Macroscopic structure of skeletal muscle
Microscopic structure
Each muscle cell runs the length of the muscle belly and is polynucleate. The vast majority of muscle cells are innervated by a single nerve ending, located at the centre of the muscle belly. The cell wall of the muscle cell is called the sarcolemma, which contains both the lipid bilayer membrane and an outer layer of polysaccharide and collagen which fuse together at the ends of the fibre with the tendons. Within the sarcolemma, each muscle fibre contains hundreds of myofibrils suspended in the specialised cytoplasm called sarcoplasm. The sarcoplasm is notable for containing the large numbers of mitochondria needed to power muscle contraction, and the sarcoplasmic reticulum. The sarcoplasmic reticulum is a specialised organelle and provides an intracellular store of calcium ions. It is wound tightly around the myofibrils. Long invaginations of the sarcolemma called T tubules penetrate deep into the cell and provide a mechanism for surface depolarisation to reach all parts of the mechanical apparatus contained within the myofibrils.
Myofibrils are compsed of adjacent blocks of actin and myosin, called sarcomeres, giving a characteristic ‘light’ and ‘dark’ pattern on polarised light microscopy (Figure 13.3). The light band is referred to as the ‘I’ band and contains only thin myofilaments containing actin, whereas the dark or ‘A’ bands contain both thick myofilaments made of myosin and the overlapping ends of some actin filaments. A and I refer to the anisotropic and isotropic appearance of the bands on polarised light microscopy. Each block of actin and myosin is separated by a Z line, and an H line is seen running through the middle of the I band. The side-by-side arrangement of actin and myosin is maintained by titin, a large protein.
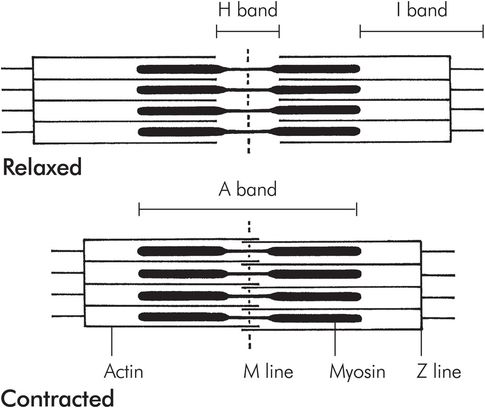
Structure of a sarcomere
There are two T tubules for each sarcomere, positioned at each end of the myosin filaments (Figure 13.4).
Thin myofilament
The thin myofilament comprises a complex of two molecules of actin and a molecule of tropomyosin bound up in a helical arrangement (Figure 13.5). At each half-turn of the helix, another protein complex called troponin is bound to the actin/tropomyosin structure. Troponin is made up from three complexes, troponin I, troponin T and troponin C.
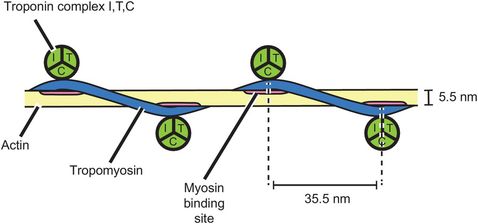
Thin myofilament
Thick myofilament
The thick myofilament is made of myosin molecules. Each myosin molecule consists of a long ‘tail’ and two shorter ‘head’ regions (Figure 13.6). Each head region contains an ATP and an actin binding site. The myosin molecules are packed together in a helical bundle, with the heads sticking outwards, between the thin myofilaments. The ‘headless’ region between adjacent myosin chains corresponds to the H line seen on light microscopy.
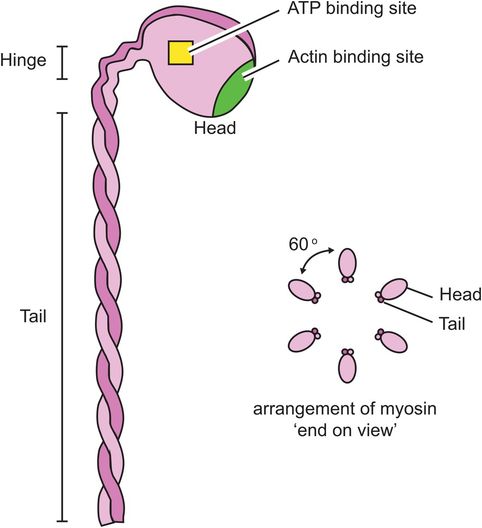
Thick myofilament
Actin and myosin bind strongly to each other, forming links between the head of the myosin molecules and active sites on the actin molecules. In the presence of tropomyosin and troponin, this binding does not occur. It is thought that the inhibitory action of tropomyosin and troponin itself has to be inhibited to allow muscle contraction to occur.
