Questions
- 1.
- 2.
What are the basic principles of neuromuscular blockade monitoring?
- 3.
Why is a supramaximal stimulus used for neuromuscular blockade monitoring?
- 4.
Describe the clinically available devices used to monitor neuromuscular blockade.
- 5.
- 6.
How can you evaluate this patient who has no response to train-of-four stimulation?
A 50-year-old, healthy, 70-kg man (body mass index 24 kg/m 2 ) is undergoing an inguinal hernia repair. He is tracheally intubated after administration of 70 mg of rocuronium (1 mg/kg) and is receiving sevoflurane in oxygen/air and fentanyl. At 30 minutes into the procedure, the surgeon complains that the patient is “tight,” and an additional rocuronium dose of 20 mg (approximately 0.3 mg/kg) is administered. The surgeon announces 5 minutes later that he is finished. You apply train-of-four (TOF) stimulation over the ulnar nerve at the wrist, but no twitch responses are elicited.
1
Why is monitoring of neuromuscular blockade important whenever a neuromuscular blocking drug is administered?
Neuromuscular blocking drugs (NMBDs) are widely used to facilitate tracheal intubation and to provide and maintain optimal operating conditions. In the past, neuromuscular blockade was commonly evaluated on the basis of clinical criteria alone, such as a surgeon’s assessment of inadequate relaxation, patient movement, attempts at breathing “against the ventilator,” or observation of “curare clefts” in the capnograph tracing. For certain procedures, excessive doses of an NMBD may be administered. Because there is considerable variability among patients’ sensitivities to NMBDs, clinical criteria alone cannot be used to accurately asses depth of, or recovery from, neuromuscular blockade. A quantitative and documentable method of assessment is necessary. Monitoring neuromuscular blockade in response to administration of a NMBD allows titration of doses to the desired effect. Adequate relaxation can be achieved without administering unnecessary doses of a NMBD and recovery from relaxation becomes more predictable. Monitoring to ensure adequate recovery from neuromuscular blockade is important to prevent respiratory failure after tracheal extubation, which may occur as a problem in the postanesthesia care unit.
2
What are the basic principles of neuromuscular blockade monitoring?
Monitoring of neuromuscular transmission requires supramaximal stimulation of a motor nerve and measurement of the response evoked in the innervated muscle. NMBDs have no direct effect on muscle; if stimulation is applied directly over a muscle, that muscle contracts despite complete blockade at the neuromuscular junction. It is important to stimulate a motor nerve in a location where direct muscle stimulation cannot occur and only the indirectly evoked response (i.e., via the neuromuscular junction) is assessed.
It is essential to know which muscle to observe in response to indirect stimulation. When the ulnar nerve is stimulated, thumb adduction by the adductor pollicis brevis muscle is the appropriate site to monitor. Finger and wrist flexion should be ignored because they are likely due to direct stimulation if electrodes are placed over the forearm muscles.
During recovery from anesthesia, the most important muscles are the muscles of respiration and airway patency, but these muscles (e.g., diaphragm, laryngeal) cannot be monitored during clinical anesthesia. The ulnar nerve–adductor pollicis brevis combination is easily monitored and is used as a surrogate.
3
Why is a supramaximal stimulus used for neuromuscular blockade monitoring?
Motor nerves consist of numerous nerve fibers, each of which innervates a motor unit in the muscle. Because the muscle’s total response is required to assess neuromuscular blockade, all of the nerve fibers must be reliably stimulated. In this way, any decrement in muscle contraction is due to neuromuscular junction blockade and not due to a failure to stimulate all of the fibers in the motor nerve.
In a normal unparalyzed patient who has surface electrodes placed over the ulnar nerve, as the intensity (mA) of nerve stimulation is gradually increased, the force of contraction of the muscle increases until all of the nerve fibers are stimulated. Further increase in stimulus intensity does not result in further increase in evoked response. The stimulus producing a maximal muscle response is called the maximal stimulus. Stimuli of greater intensity are termed supramaximal. During neuromuscular blockade monitoring, supramaximal stimuli (usually 120% of maximal stimulus current) are used to ensure that all nerve fibers are stimulated. The maximal (and supramaximal) stimulus varies among individual patients, depending on the amount of tissue between the skin and the nerve. The negative stimulating electrode should always be placed distal to the positive one because this configuration results in the greatest muscle response.
4
Describe the clinically available devices used to monitor neuromuscular blockade.
