PATHOPHYSIOLOGY
MODS usually occurs in patients who exhibit signs of a generalized inflammatory response (systemic inflammatory response syndrome [SIRS]; Table 52.2) (1). Although SIRS is often the result of infection (triggered by microbial products), other conditions such as necrotizing pancreatitis or trauma can release patient-derived factors called danger-associated molecular patterns (DAMPs) that lead to systemic manifestations of inflammation (18). Two or more SIRS criteria due to infection have been defined as sepsis. However, the need for two SIRS criteria to define sepsis will exclude one out of eight patients with sepsis and organ failure (19). Updated guidelines have broadened the diagnostic criteria for sepsis that were originally proposed in 1992 (20). Analysis of these two definitions shows high sensitivity for diagnosing sepsis but with a low specificity (21). For those patients who present with SIRS only, a significant number will progress to sepsis, septic shock, and, ultimately, MODS (22). Although suspected or documented infection is not required for the development of MODS, the syndromes of SIRS, sepsis, and MODS are closely related. Consequently, the review of the pathophysiology of MODS will also include discussions of SIRS and sepsis.
Derangements in Oxygen Delivery and Consumption
In most tissues, oxygen consumption (V̇O2) is determined by metabolic demand and is independent of ḊO2. When ḊO2 is reduced, V̇O2 is maintained by increased oxygen extraction by the tissues. If ḊO2 is reduced to the point where the metabolic need cannot be met, V̇O2 becomes “supply dependent” (Fig. 52.2). The point at which V̇O2 decreases is called the critical ḊO2. Although one study (23) suggested that the critical ḊO2 in anesthetized humans is 330 mL/min/m2, another investigation in which life support was withdrawn in critically ill patients demonstrated that the value is substantially lower (24).

FIGURE 52.1 Relationship between the number of failed organs on admission and intensive care unit mortality. (From Vincent J-L, Sakr Y, Sprung CL, et al. Sepsis in European intensive care units: results of the SOAP study. Crit Care Med. 2006;34: 344–353, with permission.)
TABLE 52.2 American College of Chest Physicians/Society of Critical Care Medicine Definitions of Sepsis and Organ Failure |
![]() |
A number of studies from the 1970s, 1980s, and early 1990s seemed to suggest that systemic V̇O2 was supply dependent over a wide range of ḊO2 in patients with sepsis or ARDS, a condition termed “pathologic supply dependency.” The validity of the concept of pathologic supply dependency was subsequently challenged as clinical investigations that utilized independent means to measure both ḊO2 and V̇O2 failed to demonstrate pathologic supply dependency in patients with ARDS or sepsis.
More recently, there has been a recognition that nonglobal supply dependency may occur in patients. There is an increasing appreciation that the regional circulation and microcirculation—arterioles, capillary bed, and postcapillary venules—play a crucial role in the pathogenesis of organ dysfunction in shock. Heterogeneous microcirculatory abnormalities occur due to changes in the activation state and shape of endothelial cells, alterations in vascular smooth muscle tone, activation of the clotting system, and changes in red and white blood cell deformability. Alterations in microvascular circulation have been demonstrated in congestive heart failure, cardiogenic shock, hemorrhage, and sepsis; in congestive heart failure these include reduced conjunctival microvascular density and attenuated nailfold capillary recruitment during postocclusive reactive hyperemia (25). Animal models of hemorrhagic shock demonstrate attenuated functional capillary perfusion—a measure of the number of capillaries that are actively moving blood—of skeletal muscle and the intestinal villi (26,27). Renal and intestinal regional blood flow is reduced despite resuscitation and return of global hemodynamics back to baseline values (28). Tissue (skeletal muscle) oxygen saturation in septic patients is no different than that observed in healthy controls or postsurgical patients (29); however, microvascular compliance and skeletal muscle oxygen consumption is reduced, and postischemic reperfusion time is increased compared to controls. Sidestream dark field (SDF) imaging is a technique by which perfusion of small and large vessels in the microcirculation of mucosal surfaces can be seen and quantified. Clinical studies (30,31) where SDF imaging has been utilized have shown that the fraction of perfused small vessels in patients with severe heart failure, cardiogenic shock, or sepsis is significantly lower than in those critically ill patients without those conditions (Fig. 52.3). Furthermore, alterations in microvascular perfusion are a strong predictor of poor ICU outcome in patients with severe sepsis (32). Transfusion of packed red blood cells may improve microcirculatory perfusion in patients who are identified as having altered microcirculatory perfusion by SDF imaging. Nonetheless, efforts to improve microcirculatory flow with a variety of agents—including nitroglycerin, dobutamine (33), inhaled nitric oxide (NO) (34), enoxi-mone, dopamine, and corticosteroids—have had no effect on organ dysfunction (35).

FIGURE 52.2 The relationship between oxygen delivery and consumption. The solid line represents the normal relationship. The dashed line shows pathologic supply dependency. (From Heard SO, Fink MP. Multiple-organ dysfunction syndrome. In: Murray MJ, Coursin DB, Pearl RG, et al., eds. Critical Care Medicine. Perioperative Management. Philadelphia, PA: Lippincott Williams & Wilkins; 2002.)
Functional cellular hypoxia—“cytopathic hypoxia”—or metabolic failure is a condition where the cell is incapable of utilizing oxygen to produce ATP despite adequate oxygen delivery (36–38). The defect in oxygen utilization likely resides in the mitochondrion. Rapid autopsies of patients dying from sepsis show normal gross histology but significant mitochondrial injury on electron microscopic examination (39). Muscle biopsies from septic patients reveal that skeletal muscle ATP concentrations and respiratory chain activity are lower compared to samples obtained from control patients (40,41). The mechanism by which cytopathic hypoxia or metabolic failure occurs has not been fully elucidated. However, NO and its metabolite, peroxynitrite, are mediators released during sepsis and are inhibitors of the mitochondrial electron transport chain (42). Single-strand breaks in nuclear DNA can occur in sepsis by a variety of endogenously formed oxidants, including peroxynitrite. Poly(ADP-ribose) polymerase (PARP) is a highly energy dependent enzyme that is activated by the formation of these DNA breaks and may cause cellular energy depletion (43,44). Porphyrin-based agents which increase the degradation of peroxynitrite improve cardiovascular function and decrease the incidence of MODS in animal models of septic shock (45). If the reduction in ATP production is sufficient, cell death should occur; however, this does not appear to the overriding cause of MODS. More likely, a new steady state is reached where there is enough ATP production for cell survival but not for all metabolic functions (18).

FIGURE 52.3 Sublingual microcirculation as assessed by orthogonal polarization spectral imaging in a healthy volunteer (A) and in a patient with early septic shock (B). Normal capillary density is observed in panel A whereas low capillary density is observed in panel B. Real-time images may be viewed at http://www.cooperhealthorg/content/gme_fellowship_shock.htm. (From De Backer D, Creteur J, Preiser J-C, et al. Microvascular blood flow is altered in patients with sepsis. Am J Respir Crit Care Med. 2002;166:98–104, with permission.)
