacental insufficiency will present as both arterial and venous
hypoxia and acidemia; inadequate oxygenation of placental blood leads to inadequate tissue oxygenation in the fetus. On the other hand, fetal asphyxia due to umbilical cord compression will lead to solitary umbilical arterial acidemia; umbilical venous blood has prolonged gas exchange time (leading to higher PO2 and pH) and does not reach the fetus, leading to acidosis (31). The cord pH arteriovenous difference can be used as an indication of placental insufficiency versus cord compression as an etiology of fetal acidosis; an arteriovenous pH difference of greater than 0.15 units has been shown to be an effective cutoff between the two etiologies (32).
hypoxia and acidemia; inadequate oxygenation of placental blood leads to inadequate tissue oxygenation in the fetus. On the other hand, fetal asphyxia due to umbilical cord compression will lead to solitary umbilical arterial acidemia; umbilical venous blood has prolonged gas exchange time (leading to higher PO2 and pH) and does not reach the fetus, leading to acidosis (31). The cord pH arteriovenous difference can be used as an indication of placental insufficiency versus cord compression as an etiology of fetal acidosis; an arteriovenous pH difference of greater than 0.15 units has been shown to be an effective cutoff between the two etiologies (32).
Table 16-6 Apgar Scoring System | ||||||||||||||||||||||||||||
---|---|---|---|---|---|---|---|---|---|---|---|---|---|---|---|---|---|---|---|---|---|---|---|---|---|---|---|---|
|
Resuscitation of the Newborn
Although the majority of deliveries are uneventful, approximately 10% of newborns require some assistance in making the transition to extrauterine life. Because of the precarious physical status of the newborn, it is imperative that all providers working within labor and delivery units are familiar with neonatal resuscitation.
Neonatal resuscitation should not only save life but also prevent the sequelae of acute asphyxia, including hypoxic–ischemic encephalopathy, cerebral palsy, cognitive impairment, multiorgan failure, bone marrow depression, disseminated intravascular coagulation, and asphyxial cardiomyopathy. The degree of organ damage and potential for full recovery depends on the severity and duration of the asphyxial event as well as the speed of successful resuscitative efforts.
Steps in Initial Resuscitation
Personnel: Every labor and delivery unit should have a list of both fetal and maternal conditions that require the presence of a dedicated neonatal resuscitation team at delivery. According to the American Society of Anesthesiologists’ practice guidelines, this team should be available for any delivery in which fetal compromise is anticipated (33). On the resuscitation team, each member must be assigned individual tasks including airway management, placement of monitors, insertion of lines, drawing up of medications, and charting. While an anesthesiologist may be invaluable in managing difficult intubation and ventilation, the resuscitation team should not include the anesthesiologist attending the mother, though she/he may provide brief assistance if care of the mother is not compromised (34). Additional personnel such as respiratory therapists with newborn resuscitation experience can be a very useful part of the team. Given the urgent nature of neonatal resuscitation, preparation, including practice drills, is an integral aspect of labor floor preparedness.
Table 16-7 Normal Umbilical Arterial and Venous Blood Gas Values | ||||||||||||||||||
---|---|---|---|---|---|---|---|---|---|---|---|---|---|---|---|---|---|---|
|
Equipment: Standard neonatal resuscitation equipment and medications should be organized in a central location, checked frequently for proper functioning and expiration date, and replenished immediately after use (Table 16-8). Suction, oxygen, compressed air, and an air–oxygen blender should be immediately available. In addition, a source of radiant heat should be attached to the resuscitation bed and controlled by a sensor taped to the infant’s abdomen.
Neonatal Resuscitation Protocol: The American Heart Association (AHA) and the AAP endorse a neonatal resuscitation protocol based on frequent assessment of the newborn and escalating levels of intervention (Fig. 16-3) (35). The algorithm is organized into 30-second periods in which an intervention is completed, the neonate is reevaluated, and the decision is made whether to progress to the next step. Assessment of the neonate focuses on evaluation of the heart rate and respirations. Successful completion of the previous step is a prerequisite to proceed to the next intervention.
