Chapter 5 Traditional blood pressure measurement by sphygmomanometer can be obtained by Doppler, palpation, auscultation, and oscillometric methods. Palpation allows for only systolic pressure determination, is time and resource intensive, and generally is used only in noisy environments (such as emergency medical services [EMS] transport) where measurement by oscillometric means may be difficult. Doppler is particularly helpful in identifying the presence of a systolic pulse in hypotensive individuals or in internally (i.e., atherosclerosis) or externally (i.e., compartment syndrome) flow-limited extremities. Auscultation, otherwise known as “manual” blood pressure measurement, remains a common, reliable, and reproducible method of blood pressure measurement 100 years after the Korotkoff technique was discovered. This method relies on placing the stethoscope at the antecubital fossa, inflating the blood pressure cuff, and recording the pressure at which auscultation of the pulse returns (the systolic blood pressure) and when it disappears (the diastolic blood pressure). Auscultation is generally considered to underestimate the systolic blood pressure and overestimate the diastolic pressure.1 Although accuracy has improved with standardization of the technology, this technique is occasionally prone to significant inaccuracy.2 The oscillometric technique measures oscillations in the pressure during deflation of the cuff; the maximal point of oscillation represents the mean arterial pressure. This is the most common noninvasive method of measuring blood pressure in the ED, commonly called the “automatic” blood pressure. This method has several advantages over auscultation, including less susceptibility to noise, the lack of necessity for specific placement of a transducer over the artery, less resource usage, regular measurement intervals, and the ability to embed readings into other clinical monitors. Comparisons of auscultation and oscillometric measurement with intra-arterial measurement have found good agreement (mean percent error ≤3%).3 Cuff-based blood pressure measurement is limited by technique and clinical circumstances. The quality of the device, cuff size, body positioning, and arm positioning are all components of the measuring technique that can affect the accuracy. Clinical circumstances such as obesity, dysrhythmias, and extremes of age (young children and elderly) may also affect the accuracy.4 The ease of use of oscillometric blood pressure measurement makes this modality ideal for ED noninvasive monitoring. Other methods of providing continuous, noninvasive blood pressure monitoring often rely on partial radial artery compression or arterial loading counterpressure and offer results comparable to those of an arterial catheter with the benefit of added mobility.5,6 Newer continuous, noninvasive blood pressure monitors have the advantage of providing additional advanced hemodynamic measures, such as stroke volume and cardiac output,7 which may be beneficial in critically ill patients. Early ED use of pulse oximetry came through extrapolation from anesthesiology literature, which demonstrated less frequent and shorter episodes of desaturation and earlier recognition of hypoxia during or after anesthesia.8,9 In the ED, pulse oximetry is largely used in initial triage of patients, particularly those with cardiopulmonary complaints, or in the monitoring and management of patients who are sedated or critically ill, particularly with respiratory distress or during endotracheal intubation. In the latter case, pulse oximetry has been shown to reduce the frequency of hypoxic episodes. SaO2 values at or below 96% have been shown to be 100% sensitive for detection of hypoxia (PaO2 < 70 torr), but such a cutoff can be different in patients with obstructive lung disease.10,11 Pulse oximetry has several important clinical limitations. By design, pulse oximetry measures only two wavelengths. At these wavelengths, traditional pulse oximeters are unable to distinguish oxyhemoglobin and deoxyhemoglobin from two other important dyshemoglobinemias: methemoglobin (MetHb) and carboxyhemoglobin (COHb). Multiwavelength CO-oximeters are now available that can distinguish the four wavelengths.12 Oxygen saturation by pulse oximetry will be equivalent to actual saturation, presuming insignificant amounts of