TABLE 52.3 Inflammatory Mediators Important in the Pathogenesis of Sepsis and the Multiple Organ Dysfunction Syndrome |
![]() |
ROLE OF INFLAMMATORY AND VASOACTIVE MEDIATORS
Although early clinical series emphasized the implication of uncontrolled infection in the development of MODS, it is clear that MODS can occur with either extensive tissue injury such as that seen with trauma, pancreatitis, or sepsis. A large amount of evidence is available that implicates the release of inflammatory mediators in the pathogenesis of MODS (Table 52.3).
Complement, Neutrophils, and Reactive Oxygen Metabolites
The complement cascade is activated via three pathways (Fig. 52.4). The classical pathway is triggered by antibody-coated targets or antigen–antibody complexes. The alternative pathway is activated by aggregated immunoglobulins, products of tissue trauma, lipopolysaccharide (LPS), and other complex polysaccharides. The lectin-ficolin pathway is initiated by the binding of organisms to mannose–binding lectin (MBL), a protein important in innate immunity (46). Once MBL is bound to a pathogen, an MBL-associated serine protease is produced, which forms a C3 convertase by cleavage of C4 and C2. Products of the complement pathway activate neutrophils, which can obstruct capillaries and release oxygen radicals and lysosomal enzymes—among other mediators, thereby damaging the endothelium. Furthermore, adhesion molecules, which are expressed on both polymorphonuclear leukocytes (PMNs) and vascular endothelium in response to LPS and other inflammatory mediators, facilitate the adherence and diapedesis of PMNs through the endothelium.

FIGURE 52.4 The three complement activation pathways (classic, alternative, and lectin). IgG, immunoglobulin G; IgM, immunoglobulin M; IgA, immunoglobulin A; CRP, C-reactive protein; MBL, mannose-binding lectin protein; MASP, MBL-associated proteases; C1-INH, C1-inhibitor; LPS, lipopolysaccharide; CAM, cell adhesion molecules; MAC, membrane attack complex; PMN, polymorphonuclear leukocytes; MCP, monocyte chemoattractant protein; CR1, complement component receptor 1. (From Goldfarb RD, Parrillo JE. Complement. Crit Care Med. 2005;33:S482–S484, with permission.)
A significant amount of animal evidence exists suggesting that complement activation is important in the pathophysiology of MODS (47–50). Clinically, activation of both complement and neutrophils occurs in patients with ARDS or burns (51,52), and circulating plasma levels of C3a correlate with severity of injury and outcome in patients with multiple trauma (53). Evidence of complement and neutrophil activation has also been found in bronchoalveolar lavage (BAL) from patients with ARDS (54). Administration of a C1 inhibitor in patients with severe sepsis and septic shock reduces neutrophil activation (55) and improves renal function and SOFA scores compared to untreated control patients (56).
Reactive oxygen species—superoxide anion, hydrogen peroxide, and the hydroxyl radical—are produced in the mitochondria and released by activated PMNs. They can injure tissues by damaging DNA, cross-linking cellular proteins, and causing peroxidation of membrane lipids (57,58), diminished membrane fluidity and increased membrane permeability, resulting in cellular dysfunction. The conclusion that toxic oxygen radicals are important in the pathophysiology of respiratory dysfunction comes from clinical studies of patients with ARDS where plasma levels of lipid peroxides are elevated, levels of hydrogen peroxide are increased in the expiratory condensate (59), and oxidative damage to proteins in BAL fluid is found (60); ARDS patients have reduced levels of oxygen radical scavengers (e.g., α-tocopherol, ubiquinone, and glutathione), a sign of “oxidant stress” (61,62). Antioxidant therapies, however, have not translated into improved outcome for patients with ARDS or MODS. The OMEGA trial which examined dietary supplementation with omega-3 fatty acids, gamma-linolenic acid, and a number of antioxidants on the outcome of patients with ARDS showed the antioxidant group had fewer ventilator-free days, ICU free days and nonpulmonary organ failure free days (63). Other studies suggest such interventions may improve days “free” of acute lung injury (ALI) (64), mechanical ventilation (65), and incidence of new organ failures (65); decrease SOFA scores (66); and reduce oxidative stress during septic shock (67).
The Kallikrein–Kinin System
The kallikrein–kinin system is part of the contact system, and is composed of complement, coagulation, and kallikrein–kinins, with bradykinin, the end product of this cascade, resulting in vasodilation and increased vascular permeability. Some of these effects are mediated by the release of secondary mediators, such as NO and eicosanoids. A bradykinin receptor antagonist (CP-0127) for the adjuvant therapy of sepsis failed to alter the 28-day mortality (68).
The Coagulation System
LPS and many proinflammatory mediators will activate the coagulation system (Fig. 52.5), primarily by the extrinsic tissue factor–dependent pathway, as these mediators induce the expression of tissue factor (TF) on monocytes and endothelial cells (69). Although these same mediators activate the fibrinolytic system, subsequent increases in plasminogen activator inhibitor-1 (PAI-1) and thrombin-activatable fibrinolysis inhibitor (TAFI) effectively suppress fibrinolysis. Important coagulation inhibitors—antithrombin, tissue factor pathway inhibitor (TFPI), protein C, protein S, and the endothelial-bound modulators heparan sulfate and thrombomodulin—may be downregulated (69), resulting in a net procoagulant tendency with potential for the development of disseminated intravascular coagulation (DIC). DIC can cause microvascular thrombosis and organ failure, and/or bleeding from consumption of platelets and clotting factors (70,71). Nonetheless, three clinical trials of anticoagulant therapy failed to showed benefit in patients with severe sepsis (72–74).

FIGURE 52.5 Revised coagulation scheme. VIIa complex is capable of mediating the conversion of factor IX to IXa directly, thereby establishing a link between the two pathways. Thus, TF appears to have a dual role with respect to coagulation: (i) TF initiates coagulation through activation of factor X, and (ii) through its action on factor IX, TF supports amplification. An additional mechanism that supports the importance of the intrinsic cascade is activation of factor VIII through feedback mechanisms involving thrombin. (From Aird WC. Coagulation. Crit Care Med. 2005;33:S485–S487, with permission.)

FIGURE 52.6 The eicosanoid pathway. PLA2, phospholipase A2; ROI, reactive oxygen intermediates; COX, cyclo-oxygenase; LO, lipo-oxygenase; HETE, hydroxyeicosatetraenoic acid; LT, leukotriene; TX, thromboxane; LX, lipoxin; PG, prostaglandin. (From Cook JA. Eicosanoids. Crit Care Med. 2005;33: S488–S491, with permission.)
Prostaglandins, Leukotrienes, and Platelet-Activating Factor
Prostaglandins (PGs), leukotrienes (LTs), and platelet-activating factor (PAF) are potent lipid mediators formed by the stimulation of a membrane-bound enzyme, phospholipase A2 (PLA2), via a variety of mediators including norepinephrine, adenosine, bradykinin, PAF, tumor necrosis factor (TNF), and interleukin (IL)-1β (75). PLA2 catalyzes membrane phospholipids to lyso-PAF and arachidonic acid (Fig. 52.6). Both experimental and clinical studies support the notion that these mediators play a role in the pathophysiology of sepsis and MODS.