The sequential steps in muscle contraction
1. Action potential depolarises nerve ending attached to muscle cell, releasing small amounts of acetylcholine.
2. Acetylcholine acts as a neurotransmitter and on binding with acetylcholine receptors on the muscle cell membrane causes depolarisation of the sarcolemma, identically to a nerve membrane.
3. Depolarisation spreads rapidly across the sarcolemma, including the portions deep within the cell, via the T tubules.
4. Depolarisation of the cell causes release of calcium from the sarcoplasmic reticulum.
5. Calcium ions bind to troponin C, releasing the inhibition of tropomyosin on actin and allowing actin to bind to myosin.
6. Repeated binding of actin and myosin causes the molecules to slide alongside one another, causing muscular shortening.
7. The calcium is pumped back into the sarcoplasmic reticulum, troponin/tropomyosin inhibits actin and myosin binding once again, and contraction stops.
Muscle contraction
Muscle contraction occurs as a sequence of events beginning with depolarisation of the motor nerve cell endings and resulting in shortening of the myofibrils. The process linking nerve cell depolarisation to muscle cell contraction is known as excitation–contraction coupling.
Excitation–contraction coupling
Nerve endings attached to muscles form specialised neuromuscular junctions (NMJs). The end of the nerve is flattened into an unmyelinated terminal button and the muscle cell wall opposite is thickened and convoluted into junctional folds. Between the two cells there is a space, the junctional gap (Figure 13.7).
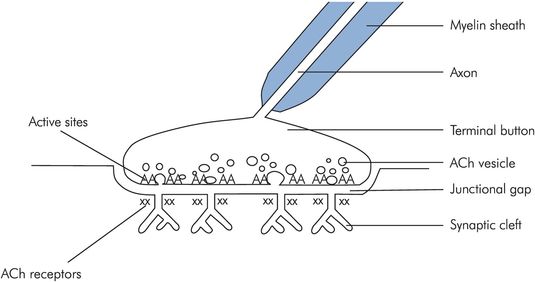
Structure of the NMJ
When the nerve ending is depolarised, vesicles containing acetylcholine (ACh) fuse with the cell membrane, releasing the ACh into the junctional gap. Approximately 60 vesicles fuse, each containing around 4000 molecules of ACh. The ACh diffuses across the gap, and binds to nicotinic acetylcholine receptors on the muscle wall. Each muscle end plate contains around 50 million acetylcholine receptors, but activation of as few as 25,000 is sufficient to cause muscle depolarisation. With approximately 240,000 molecules of ACh being released with each nerve ending depolarisation, there is a 10:1 excess of neurotransmitter above the necessary threshold, ensuring reliable neurotransmission.
The nicotinic acetylcholine receptor is made of five protein subunits and spans the lipid bilayer of the muscle cell. Binding of acetylcholine on the α subunit causes a conformational change and opens a pore in the receptor, allowing the rapid passage of sodium down its concentration gradient into the cell and causing muscle cell membrane depolarisation (Figure 13.8).
Myofibril depolarisation is analogous to nerve cell depolarisation, but with some differences. The resting membrane potential of muscle is around –90 mV and the action potential lasts 2–4 ms. Conduction of the action potential travels at around 5 m s–1. Initiation of myofibril contraction involves the interaction of three components of the sarcotubular system, the T-tubule, the sarcoplasmic reticulum and the sarcolemma. These form the ‘sarcotubular triad’ (Figure 13.9).
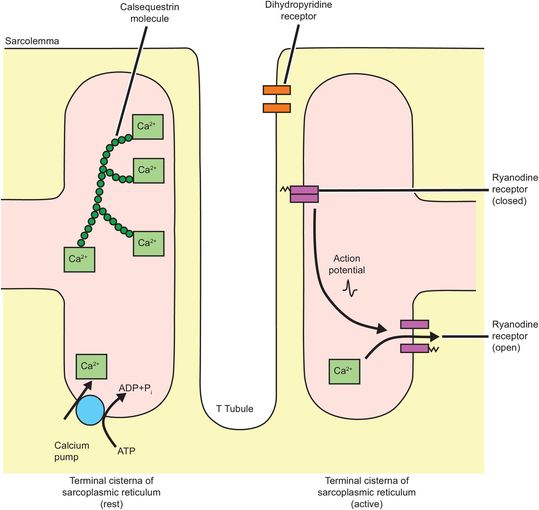
Sarcotubular triad
Depolarisation of the sarcolemma is sensed by dihydropyridine receptors in the T tubules. Dihydropyridine receptors are voltage-sensitive calcium channels and are attached to ryanodine receptors. Ryanodine receptors span both the sarcoplasmic reticulum membrane and connect to sarcolemma. The signal received from the dihydropyridine receptors induces a conformational change in the ryanodine receptor.
