Fig. 6.1
Recording methods of spinal cord potentials (SCPs). A catheter electrode is inserted into the dorsal epidural space using the same technique as that for the continuous epidural block at a level corresponding to the spinal segment to be monitored [8]. To exclude ECG contamination, stimulation pulses are triggered by a QRS component of ECG with a delay of 0.3–0.5 s, when the triggered pulses coincide with the end of a T wave. In this manner, evoked SCPs are recorded in the electrically silent phase (0.5–0.7 s) between T and P waves [9]. (a) A catheter (1 m diameter) equipped with several Ag-AgCl electrodes (A-1), and platinum–iridium electrodes (A-2), which are designed for spinal cord stimulation, are also available. (b) An illustration of a catheter electrode inserted into the epidural space. (c) The relation between ECG and electrical stimulation [14, 15]
The peripheral nerve trunk is electrically stimulated to evoke SCP responses. For monitoring of cervical spinal cord activity, epidural catheter electrodes are inserted into the cervical epidural space close to the cervical enlargement. Next, the brachial plexus or the radial, ulnar, or median nerve is stimulated (segmentally evoked SCPs, Fig. 6.2a). To monitor lumbar spinal cord function, the recording electrodes are placed close to the lumbar enlargement, and the common tibial or peroneal nerve is stimulated (segmentally evoked SCPs, Fig. 6.2a, c) [7, 9, 12].
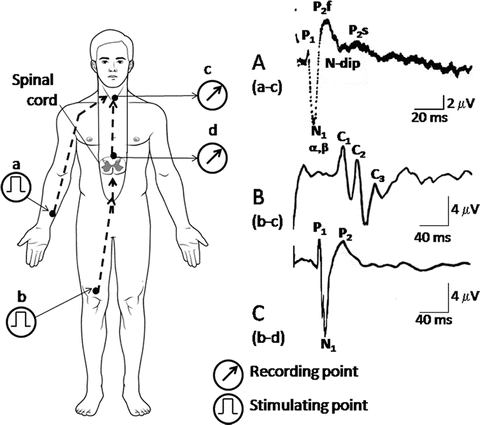
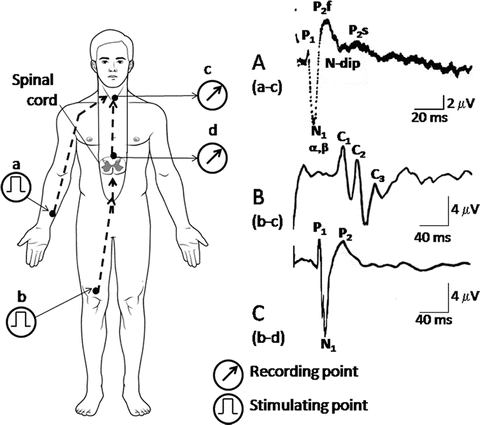
Fig. 6.2
Recording of spinal cord potentials (SCPs). A peripheral nerve trunk is electrically stimulated (see Fig. 6.1). Recording electrodes are inserted into the epidural space to monitor the dorsal spinal activity. Dysfunction of the spinal cord is reflected as abnormalities in the waveforms, such as prolongation of peak latencies and depression or augmentation of amplitudes. The waveforms of the segmental SCPs recorded in the cervical and lumbosacral enlargements are very similar to each other. In the ascending evoked SCPs, complex positive waves (C1, C2, and C3) are recorded, but N1 and P2 waves are hardly noticed. (a) The waveforms of segmental evoked SCPs recorded from the cervical enlargement (c) by stimulation of the ulnar nerve (a). (b) Ascending evoked SCPs recorded from the cervical enlargement (c) by stimulation to the common tibial nerve (b). (c) Segmental evoked SCPs recorded from the lumbar enlargement (d) by stimulation at the common tibial nerve (b). P1: action potential of spinal nerve roots. N1: synchronized activities of interneurons. P2: primary afferent depolarization (PAD). Sometimes, P2 splits into two components, first (P2f) and second (P2s) components. N-dip: negative dip driving P2f and P2s [8, 22, 23]
The waveforms of the segmental SCPs recorded in the cervical and lumbosacral enlargements are very similar. Evoked SCPs can be recorded at the level of the cervical enlargement by the stimulation of a nerve trunk in the lower limb (ascending evoked SCPs; see Fig. 6.2b). The waveforms of SCPs recorded at the lumbar enlargement by epidural stimulation of the cervical spinal cord are similar to those of the segmentally evoked SCPs (descending evoked SCPs; see Fig. 6.2a, c) [8, 9, 10, 14, 15, 22, 23].