Elevated plasma levels of thromboxane B2 (TXB2), the metabolite of the prostaglandin thromboxane A2 (TXA2), are observed in animal models of sepsis and are correlated with organ injury and outcome. However, clinical trials of nonselective inhibitors of cyclo-oxygenase (COX) (ibuprofen) showed no effect on survival in sepsis (76) and a trial evaluating the effectiveness of a selective inhibitor of a group IIA secretory phospholipase in patients with suspected sepsis and organ failure showed no effect on organ function (77). Ketoconazole, an imidazole derivative that inhibits thromboxane synthase, prevents the development of ARDS in patients at risk (78,79); established ARDS is not reversed nor is survival improved (80).
Significant data exists supporting the role of PAF in the pathogenesis of sepsis and MODS. In vitro, incubation of macrophages with PAF will lead to an exaggerated release of TNF and TF by these cells after LPS exposure; LPS-induced release of TNF by macrophages is inhibited by PAF receptor antagonists. PAF expression on the surface of endothelial cells will result in PMN adherence and activation. In addition, stimulation of PAF receptors on the endothelium results in changes in cell shape and cytoskeletal structure. Clinically, depressed plasma levels of PAF acetylhydrolase (PAF-AH), the enzyme responsible for metabolizing PAF, have been observed in critically ill patients and correlate inversely with organ dysfunction. However, a phase III trial of recombinant PAF-AH failed to improve outcome or prevent organ dysfunction (81). A subsequent study of critically ill patients revealed that plasma levels of PAF-AH were variable over time and with severity of illness (82,83), providing a partial explanation for the lack of efficacy of recombinant PAF-AH.
Cytokines
Cytokines are small proteins secreted by nearly all nucleated cells and exhibiting autocrine, paracrine, or endocrine activity (84,85); they are generally classified as proinflammatory or anti-inflammatory. This classification, however, is somewhat arbitrary as an individual cytokine may act in either fashion, depending on the underlying biologic process. Proinflammatory cytokines such as TNF and IL-1 can stimulate the release of other mediators: PAF, NO, LTs, and PGs.
TNF assumes an important role in the pathogenesis of human sepsis, septic shock, and MODS; it is directly cytotoxic to some cell types, will induce the expression of adhesion molecules on neutrophils and endothelial cells to promote the recruitment of these cells to the site of injury or infection and will increase endothelial permeability. Metabolic effects attributable to TNF include activation of the acute-phase response, fever (along with IL-1), skeletal muscle catabolism, and increased peripheral lipolysis and hepatic lipogenesis (85). When injected into normal volunteers, small doses of LPS or recombinant TNF will reproduce many of the metabolic and hemodynamic changes observed in sepsis (83,86). Similar findings are observed in animal studies, and treatment with anti-TNF antibodies will prevent many of the adverse consequences of endotoxic or live gram-negative bacterial shock (87). However, in studies of critically ill patients, the correlation of plasma TNF levels and outcome is variable (88,89), possibly related to timing and method of the TNF assay, acuity, etiology, or treatment of the patient’s illness, or patient genetic differences. The modulation of TNF as a therapy for sepsis and MODS remains murky as multiple studies failed to show significant benefit of either anti-TNF antibodies or soluble TNF receptors in severe sepsis (90). In one trial where patients were stratified according to initial plasma levels of IL-6 (as a marker of severity of illness), outcome was improved, and organ dysfunction was ameliorated with the administration of a monoclonal antibody to TNF (91).
Like TNF, IL-1 has a wide variety of biologic actions and has been implicated in the pathogenesis of sepsis and MODS (92); these molecules often act synergistically. IL-1 induces the expression of COX-2 and inducible nitric oxide synthase (iNOS) expression (93). Furthermore, IL-1 increases the expression of other cytokines—most notably TNF and IL-6—chemokines, adhesion molecules, and a number of tissue proteases and matrix metalloproteases (93). IL-1 also stimulates the release of myeloid progenitor cells, resulting in neutrophilia (93). However, use of IL-1 receptor antagonists in patients with sepsis does not reduce mortality nor reverse organ failure (94–96).
Interleukin 6 (IL-6) is another cytokine that has been identified to be important in the response to infection and development of MODS. Small doses of endotoxin administered to normal volunteers will stimulate the release of IL-6 (97). IL-6 will persist for longer periods of time in the blood than other cytokines and may serve as an important marker for the outcome of patients with sepsis or septic shock. Both the IL-6 receptor and the signaling receptor gp130 are required for the biologic activity of IL-6 to be realized (97). Murine models of hemorrhagic shock indicate that IL-6 is important in the development of gut barrier dysfunction (97). In addition, IL-6 may be important in promoting thrombosis during sepsis. Passive immunization with an anti–IL-6 antibody reduces activation of the coagulation cascade in a primate model of endotoxicosis but has no effect on the coagulation abnormalities associated with low-dose LPS in humans (98).
IL-8 is a chemotactic cytokine (chemokine) expressed principally by monocytes and macrophages by stimulation with LPS, bacteria, TNF, and IL-1 (99). IL-8 induces chemotaxis of inflammatory cells, and its presence at sites of inflammation may persist for long periods of time (99). That IL-8 is important in the development of MODS is made by the observation of high IL-8 levels in BAL fluid from patients with ARDS or pneumonia (100). Although neutralizing IL-8 may reduce cardiac ischemia/reperfusion injury in dogs, a reduction in chemokines increases mortality in animal models of pneumonia (99).
High-mobility group box 1 (HMGB1) is a cytokine that was been identified as an inflammatory mediator (Fig. 52.7) (101). HMGB1 is released in a delayed manner from a variety of cells in response to LPS or bacteria (102). Exposure of the lung to HMGB1 increases neutrophil accumulation, edema, and other proinflammatory cytokines, whereas gastrointestinal exposure results in increased gut permeability and translocation of bacteria to mesenteric lymph nodes (102). Administration of this cytokine to animals causes death as a result of epithelial barrier disruption. Treatment of experimental endotoxicosis or sepsis with antibodies to HMGB1 or HMGB1 antagonists improves survival (101–103). There have been no clinical studies to evaluate the efficacy of anti-HMGB1 therapies in sepsis or other inflammatory conditions.
Macrophage migration inhibitory factor (MIF) is a cytokine that is found in macrophages in preformed cytoplasmic pools (104). It is released rapidly in response to bacterial products and works to upregulate and sustain the activation of a variety of cell types to produce TNF, IL-1, IL-6, and IL-8 (104). The major action of MIF may be the regulation of p53-dependent apoptosis. Anti-MIF therapy in animal models of sepsis is protective (104). Although MIF appears to be an important mediator of sepsis and sepsis-induced MODS (105), it does not appear to play a prominent role in tissue injury resulting from trauma (106).