In principle, neuromuscular blockade monitoring requires two devices: a peripheral nerve stimulator to deliver the supramaximal stimulus and a device, which may be mechanical or electrical, to measure the response evoked in the muscle.
Peripheral nerve stimulators
Stimulation of a nerve fiber is a function of the amount and duration of current applied. Modern peripheral nerve stimulators are designed to deliver a constant current, despite changes in resistance that may develop over time between the surface electrodes and the nerve. The current is delivered in square wave pulses of 200 μsec (0.2 msec) duration. At this pulse width, motor nerves but not sensory nerves are stimulated; this is because stimulation of sensory nerves requires a longer pulse duration (approximately 1 msec).
Peripheral nerve stimulators are the devices most commonly used to facilitate assessment of neuromuscular blockade in clinical settings. With only a peripheral nerve stimulator, the clinician must evaluate the force of contraction by observing or palpating thumb adduction. Such observations are difficult to quantify and are subject to considerable interobserver variability.
The following devices may be used to quantify evoked response.
Mechanomyography
Historically, mechanomyography (MMG) has been the “gold standard” for objective monitoring of a muscle’s evoked response. Stimulation of a motor nerve produces isometric contraction of the innervated muscle, commonly the adductor pollicis brevis. The mechanical force developed is measured using a force transducer connected to the thumb. For accurate measurements, such as for research purposes, it is necessary to preload the muscle with 200–300 g of tension, and the force vector (i.e., the direction of thumb movement) must be perpendicular to the force transducer. The force transducer is calibrated in kg-force, and the output of the force transducer is displayed graphically or digitally on a monitor. In an unparalyzed patient, delivery of supramaximal stimuli to the nerve results in a maximal (100%) response in the muscle. This response is considered to represent 100% and is used as a reference for subsequent evoked responses after the patient has received an NMBD.
Although a purpose-designed arm board with built-in force transducer has been commercially available, MMG is not practical for routine clinical use. The force transducer is bulky, the muscle must be preloaded with a specific tension, and the arm must be abducted and kept immobile so that there is no interference with movement of the thumb.
Electromyography
Electromyography (EMG) records the compound muscle action potential in response to peripheral motor nerve stimulation. Stimulating electrodes are placed over the peripheral motor nerve, and recording electrodes are placed over the innervated muscle. The magnitude of the evoked response is measured. Clinically, EMG is easier to use than MMG because muscle preloading and precise force vector measurements are unnecessary; both arms can be adducted at the patient’s side. As a result, EMG has replaced MMG in neuromuscular studies conducted more recently. Integrated EMG monitoring is commercially available only as a modality in the Neuromuscular Transmission Module of the GE S/5 monitoring system (GE Healthcare, Datex-Ohmeda S/5 NMT Module (Datex-Ohmeda Inc. P.O.Box 7550, Madison, Wisconsin, 53707. USA)). Similar to MMG, the EMG response is first calibrated to 100% in an unparalyzed patient, and subsequent responses are displayed as a percentage of the initial response.
Accelerography
Accelerography is based on the principle that force is the product of mass times acceleration (F = ma). If mass is kept constant, force (of contraction) is directly proportional to acceleration. Accelerography uses a piezoelectric transducer attached to the thumb (the mass of which remains constant) to determine the rate of angular acceleration in response to nerve stimulation. The size of the acceleration output signal is displayed digitally and graphically. Accelerography requires unobstructed thumb movement, similar to MMG. This technology is commercially available for clinical use and has been widely employed in clinical research studies. It provides a simple and objective method of assessing neuromuscular blockade. It eliminates interobserver variability associated with subjective clinical observation of evoked responses, on which peripheral nerve stimulators rely.
Kinemyography
Kinemyography (KMG) is clinically available as a modality in the Neuromuscular Transmission Module of the GE S/5 monitoring system. KMG uses a piezoelectric strip incorporated into a flexible U -shaped (“boomerang”) device that is placed between the thumb and index finger. The U -shaped device, mechanosensor, is designed to mimic a MMG-like preload. Movement of the thumb produces a redistribution of electrical charge across the piezoelectric membrane. The voltage produced by movement of the thumb is measured, processed, and displayed digitally and graphically on the monitor screen. Results have been shown to correspond closely with MMG, making it a reasonable option for clinical use.