These receptors function as calcium channels when activated, and calcium moves down its concentration gradient out of the sarcoplasmic reticulum and into the sarcoplasm where the calcium concentration increases 100- to 1000-fold (10–8 to 10–5 mol L–1).
Ryanodine receptor mutations are important in anaesthesia because they are responsible for malignant hyperthermia and central core disease, and dantrolene is an inhibitor of the ryanodine receptor.
The released calcium binds to troponin C, causing it to change the physical shape of the other troponin (I and T) molecules. The whole troponin complex then displaces tropomyosin on the actin molecule, exposing myosin binding sites. Myosin binding to actin causes a further displacement of the tropomyosin molecule, exposing more myosin binding sites on the actin molecule and encouraging further cross-bridges to be made.
Myosin binding to actin triggers a conformational change in the myosin molecule, causing the head to move backwards, and as a result the two molecules start to move. This is the so-called ‘power stroke’ (Figure 13.10). Once the myosin head has changed position, it is released by the actin, is reset and is free to bind to the actin once again. This process, repeated thousands of times, causes the molecules to ‘walk’ past each other, resulting in macroscopic muscle contraction. This process continues in each sarcomere until the mysosin molecules reach the Z plate. Each sarcomere may shorten from around 3.5 to 1.5 μm in length.
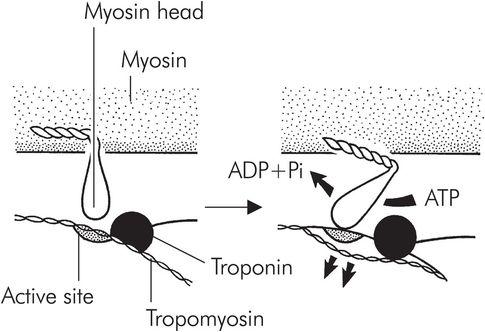
The power stroke
Evidence for these conformational changes comes from electron microscopy and x-ray interference studies.
Approximately 50 ms after a single depolarisation, the calcium ions are pumped back into the sarcoplasmic reticulum, actinomyosin slides back over the actin active sites and relaxation occurs (Figure 13.11). The calcium pump in the sarcoplasmic reticulum is continually active throughout this muscle contraction, and calcium concentration in the sarcoplasm starts to fall before maximum power is generated by the sarcomere. Although muscle cells exhibit a refractory period (like nerves), repeated depolarisation allows the concentration of calcium in the sarcoplasm to increase faster than the calcium pump can return it to the sarcoplamic reticulum, and maximum power may then be generated.
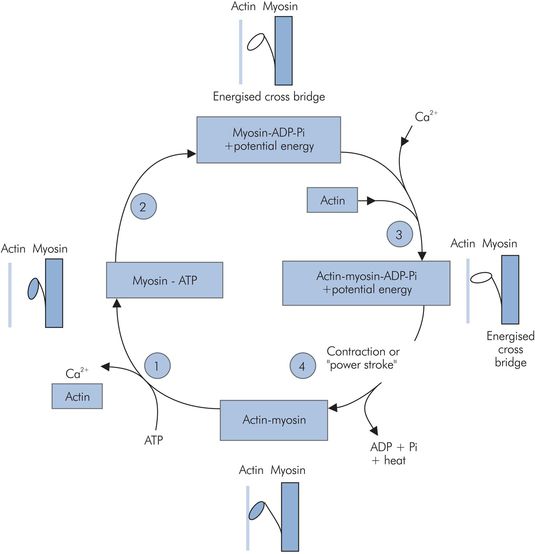
Actin–myosin contraction cycle:
1. ATP binds to myosin, releasing the actin and Ca2+.
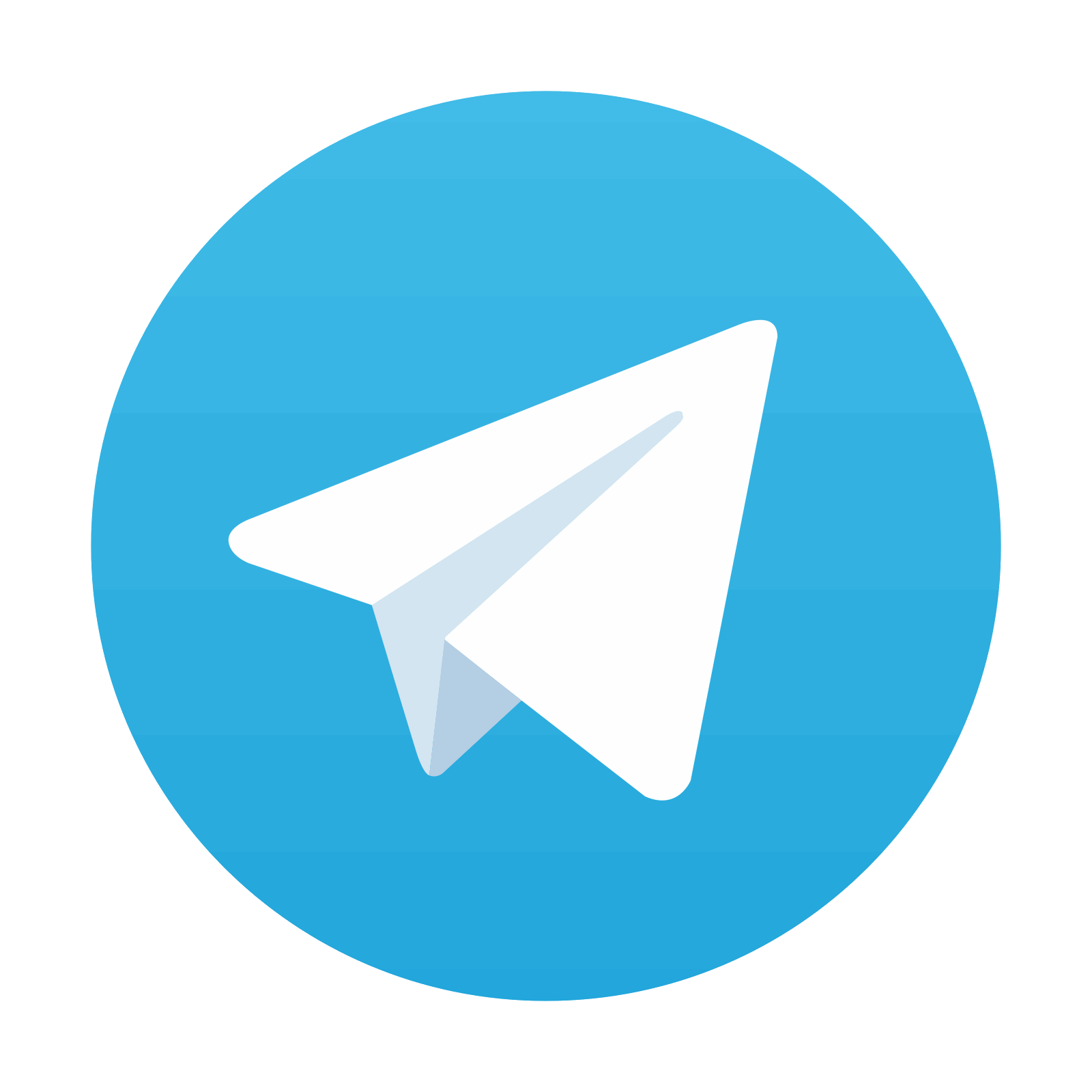
Stay updated, free articles. Join our Telegram channel

Full access? Get Clinical Tree
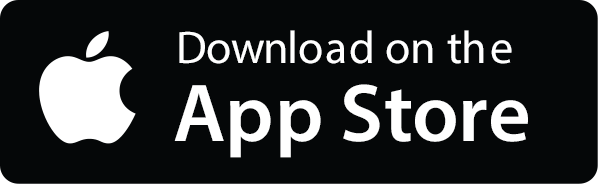
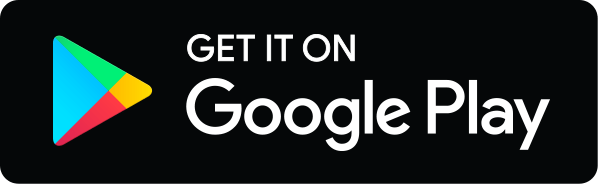