Nitric Oxide
NO is an inorganic free-radical gas, produced by catalysis of one of the terminal guanidine nitrogens of L-arginine by the NOS group of enzymes (107). Two general classes of NOS have been described: Constitutive (calcium-dependent) NOS (neuronal and endothelial) and inducible (calcium-independent) NOS (108). The production of the latter enzyme is induced by LPS, TNF, and a variety of other inflammatory mediators. A variety of cells and tissues release NO, including endothelium, vascular smooth muscle, neutrophils, and mononuclear, glial, mast, hepatic, and adrenal medullary cells (108). Vasorelaxation, neurotransmission, and microbicidal activity are some of the important functions that NO possesses. The role that NO plays in the host is a function of the rate and timing of its production and the surrounding environment. Normally, NO acts as a direct signaling molecule (e.g., vasorelaxation and neurotransmission), and low levels of NO (produced by constitutive NOS and at times by inducible NOS) have protective effects (Fig. 52.8) (108). Alternatively, it may function as an indirect cytotoxic agent and induce intestinal barrier dysfunction.

FIGURE 52.7 Inflammatory responses in various areas of the host that are medicated by high-mobility group box 1 (HMGB1). tPA, tissue plasminogen activator; PAI, plasminogen activator inhibitor. (From Wang H, Yang H, Tracey KJ. Extracellular role of HMGB1 in inflammation and sepsis. J Intern Med. 2004;255: 320–331, with permission.)

FIGURE 52.8 The divergent effects of nitric oxide (NO) derived from the endothelium (eNOS, endothelial nitric oxide synthase) or as a consequence of inflammation (iNOS, inducible or inflammatory NOS.) (From Levy RM, Prince JM, Billiar TR. Nitric oxide: a clinical primer. Crit Care Med. 2005;33:S492–495, with permission.)
Significant experimental and clinical evidence suggests that NO plays an important role in the pathophysiology of sepsis and MODS. iNOS and NO production increase in animals during both endotoxic and hemorrhagic shock. iNOS-deficient mice are protected from LPS-induced hypotension, and have a higher survival following endotoxicosis (109). NO contributes to TNF-induced cardiac dysfunction in a concentration-dependent fashion (110). Increased urinary excretion of NO metabolites (nitrite and nitrate) has been reported in septic patients and correlates inversely with systemic vascular resistance (SVR) (111).
Excess NO in the presence of superoxide anion results in the formation of peroxynitrite (ONOO (–)), a reactive oxidant that causes lipid peroxidation, inhibits mitochondrial respiration, inactivates glyceraldehyde-3-phosphate dehydrogenase, inhibits membrane sodium/potassium ATP activity, and triggers DNA single-strand breakage. As mentioned previously, DNA damage activates the nuclear enzyme, PARP, which can lead to cellular energy depletion and death (109). Furthermore, excessive amounts of NO and peroxynitrite can activate the transcription factor, NFκB, and amplify the inflammatory response (112).
The efficacy of inhibitors of NOS in the treatment of sepsis and MODS is unclear, and the use of some inhibitors may actually be detrimental. Although hypotension, vascular leak, and vasopressor requirements can be reduced with the use of nonspecific NOS inhibitors, microcirculatory blood flow can be altered, resulting in organ injury. Indeed, a large randomized, prospective trial evaluating the efficacy of the nonspecific NOS inhibitor, L-N (G)-monomethylarginine, in patients with septic shock was stopped early because the death rate was higher in the intervention group (113).
THE ENDOTHELIUM
The role of the vascular endothelium is to control the flow of nutrients, blood cells, and a broad array of biologically active molecules to the tissues; this is achieved via membrane-bound receptors for a plethora of molecules and through tight junction proteins and receptors that regulate cell–cell and cell–matrix relationships (114). Endothelial cell injury may well impair the delivery of nutrients to tissues and allow the extravasation of proinflammatory mediators into the interstitial space.
Significant data supports the role of an impaired endothelium in the development of MODS. Endothelial cell exposure to LPS will cause anatomic changes including nuclear vacuolization, cytoplasmic swelling and fragmentation, and detachment from the internal elastic lamina (115). In humans with septic shock, elevated levels of circulating endothelial cells can be detected and correlate with outcome. High plasma levels of molecules that are expressed on the surface of endothelial cells (i.e., thrombomodulin [TM], intercellular adhesion molecule [ICAM]-1, and E-selectin) are observed during sepsis and ALI, and are an indirect indication of endothelial damage (116) and predict organ failure and death (117). This injury appears to be sustained, as injection of small doses of LPS into human volunteers will result in high plasma levels of TM that peak at 24 hours and of TF that are still increasing at 48 hours (114).
The uninjured endothelial cell has important anticoagulant properties. Several heparin-like molecules are expressed on the surface of the cells to accelerate the inactivation of serine proteases of coagulation by antithrombin (114). Thrombomodulin binds thrombin and forms a complex that activates protein C; exposure of the endothelial cells to inflammatory or septic mediators will shift the endothelial cells to a procoagulant state by increasing expression of TF and internalization of TM, and the endothelial cell will have impaired release of tissue plasminogen activator and an increased release of PAI-1. This procoagulant/antifibrinolytic state is associated with fibrin deposition, platelet consumption, microthrombi, tissue ischemia and necrosis, and an increased risk of death (118).
Before leukocytes and monocytes can migrate into tissues, they must adhere to the endothelium, accomplished by the local synthesis of PAF, IL-1, IL-8, and TNF, which stimulates the expression of surface molecules called selectins on leukocytes (L-selectin) and endothelial cells (E-selectin). The interplay between these selectins allows loose binding of the leukocyte to the endothelium. Leukocytes are bound more strongly to the endothelium by the interaction between the CD11/CD18 complex, which is expressed on the leukocyte, and the ICAM-1, which is expressed on the endothelial cell membrane. In animal models of sepsis, endotoxicosis, or ischemia/reperfusion, monoclonal antibodies to the CD11/CD18 integrin or to L-selectin will improve organ dysfunction (119). Knockout animals lacking either ICAM-1 or E-selectin have improved survival during experimental sepsis (120). Clinically, plasma ICAM-1 levels are higher in patients with septic shock than in healthy controls or patients with SIRS (116), and the levels correlate with the severity of shock.
Endothelium-derived relaxation is also impaired in sepsis, and such alteration may contribute to MODS. In normal volunteers, small doses of LPS will also impair endothelium-dependent relaxation for days (121). These data help explain the observations that reactive forearm hyperemia is attenuated in patients with sepsis. In addition, the importance of intact ecNOS in these patients is supported by the observation that treatment of patients with septic shock by nonselective NOS inhibitors is associated with no change or an increase in mortality compared to untreated patients (113).
EPITHELIAL BARRIER DYSFUNCTION
Epithelial cells help maintain normal organ function by the maintenance of distinct compartments. The tight junctions between adjacent epithelial cells serve several important functions: (a) differentiation (122) of the cell into apical and basolateral domains, (b) preservation of cellular polarity, (c) generation of distinct internal environments formed by the epithelial layer, and (d) providing a semipermeable barrier that regulates the passive diffusion of solutes between the paracellular pathway and prevents entry of microbes and toxins (e.g., intestine and lung) (122).