The summated electrical potentials travel along the spinal cord in response to SCS in the epidural space of the upper or lower spinal segments, cauda equina, or from peripheral nerve stimulation. Thus, the human SCPs can be recorded from the cervical epidural space in response to cauda equina stimulation at the L3–4 vertebral level or SCS by an electrode situated in the epidural space close to the lumbar enlargement (ascending SCPs; see Fig. 6.2b, c) and vice versa (SCS from cervical epidural space and recording at the lumbar enlargement level) (descending SCPs) [8, 10, 15].
Recording Electric tc-MEPS and Magnetic tc-MEPS for Intraoperative Monitoring
In transcranial electrical stimulation , electrodes are placed at C3 or C4 on the scalp, and a train of square wave stimulation pulses (with a pulse duration of 0.02–0.2 ms and an interpulse interval of 0.2 ms) are applied at an intensity of 250–1000 V [24–26]. Because of the use of high-voltage stimulation to obtain electric tc-MEPs, durable electrodes such as corkscrew-shaped electrodes are used for stimulation so as to avoid scalp burns. For magnetic tc-MEPs, a magnetic coil is used for stimulation. For both forms of stimulation, EMG (CMAP) responses are usually recorded from the abductor pollicis brevis muscle (upper limb) or the tibialis anterior muscle (lower limb) using needle or surface electrodes [24–26].
In response to pulse train stimulations, giant pyramidal cells are directly depolarized generating D waves [27–29]. However, during magnetic stimulation, interneurons are depolarized first and the firing of giant pyramidal cells follows sequentially generating several I waves at 1.5- to 2.0-ms intervals [28, 29]. Both D and I waves descend the pyramidal tract as a group of several spiky waves (multiple descending volleys), and the spinal motor neurons are stimulated by these piston-like multiple descending volleys, resulting in summation of excitatory postsynaptic potentials (EPSP) [18, 29–31]. Thus, the spinal motor neurons are excited with a few milliseconds of delay (Fig. 6.3) [18, 19, 28–33].
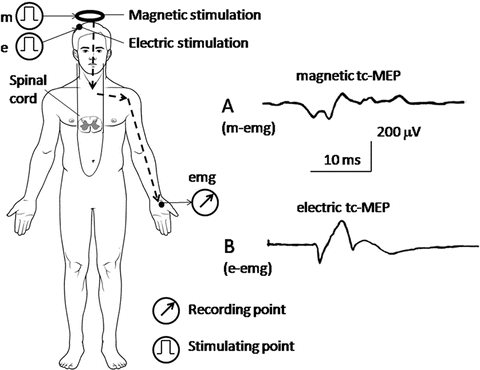
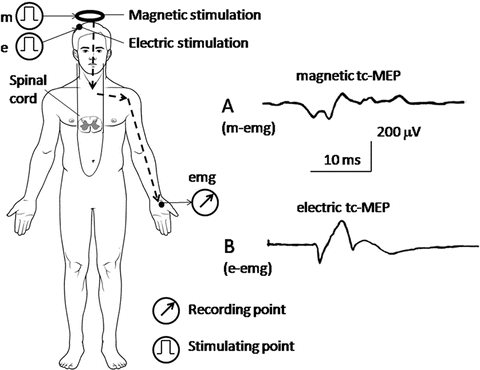
Fig. 6.3
Recording of transcranially stimulated motor-evoked potentials (tc-MEPs) . The brain is stimulated electrically or magnetically, and the evoked EMG (motor-evoked potential [MEP]) is recorded from the abductor pollicis brevis muscle (upper limb) or the tibialis anterior muscle (lower limb). (a) Magnetic tc-MEP, which is stimulated magnetically at the parietal cranium (m) and recorded from the abductor pollicis brevis muscle (m-emg). (b) Electric tc-MEP, which is stimulated electrically at the contralateral scalp, C3 or C4 (e), and recorded from the abductor pollicis brevis muscle (e-emg)
Convulsions are occasionally induced during brain stimulation for a clinical examination in the arousal state [32], but they are rare during stimulation for intraoperative monitoring under general anesthesia [33, 34]. Surgical procedures on the spine or spinal cord should be performed under general anesthesia with adequate monitoring of spinal cord function [35].