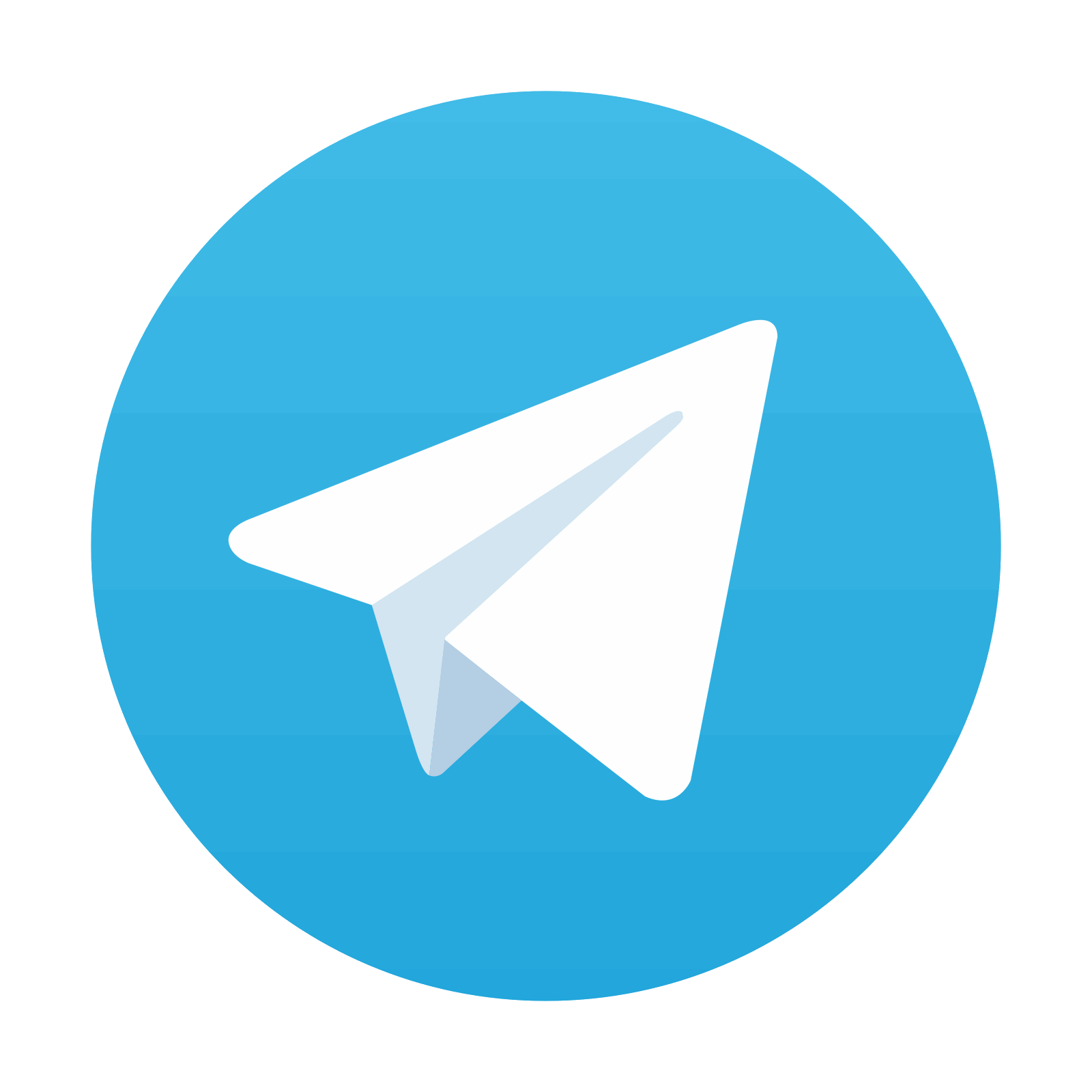
Stay updated, free articles. Join our Telegram channel

Full access? Get Clinical Tree
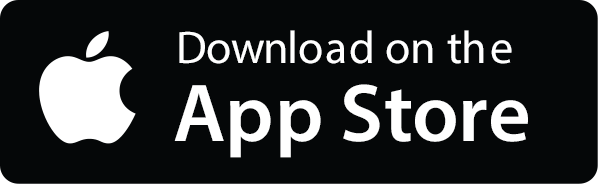
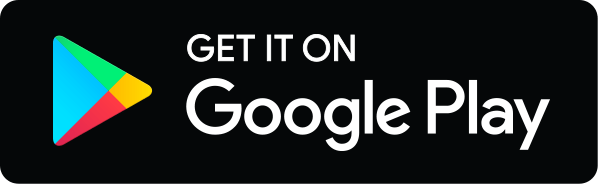