Data from studies using cultured epithelial monolayers show that NO and peroxynitrite, as well as other proinflammatory mediators, increase the permeability of the monolayer. The mechanism for this increase in permeability is incompletely understood; however, some data suggest that there is a decrease in the expression and improper localization of some of the tight junction proteins. Other investigations have determined that inhibition of the epithelial cell membrane Na (+)-K (+)-ATPase pump by these mediators results in cellular swelling and an increase in intracellular sodium concentration, which ultimately impairs the expression and localization of the tight junction proteins. Data from murine models of endotoxemia indicate that LPS will decrease the expression and alter the localization of tight junction proteins in the intestine compared to control animals (123). Furthermore, these animals will have increased number of bacteria recovered from regional mesenteric lymph nodes. NO appears to be important in the disruption of gut barrier function, as neither intestinal permeability nor bacterial translocation is observed when knockout mice lacking iNOS are treated with LPS. Similar alterations in hepatic (124) and pulmonary (125) epithelial barrier function and tight junction protein expression and localization have been shown during murine endotoxicosis.
Derangements in Gut Barrier Function
Although epithelial barrier function in the intestine may be altered during sepsis and other inflammatory states, other components of the gut barrier will prevent the bacteria or bacterial products from gaining access to systemic organs (126). In addition to alterations in the epithelial barrier described previously, other clinical conditions that can contribute to altered gut barrier function include antibiotics, stress ulcer prevention, hypoalbuminemia, vasoactive agents, and use of hyperosmolar feeding preparations. The clinical importance of the disrupted gut barrier function in the pathogenesis of MODS remains ill defined.
APOPTOSIS
Apoptosis (programmed cell death) is the term used to describe a specific method by which cells die; the event is a well-defined, active, and energy-dependent process. There are two primary pathways involved in apoptosis: The intrinsic (mitochondrial and endoplasmic reticulum) pathway and the extrinsic (“death receptor”) pathway (127). The latter pathway is activated by receptors such as Fas, with the subsequent activation of two enzymes, caspase-8 or -10. The intrinsic pathway stimulates caspase-9 by loss of the mitochondrial membrane potential and movement of cytochrome c into the cytosol. These initiator caspases cleave effector caspases (e.g., caspase-3 and -7), which result in the cleavage of cellular proteins and DNA and, ultimately, apoptosis (128).
The exact role of apoptosis in the development of MODS is unclear. Data from clinical studies show that upregulation of apoptotic pathways occurs in patients with ARDS (129). Furthermore, widespread apoptosis occurs in splenic and colonic lymphoid populations in patients who die from sepsis and MODS (130,131). The effect of apoptosis on immune function includes loss of various immune cells and impairment of immunity by apoptosis-induced immunosuppression of the remaining immune cells (132). Therapies directed against these programmed pathways are under intense investigation and include inhibition of cytochrome c release, use of RNA interference for gene silencing, and caspase inhibitors (132).
COMPLEX NONLINEAR SYSTEMS
The body may be considered a biologic network that is complex, highly coupled, and nonlinear (133). The host response to trauma, shock, or sepsis—involving metabolic, neural, endocrine, inflammatory, and immune components is such an example (134). The behavior of such a system cannot be predicted with great reliability; however, the system is “attracted” to specific states or stable configurations: “organized variability” (134,135). A large enough perturbation to an organ or mediator network may have unexpected and significant results elsewhere in the host and ultimately lead to MODS (136). In the healthy individual, there is a high degree of heart rate (beat-to-beat) variability; this may be lost and mortality increased in critically ill patients (137). In fact, normal volunteers injected with small doses of LPS exhibit loss of heart rate variability (138). Decreased heart and respiratory rate variability during spontaneous breathing trials predict extubation failure (139). Other examples of increased regularity of rhythms associated with disease include Cheyne–Stokes respiration, parkinsonian gait, neutrophil count in chronic myelogenous leukemia, and fever in Hodgkin disease (135). However, several diseases—acromegaly and Cushing disease—are associated with increased complexity (135). These data suggest that health is determined by “distance” from thermodynamic equilibrium: too much or too little variation (low or high entropy) represents pathologic conditions (135).
GENETIC SUSCEPTIBILITY
Single base variations in DNA—single nucleotide polymorphisms (SNPs)—are commonly used to discern genetic differences among patient populations (140). Approximately 1 in every 1,000 bases in the human genome is different between two unrelated individuals (140). By comparing healthy individuals to patients, SNPs involved in disease can be identified. Indeed, some—but not—all clinical studies have documented an increased risk of death and organ dysfunction in patients suffering from sepsis or ARDS and who are homozygotes for SNPs (141–145); this may have future clinical implications.
PREVENTION AND TREATMENT OF MULTIPLE ORGAN DYSFUNCTION SYNDROME
Prevention and treatment of MODS are nonspecific and include the goals of maintaining adequate tissue oxygenation, finding and treating infection, providing adequate nutrition support, minimizing iatrogenic complications, and when necessary, providing artificial support (e.g., dialysis or mechanical ventilation) for individual dysfunctional organs.
Resuscitation
An episode of circulatory shock is probably the most common event that occurs before the development of MODS. As a result, timely restoration of intravascular volume and oxygen delivery is important in preventing or abrogating MODS in high-risk patients (146).
In the ICU, the use of colloids as resuscitation fluid has been the subject of a number of important recent publications, which culminated in the Food and Drug Administration (FDA) issuing a black box warning on the use of hydroxyethyl starch (HES). The efficacy of volume substitution and insulin therapy in severe sepsis (VISEP) study (147), the Scandinavian starch for severe sepsis/septic shock (6S) trial (148), and the crystalloid versus hydroxyethyl starch (CHEST) trial (149) compared the use of starches with modified Ringer lactate, Ringer acetate, and sodium chloride 0.9%, respectively, for resuscitation. All trials failed to show a survival benefit with the use of starch, but demonstrated an increased risk of AKI. An updated systematic review and meta-analysis on the use of colloid versus crystalloids for fluid resuscitation in critically ill patients concluded that the use of colloids was not associated with an improvement in survival (150). Resuscitation with albumin compared to crystalloid in patients with severe sepsis does not alter 28- or 90-day mortality (151). However, there may be a benefit to the administration of albumin in septic shock as 90-day survival was higher and duration of vasopressor support was shorter in this subgroup (152).
The role of chloride in influencing the adverse effects of colloids or crystalloids on renal function has been recently questioned (153). A prospective, open-label, sequential period pilot study suggested that the use of balanced crystalloid or colloids solutions compared to chloride-rich solutions was associated with a significant decrease in the incidence of AKI and need for renal replacement therapy (154).