Recording tc-SCPS for Intraoperative Monitoring
Transcranial stimulation also results in electrical changes in the SCP recordings, which appear to provide better evidence of spinal motor function than tc-MEPs [18, 19, 27–29]. The methods of electric and magnetic stimulation are described for the respective tc-MEPs. However, the methods of recording the tc-SCP responses are the same as those described for the segmental SCPs [8–10, 17, 18].
When acquiring magnetic tc-SCP responses, the generation of multiple descending volleys in the pyramidal tract as well as the process of electric summation in the dorsal horn are clearly depicted [18, 19, 27, 29]. These phenomena are never evident when acquiring magnetic tc-MEP responses because only evoked EMG activity can be observed [14, 18, 19]. Therefore, magnetic tc-SCPs may provide fine data quality when monitoring spinal motor function (Fig. 6.4).
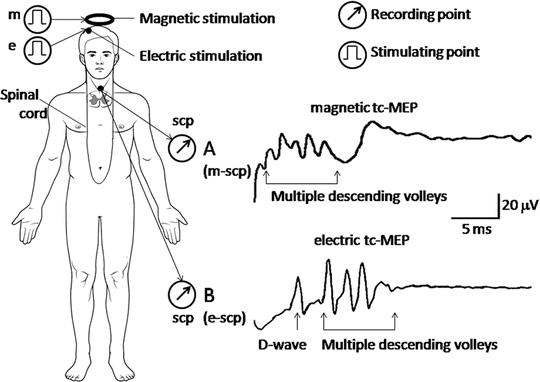
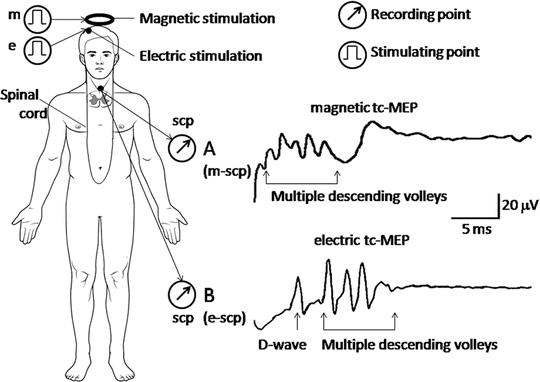
Fig. 6.4
Recording of transcranially stimulated evoked spinal cord potentials (tc-SCPs). The brain is stimulated magnetically or electrically, and the resulting spinal cord potentials (SCPs) are recorded with epidural electrodes. Both forms of stimulation evoke a group of several spiky waves (multiple descending volleys) descending the pyramidal tract. The volleys result in the summation of excitatory postsynaptic potentials (EPSPs). (a) Magnetic tc-SCP, which is stimulated magnetically at the parietal cranium (m) and recorded from the spinal cord (scp). (b) Electric tc-SCP, which is stimulated electrically at the contralateral scalp, C3 or C4 (e), and recorded from the spinal cord (scp)
Anesthetics Used in Spinal Cord Monitoring
Many general anesthetics , especially inhalation anesthetics, suppress spinal electrical activity, thereby reducing the amplitudes and prolonging the latencies of SSEPs, SCPs [36–39], and tc-MEPs. Therefore, the use of intravenous anesthetics, including low-dose propofol, ketamine, fentanyl, and remifentanil, are usually recommended for surgeries that use spinal cord monitoring. Also, anesthesia can suppress spinal electrical activity, leading to incorrect judgments [19, 23, 24, 40, 41]. In light of this, simultaneous monitoring of the bispectral index (BIS) is recommended for maintenance of adequate anesthesia depth (40–60 % in BIS) [42]. Administration of a muscle relaxant is desirable when recording SSEPs and SCPs because the use of relaxants diminishes the presence of muscle contractions and noise due to EMG artifact. Muscle relaxants, however, interfere with MEP monitoring.
Intravenously administered anesthetics, which are mixed with venous blood, stream into the heart (right atrium). After circulating through the lungs, the anesthetics return to the heart (left atrium) with minimal loss or consumption. Thus, most of the anesthetics enter the aorta. When the aorta is occluded during a surgical procedure, blood flow in the distal region of the occlusion becomes very small, and blood is pooled in the proximal region, where 30 % of the circulating blood volume is distributed [43]. Therefore, when intravenous anesthetics are continuously infused using a syringe pump, the concentration of an anesthetic in the proximal region of the aortic occlusion elevates two- to fourfold [44]. This elevation results in a deeper anesthetic state than was assumed before the aorta occlusion, leading to misjudgments. The monitoring of BIS is therefore helpful in realizing correct judgments in such cases [45].