MetHb and COHb exist in the blood. In the setting of MetHb or COHb exposure, the pulse oximeter will read falsely elevated levels of SaO2 even at relatively high exposures. In heavy smokers, COHb levels of 3 to 15% can commonly be found, but this has little impact on the accuracy of the SaO2. Although signal artifact is probably the most common technical limitation to adequate measurement, other limitations include low perfusion and therefore low pulsatile component, ambient light, deep skin pigmentation, methylene blue, and nail polish. Averaging saturation measurements over several seconds limits the impact of motion artifact and improves the accuracy. The concentration of carbon dioxide (CO2) in an exhaled breath is intrinsically linked to tissue metabolism, systemic circulation, and ventilation.13 Capnography is the graphic record, represented as a waveform, or capnogram, of the instantaneous CO2 concentrations in respired gases during a respiratory cycle.14,15 Capnography provides continuous, real-time, breath-to-breath feedback on the clinical status of the patient, allowing the clinician to determine the baseline ventilatory status and to track changes over time.16 Capnography is also a diagnostic monitoring modality because certain disease conditions are associated with characteristic waveforms.14–17 Although the concentrations of CO2 can be displayed continuously throughout the respiratory cycle, by convention only the maximum CO2 concentration at the end of each tidal breath, the ETCO2, is ordinarily displayed. Capnometry is the quantitative measurement of ETCO2 displayed as a number without a waveform. Colorimetric detectors use color scales to estimate ranges of ETCO2 but are not sufficiently accurate to give quantitative measurements. Their use is therefore limited to confirmation of correct endotracheal tube (ETT) placement and its continuous location in the trachea. Although originally used during general anesthesia in the operating room, ETCO2 monitoring has now become a standard monitoring modality in the ED and nonhospital medical setting. Colorimetric CO2 detectors use pH-sensitive filter paper impregnated with metacresol purple, which changes color from purple (<4 mm Hg CO2) to tan (4-15 mm Hg CO2) to yellow (>20 mm Hg CO2) depending on the concentration of CO2.13,15 (See Chapter 1.) The indicator, housed in a plastic casing, is inserted between the ETT and the ventilator bag and detects changes on a breath-by-breath basis.13 These detectors are also inexpensive and easy to use and should be available in every ED and on every EMS unit that performs intubation for confirmation of ETT placement if capnography or capnometry is not available. In patients with normal cardiopulmonary function, there is a close correlation between alveolar CO2 (PACO2) and arterial CO2 (PaCO2). The ETCO2 is usually 2 to 5 mm Hg less than the PaCO2 because of the dilution of the end-tidal gases by physiologic dead space gas.18,19 Conditions that affect ventilation-perfusion ratios (including pulmonary embolism), cardiac arrest, hypovolemia, obstructive lung disease, and the lateral decubitus position can widen the Pa-ETCO2 gradient.18–22 Several recent studies, however, have shown high concordance between ETCO2 and PaCO2 in adult asthmatics and in children with moderate and severe respiratory distress from bronchiolitis, asthma, and pneumonia.20 Although ETCO2 may not accurately reflect the absolute PaCO2 in critically ill patients, it is still valuable in detecting ventilatory trends and identifying sudden airway events. Analysis of the shape of the capnogram can yield valuable diagnostic information.14–16 A normal capnogram has four phases (Fig. 5-1A). Phase 1-2 represents a CO2-free portion of the respiratory cycle. Most often this is the inspiratory phase, although it may represent apnea or a disconnection of the device from the patient. An elevation of this baseline above zero implies rebreathing of CO2, as in increased dead space in the circuit or contamination of the sensor.14–16
Monitoring the Emergency Patient
Noninvasive Blood Pressure Measurement
Pulse Oximetry
End-Tidal Carbon Dioxide Monitoring
< div class='tao-gold-member'>
Stay updated, free articles. Join our Telegram channel

Full access? Get Clinical Tree
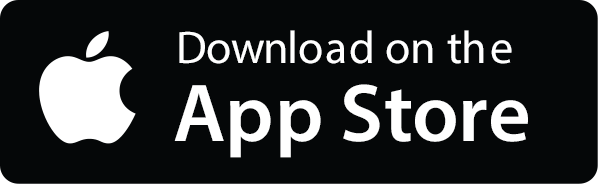
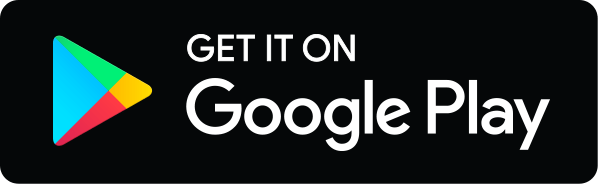