Assessing the adequacy of tissue oxygenation can often be difficult. The clinical parameters used most often, including arterial blood pressure, skin color, temperature, urine flow, mixed venous oxygen saturation, and blood lactate concentrations, may be unreliable. Although observational studies found that “supranormal” levels of ḊO2, V̇O2, and cardiac index were associated with higher survival rates, the majority of work over the past 15 years clearly show that resuscitation to these end points in critically ill patients is of no benefit, or actually worsens outcome. Likewise, the use of the pulmonary artery catheter to guide therapy has not been shown to be of benefit in a large number of studies (155–157) and pulmonary capillary wedge pressure does not accurately reflect ventricular volume, even in normal volunteers (158). Less invasive, and probably safer, monitors have been developed for monitoring cardiac output that compare favorably with the accuracy of the thermodilution method. Use of systolic blood pressure variation (SPV), pulse pressure variation (PPV), stroke volume variation (SVV), left ventricular end-diastolic area (as assessed by transesophageal echocardiography) and respiratory variability of the inferior vena cava diameter may be of greater benefit to guide volume resuscitation (159). Whatever monitor is used, resuscitation for severe sepsis or septic shock needs to happen early while avoiding fluid overload (16,17). Protocol-based algorithms do not appear to improve mortality (160–162); initial fluid therapy is 30 mL/kg of crystalloid (146). A higher transfusion threshold in established septic shock does not alter outcome (163). If vasopressor support is needed, norepinephrine is the vasopressor of choice and is titrated to achieve a MAP of 65 mmHg (164). Vasopressin (0.03 units/min) may be added to achieve the MAP goal or to reduce the dose of norepinephrine (146). Dobutamine may be added if signs of hypoperfusion persist and transthoracic or transesophageal echocardiography suggests cardiac dysfunction. Corticosteroids may be administrated if fluids and vasopressors have not restored hemodynamic stability (146). The abdominal compartment syndrome can be the cause of persistent hypotension and should be ruled out by measuring bladder pressure (165).
Mechanical Ventilation
The method by which patients are mechanically ventilated can contribute to organ dysfunction. A plethora of experimental and clinical data indicate that overdistension of the lung through the use of large tidal volumes will cause lung injury, stimulate the release of inflammatory mediators, and effect derangements in organs other than the lung (166–168). Use of small tidal volumes (6 mL/kg) in the care of patients with ALI and ARDS decreases mortality and increase ventilator-free and organ failure–free days (169). Nonetheless, an analysis of data from nine randomized ARDS trials demonstrated a direct correlation between driving pressure and survival (170). In addition, short-term use of cisatracurium (171) (48 hours) and the prone position (172) in patients with severe ARDS will improve outcome.
Cyclic “opening” and “closing” of collapsed airways during tidal ventilation is also thought to cause lung injury (173). A small study of patients with ARDS suggests that the use of positive end-expiratory pressure (PEEP) above the lower inflection point of the respiratory system compliance curve reduces mortality and the number of failed organs compared to control patients (174). Although this concept has been called into question by data demonstrating that efforts to improve recruitment of collapsed lung units with high levels of PEEP do not reduce mortality or duration of mechanical ventilation (175), the experimental protocol in this study was altered in mid-study, making questionable any conclusions drawn from this particular investigation. A systematic review and meta-analysis revealed that higher PEEP compared to lower PEEP in patients with moderate-to-severe ARDS was associated with improved survival (176). A computed tomography (CT) scan investigation of patients with ARDS showed that ventilator-induced hyperinflation rather than cyclic recruitment/derecruitment is associated with a greater release of pulmonary inflammatory mediators (177).
Fluid management is also an important component in the care of the patient with ARDS or ALI. Data show that a restrictive fluid strategy where cumulative fluid balance is kept close to zero in these patients improves the oxygenation index and increases the number of ventilator-free days without increasing the number of other organ failures (178).
Acute Renal Failure
Acute tubular necrosis (ATN) accounts for over 75% of the cases of acute renal failure (ARF) in the ICU (179), with a mortality rate ranging from 40% to 80%. The most common insult that predisposes ICU patients to ATN is persistent prerenal azotemia (179). Furthermore, in the critically ill patient, there is often more than one insult to the kidney: sepsis; exposure to aminoglycosides, amphotericin B, or radiocontrast agents; and the administration of nonsteroidal anti-inflammatory agents. Efforts to minimize these insults to the kidneys should be maximized. Timely resuscitation is important to prevent renal ischemia but targeting oliguria reversal as part of goal-directed management does not reduce renal dysfunction (180). If aminoglycosides must be used to treat infection, once-daily dosing (181) or the use of drug levels to discern pharmacokinetics (182) appears to reduce the risk of nephrotoxicity. Use of liposomal preparations of amphotericin B reduces the risk of renal damage (181). If patients are to receive contrast agents, hydration with sodium bicarbonate solutions have been shown to reduce the risk of subsequent renal dysfunction (183). Although N-acetylcysteine has been purported to reduce the risk of contrast-induced ARF (184), the observed results may be a reflection of the activation of creatinine kinase or an increase in the tubular secretion of creatinine (181). Medications such as “low-dose” dopamine or fenoldopam, which increase renal blood flow or loop diuretics, have no impact on preserving renal function in high-risk patients and should be avoided (181).
Debridement of Necrotic Tissue and Fracture Stabilization
The presence of dead or devitalized tissue appears to predispose patients to the development of MODS, hence timely debridement of dead tissue is an important component in prevention. Early surgical fixation of major lower extremity fractures will result in a lower incidence of ARDS and pneumonia. However, “damage control” orthopedics has recently gained popularity and is a concept whereby fractures are initially treated with external fixation; definitive therapy occurs later when the patient is more stable. The inflammatory response appears to be attenuated in these patients, and the incidence of organ dysfunction is no higher compared to patients undergoing definitive therapy (185).
Infection
Sepsis is an important cause (or correlate) of MODS, making it imperative to rule out an active source of infection in the critically ill population. Prompt hemodynamic resuscitation and timely administration of antibiotics are crucial, as well as source control (146).
Intra-abdominal Sepsis
There are many diseases presenting with intra-abdominal sepsis treated primarily by surgery (i.e., perforated viscus); these will not be discussed herein. Rather, we will review disease processes those have both medical and surgical aspects requiring ICU care, close monitoring, resuscitation, and possible intervention.
Pancreatitis. Pancreatitis is a common disease among both medical and surgical ICU patients. There have been important changes over the past several decades in the management of pancreatitis: overall there is consensus regarding nonoperative management in the setting of acute severe pancreatitis that mainly includes liberal fluid resuscitation, early enteric nutrition, and avoidance of empiric antibiotics without objective evidence of infection (186). There is less endocrine and exocrine insufficiency in patients managed nonoperatively in severe acute pancreatitis compared to operative management (187). Necrosis of pancreatic parenchyma or extrapancreatic tissue is present in 10% to 20% of patients with acute severe pancreatitis and is associated with high morbidity and mortality rates. Nonoperative management should still be the same as recommended for severe acute pancreatitis.
Urgent endoscopic retrograde cholangiopancreatography (ERCP) is indicated when cholangitis is suspected. A recent Cochrane review showed no benefit of antibiotics in preventing infection of necrotic pancreatic material except when imipenem was considered; in this case a significant decrease in pancreatic infection was found. None of the studies included in the review were adequately powered for mortality (188).