The general condition of the neonate is quickly assessed with three screening questions addressed immediately following delivery: Is the infant full-term? Is the newborn breathing or crying? Does the infant have good muscle tone? Routine care is provided for full-term newborns with good muscle tone and breathing without distress. Neonates with difficulty making the transition to extrauterine life should be warmed, gently stimulated, and placed in the “sniffing position” to open the airway. Suctioning of the pharynx and nose should be brief and gentle. Prolonged or vigorous suctioning may result in breath holding, laryngospasm, and/or bradycardia.
Support of Thermal Stability
Minimizing heat loss is an integral part of neonatal resuscitation. Depressed and asphyxiated infants often have an unstable thermal regulatory system. The large surface area
to body mass ratio of the neonate facilitates rapid heat loss by conduction, convection, evaporation, and radiation. The term neonate’s primary defense against hypothermia is catecholamine-mediated metabolism of brown fat. Nonshivering thermogenesis, in turn, leads to an increase in oxygen consumption, calorie utilization, and metabolic rate (36). The resulting hypoxemia, hypercarbia, and hypoglycemia promote persistence of the fetal circulation and hinder resuscitation.
to body mass ratio of the neonate facilitates rapid heat loss by conduction, convection, evaporation, and radiation. The term neonate’s primary defense against hypothermia is catecholamine-mediated metabolism of brown fat. Nonshivering thermogenesis, in turn, leads to an increase in oxygen consumption, calorie utilization, and metabolic rate (36). The resulting hypoxemia, hypercarbia, and hypoglycemia promote persistence of the fetal circulation and hinder resuscitation.
Table 16-8 Essential Supplies for Neonatal Resuscitation | ||||||||||||||||||||||||||||||||||
---|---|---|---|---|---|---|---|---|---|---|---|---|---|---|---|---|---|---|---|---|---|---|---|---|---|---|---|---|---|---|---|---|---|---|
|
Assisted Ventilation
Spontaneously breathing infants generally make their first respiratory efforts seconds after delivery of the thorax. These neonates generate a negative intrathoracic pressure of 60 to 100 cm H2O and inspire approximately 80 mL of air with their first breath. The majority of air in the first breath is retained within the lungs as part of the developing functional residual capacity (37). Infants who fail to initiate respirations with gentle stimulation, warming, and airway suctioning require further intervention to ensure adequate ventilation.
Assisted ventilation is required in about 3% to 5% of all newborn infants (Fig. 16-1). Positive pressure ventilation is indicated in neonates who remain apneic 30 seconds after delivery, have ineffective or gasping ventilation, and/or a heart rate less than 100 bpm (Fig. 16-3). Assisted ventilation can be initiated with a bag–mask device, a laryngeal mask airway (LMA), or an endotracheal intubation. Traditionally, ventilation is established with a bag–mask device and then converted to an endotracheal tube in the absence of clinical improvement.