Case Studies (Clinical Applications)
Spinal cord monitoring by SCP, tc-MEP, or tc-SCP is utilized during spinal surgery to assess the force on spinal neurons caused by traction, the stress or injury caused by surgical manipulation, the stress or damage due to ischemia, and hypofunction under conditions of hypothermia. Representative cases of each clinical application are presented below.
Traction force on the spine and spinal cord produces immediate spinal cord ischemia as a result of blood vessels being stretched. The force can reduce the amplitudes of SCPs by over 50 %. Amplitude reduction in the SCP due to ischemia can occur with or without prolongation of latency. Based on findings obtained from SCP monitoring, a nonharmful traction force on the spine can be determined. Consequently, a spinal cord injury was able to be prevented in the following case involving open traction and fixation of the spine to correct idiopathic scoliosis (Fig. 6.5) [46].
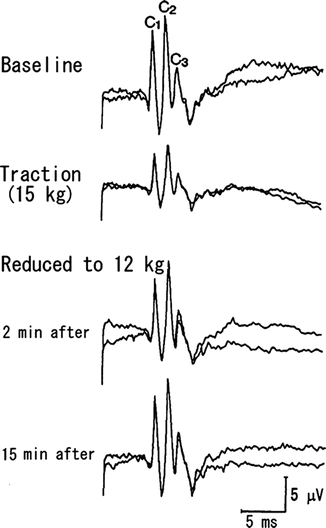
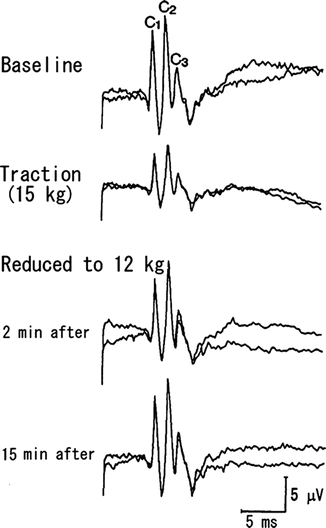
Fig. 6.5
Clinical application of SCP for spine traction . Open traction and fixation of the thoracic spine to correct idiopathic scoliosis in a 26-year-old female. Anesthesia was maintained with fentanyl and ketamine. The cauda equina (L4) was supramaximally stimulated by electric pulses. Ascending SCPs were recorded from the posterior epidural space at the C7 spinal cord level. Immediately after traction of the spine at 15 kg, the amplitudes of C1, C2, and C3 were reduced to 47.0, 47.5, and 49 %, respectively. These reductions in amplitude recovered within 15 min after the traction force was reduced to 12 kg. In this case, the latency did not change. Reprinted with permission from Fujioka et al. [46]
The surgical manipulation during resection of spinal cord tumors causes direct and/or indirect mechanical stress or injury to spinal neurons, which can be determined by using tc-MEP monitoring. When spinal cord damage occurs, the amplitudes of the tc-MEP responses drop and the stimulation thresholds elevate. Based on observations of the tc-MEPs, alterations to the tumor resection can be recommended so as to avoid postsurgical motor dysfunction (Fig. 6.6). When amplitude reductions exceed 50 % during the resection, the surgical approach or the resection size needs to be reconsidered: should the resection be continued, should a smaller resection be performed, should the angle or direction of the section be changed, or should the resection be discontinued altogether? Also, based on the final findings from the tc-MEP monitoring, the level of postsurgical motor dysfunction can be predicted [15].