As a result of a shift from early surgical debridement to a staged, minimally invasive, multidisciplinary, step-up approach, outcome following necrotizing pancreatitis has improved substantially (189). Diagnosing infected necrosis is important, as it typically requires invasive intervention. Gas in a necrotic collection demonstrated on imaging investigations is considered proof of infection, occurring in around 40% of patients with infected necrosis. Utilization of fine-needle aspiration (FNA) may be helpful in patients without radiologic evidence of infection. However, there is a clear lack of consensus on the use of FNA to diagnose infected necrotizing pancreatitis (190). Common practice is that FNA must be considered for patients who fail to recover from organ dysfunction and without signs of findings of infection on radiologic studies. Positive FNA result hence documented infection would warrant staged multidisciplinary “step-up” approach (189). Once infection is documented, there is good evidence—and consensus—about the approach to treatment.
The initial step in the “step-up” approach is drainage, either percutaneous or transluminal, by means of cystgastrostomy, or cystduodenostomy, followed by surgical or endoscopic transluminal debridement if needed. The response rate to initial drainage is up to 30%; debridement is delayed until the acute necrotic collection has become encapsulated, typically no earlier than 6 to 8 weeks.
Acute Mesenteric Ischemia. This is another major source of intra-abdominal sepsis, and may occur due to mesenteric arterial thrombus, embolus, or venous thrombus; these are dealt with elsewhere. On the other hand, acute mesenteric ischemia may occur as a secondary problem due to ongoing sepsis, in the setting of underlying mesenteric atherosclerosis. This scenario is the least common type of mesenteric ischemia, also known as “low flow state” ischemia or “nonocclusive mesenteric ischemia”; however, it has higher morbidity and mortality compared to thromboembolic ischemia (191). Essentially, patients become septic from a source other than the abdomen and, if this progresses, the untreated hypotension and hypovolemia can cause ischemia in the gastrointestinal system.
Treatment of nonocclusive mesenteric ischemia relates to optimization of hemodynamics, elimination of vasopressor treatment, and correction of any systemic factors contributing to shock. Patients with peritoneal signs on examination or evidence of bowel necrosis or perforation on CT scan require surgical exploration to remove any source of sepsis; a second-look laparotomy should be performed routinely.
Antibiotic Duration for Intra-abdominal Infections. This has been investigated after successful treatment of intra-abdominal infection. In patients with intra-abdominal infections who have undergone an adequate source-control procedure—via surgical or percutaneous drainage—the outcomes after fixed-duration antibiotic therapy (approximately 4 days) are similar to those after a longer course of antibiotics (approximately 8 days); the latter extending until after resolution of physiologic abnormalities (192).
Pulmonary Sepsis
Ventilator-associated pneumonia (VAP) can play a role in the development and course of MODS. Proven preventive measures include noninvasive positive pressure ventilation, elevation of the head of the bed (193), continuous subglottic suctioning, in-line suctioning systems, weaning protocols, optimization of sedation with daily “wake-ups” or protocolized sedation for the patient (194,195), and chlorhexidine oral rinse.
VAP can be difficult to diagnose because radiographic findings are commonly used to secure the diagnosis and the findings are inaccurate. Recently, a ventilator-associated event (VAE) algorithm has been developed that utilizes duration of mechanical ventilation and new alterations in FiO2 and PEEP as well as new administration of antibiotics to define complications of mechanical ventilation. At this point it is intended as a surveillance tool but, because most VAEs are associated with pneumonia, ARDS, atelectasis, or congestive heart failure, the tool can provide insight into quality of care at an institution (196).
Selective digestive decontamination (SDD) is a technique by which topical nonabsorbable antibacterial and antifungal agents (usually with a concomitant 3- to 5-day course of systemic antibiotic therapy) are applied to the oropharynx and proximal bowel in mechanically ventilated patients to reduce the incidence of nosocomial infections, organ dysfunction, and mortality. A meta-analysis of 57 randomized controlled trials demonstrated a favorable effect on bloodstream infections and mortality (197,198). Fears concerning the emergence of resistance organisms do not appear to be well founded in ICUs where there is a low level of antibiotics resistance (199) although in one trial, there was a gradual increase in aminoglycoside-resistant gram-negative bacteria (200). SDD may very well reduce the incidence and prevalence of colonization with resistant gram-negative aerobic bacteria (201). However, the use of SDD in the United States does not enjoy widespread popularity for reasons that remain unclear.
Catheter-Related Sepsis
Catheter-related bloodstream infections (CRBSIs) may contribute to the development and propagation of MODS. Proven strategies to reduce the risk of CRBSIs include handwashing prior to catheter insertion, use of maximum barrier precautions (cap, mask, sterile gloves and gown, and a large sterile drape that covers the patient), use of an alcohol-based chlorhexidine skin preparation solution, avoiding the femoral site for catheter insertion, and removing catheters when no longer needed (202,203). If these measures are ineffective in reducing the risk of infection, catheters with antiseptic surfaces (204,205) or impregnated with antibiotics (206) or the use of chlorhexidine dressing sponges can reduce the risk of infection.
Other Sources of Sepsis
Many other inapparent sources of infection in critically ill patients may contribute to the development of MODS. Some of these sources of infection include purulent sinusitis, suppurative thrombophlebitis, otitis media, perirectal abscess, epididymitis, prostatitis, calculous or acalculous cholecystitis, meningitis or brain abscess (particularly after instrumentation of the CNS), prosthetic intravascular graft infection, lower or upper urinary tract infection, and endocarditis. Physical examination and appropriate laboratory and radiographic studies should exclude these conditions.
Nutrition Support
Malnutrition can contribute to the morbidity and mortality of sepsis and MODS. Proteolysis is a prominent finding in sepsis and, although it cannot be suppressed by infusing amino acids, anabolism may be achieved by appropriate nutritional support. Furthermore, catabolism is mediated by endogenous catecholamines, and administration of β-adrenergic blocking agents can reverse the hypermetabolic response and protein catabolism (207). Early nutritional support may be beneficial in patients at risk for developing MODS (208). Data exist to support enteral nutrition (EN) over parenteral nutrition (PN); therefore this should be the choice of feeding unless there is contraindication to use of the GI system. Nutritional support should be initiated within the first 24 to 48 hours following admission (209), although the degree of caloric intake that is required is controversial (see below) (210).
Although current practice supports giving 20 to 25 kcal/kg/day (2 to 5 g/kg/day glucose, 0.5 to 1.0 g/kg/day of fat, and 1.2 to 1.5 g/kg/day of protein) in critically ill patients, a recent prospective randomized demonstrated that permissive “underfed” (moderate nonprotein calories but similar amount of protein compared to standard feeding) resulted in similar 90-day mortality rates and hospital and ICU lengths of stay despite the fact that caloric intake was reduced by 36% in the underfeeding group (211). Protein intake was similar between the two groups. It is to be determined whether current practice will change regarding nonprotein caloric intake in critically ill patients.