Endotracheal Intubation
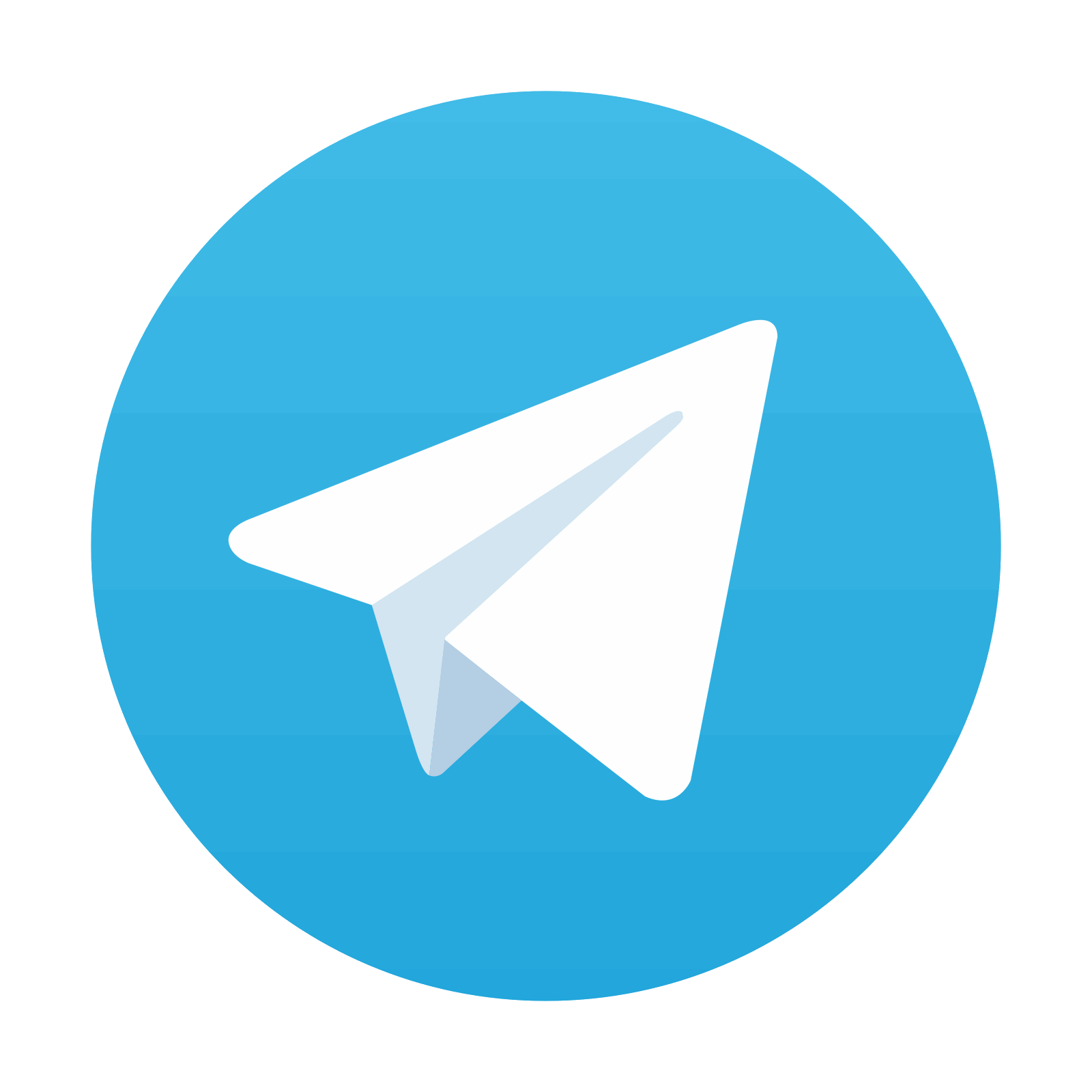
Stay updated, free articles. Join our Telegram channel

Full access? Get Clinical Tree
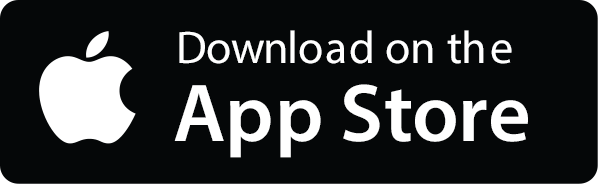
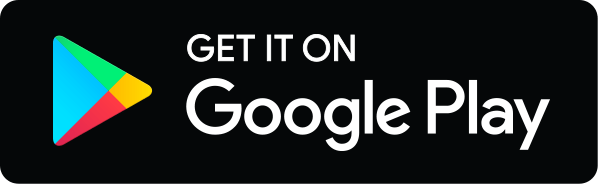