Insulin therapy of hyperglycemia has evolved in the critically ill patients. A meta-analysis evaluated 26 trials involving 13,567 patients. Among the 26 trials that reported mortality, the pooled relative risk (RR) of death with intensive insulin therapy (titration of insulin to keep blood glucose between 80 and 110 mg/dL) compared to less “tight” glucose control was not significant. Among the 14 trials that reported hypoglycemia, the pooled RR associated with intensive insulin therapy was significantly higher. The ICU setting was a contributing factor, with patients in surgical ICUs appearing to benefit from intensive insulin therapy whereas patients in the other ICU settings did not. Intensive insulin therapy significantly increased the risk of hypoglycemia and conferred no overall mortality benefit among critically ill patients (212).
Specialty Formulas
A number of enteral nutritional formulas are available that provide specific nutrients: glutamine, peptides, arginine, omega-3 fatty acids, nucleic acids, and antioxidants (e.g., vitamins E and C, β-carotene). Arginine is the substrate for NO synthase and is important in lymphocyte proliferation and wound healing (213). Omega-3 fatty acids change membrane lipid composition and can alter the inflammatory response (214). Nucleic acids assist in the proliferation of lymphocytes and intestinal crypt cells, as well as DNA and RNA synthesis (213). Several enteral nutrition formulas are available that include combinations of these additives. The Canadian Critical Care Clinical Practice Guideline Committee (215) recommends that arginine and other “select” nutrients not be routinely used for enteral nutrition. In patients with ARDS, a formula supplemented with fish oil, borage oil, and antioxidants should be considered (215). Although glutamine should not be used in patients with shock or MODS, enteral glutamine should be considered in trauma and burn patients (215).
SUMMARY
Standard therapy for patients with MODS includes adequate cardiovascular resuscitation, identification and timely treatment of infection, early enteral nutrition, glucose control, individualized support for dysfunctional organs, and minimizing iatrogenic complications by following clinical practice guidelines based on evidence-based medicine for mechanical ventilation and prevention of VAP and CRBSIs (Table 52.4). Development of well-functioning ICU teams helps facilitate these paradigms of care. Improved outcome may be realized if patients at high risk for developing the syndrome can be identified earlier so that preventive measures can be instituted when appropriate. Because the pathogenesis of MODS involves numerous mediators, it is doubtful that all patients can be treated with a single agent or mode of therapy.
TABLE 52.4 Suggested Strategies for the Prevention of Multiple Organ Dysfunction Syndrome |
![]() |
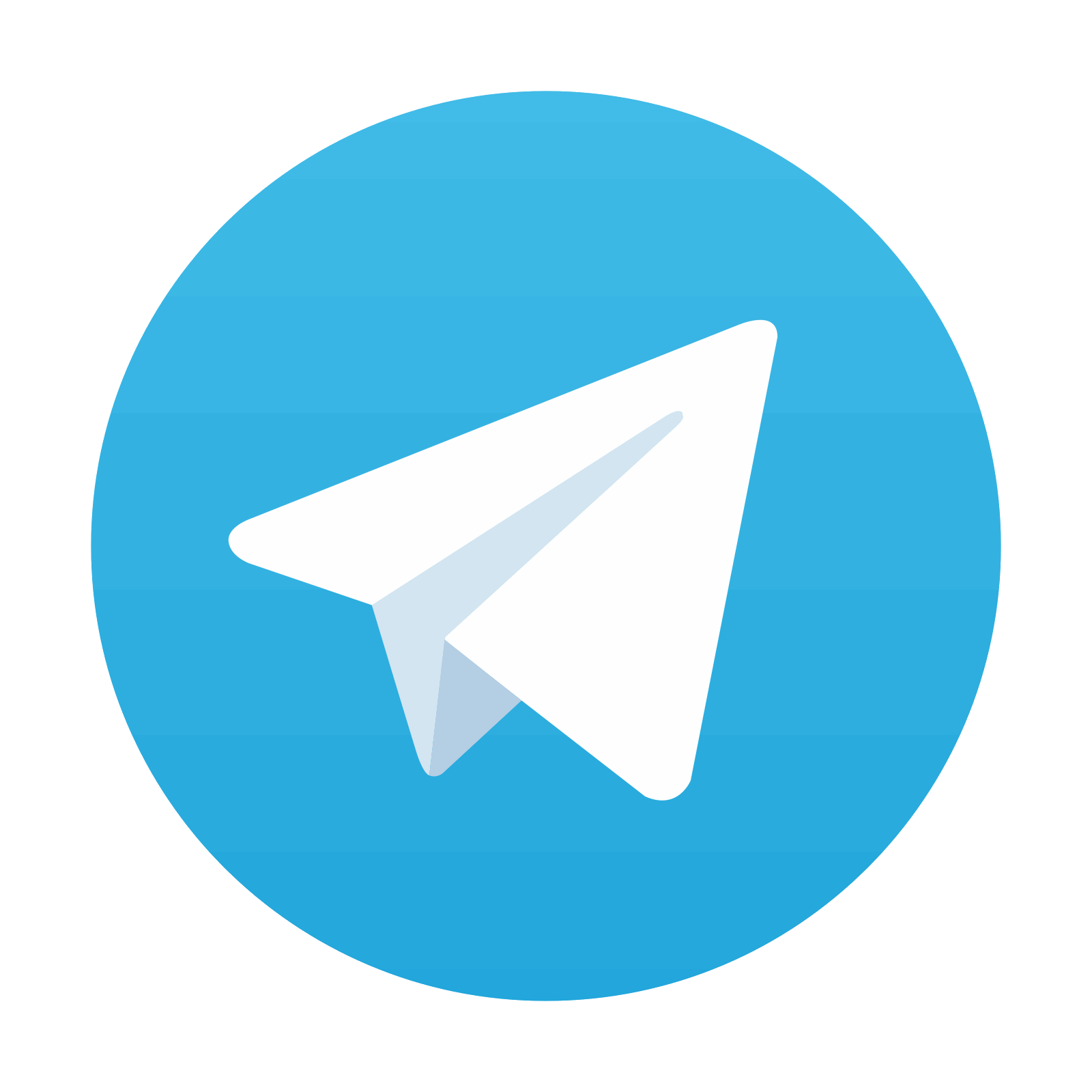
Stay updated, free articles. Join our Telegram channel

Full access? Get Clinical Tree
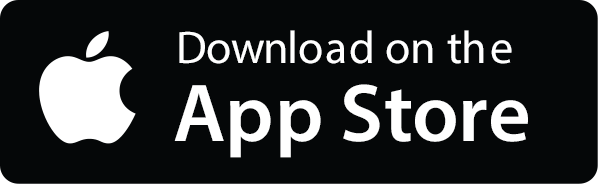
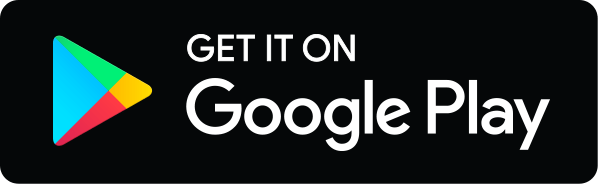