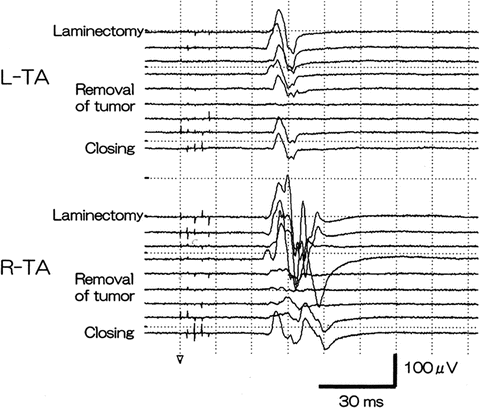
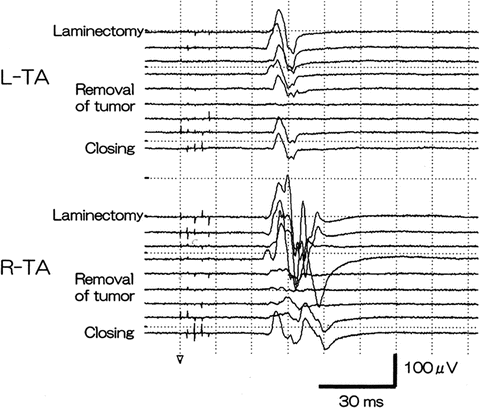
Fig. 6.6
Changes in tc-MEPs during spinal cord tumor resection. A 38-year-old male patient with a spinal cord tumor in the T5–6 area underwent tumor resection. Anesthesia was maintained by TIVA. While monitoring tc-MEPs, electric stimulation was performed with a five-pulse train (50–100 μs of duration, 2-ms intervals, 600 V) on the scalp (C3 and C4), and an EMG of the tibialis anterior (TA) muscles was recorded. During tumor resection, the amplitudes decreased, but they recovered immediately after the surgical approach was altered. The tumor resection was continued from the other direction. Thus, postsurgical motor weakness was not evident. Reprinted with permission from Fukaya et al. [15]
Aortic surgery usually requires aortic cross-clamping , which often results in spinal cord ischemia [47, 48]. Because of the prompt response to ischemia, SCPs are monitored during aortic surgery to assess the severity of ischemic stress to the spinal cord. Sequential changes in segmental SCPs during surgery for aortic aneurysm are shown in Fig. 6.7. Ischemia due to aortic cross-clamping caused a rapid decrease in the amplitude and an increase in the latency of the responses. The waveforms recovered quickly after declamping. However, the duration of ischemia that is tolerable or reversible when a reduction/abolition of the SCP occurs without any resulting postsurgical neurological symptoms is still not clear (see Fig. 6.7).
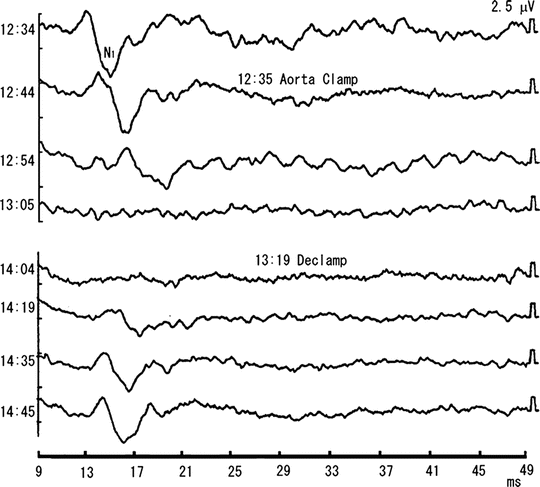
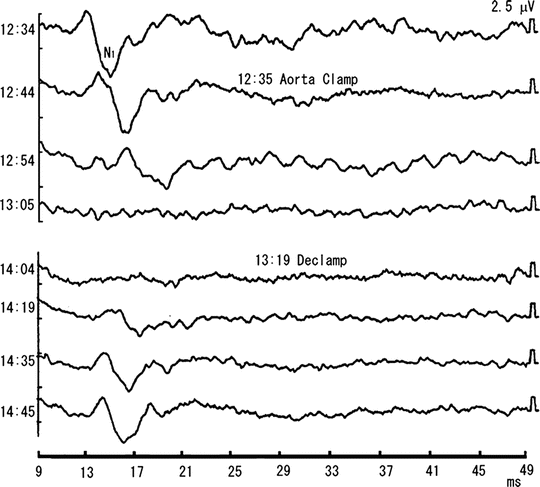
Fig. 6.7
Effects of aortic cross clamping on segmental SCPs. A 71-year-old man underwent abdominal aortic surgery. Segmental SCPs were recorded from the epidural space at the T12/L1 vertebral level, and the common tibial nerve was stimulated supramaximally at the popliteal fossa. Anesthesia was maintained mainly with fentanyl and midazolam, and blood cooling was initiated by cardio pulmonary bypass. The N1 wave disappeared 30 min (13:05) after cross-clamping (12:35) and reappeared (14:12) after the declamping (13:19), with the time lag between its reappearance and the declamping being 53 min. The durations of aortic cross-clamping and that of the disappearance of SCP were 44 and 67 min, respectively. There were no neurological sequelae after surgery. Reprinted with permission from Kondo et al. [49], with modification
Thus, test cross-clamping before dividing an aneurysm is recommended (i.e., SCP is closely observed for 15 min after aortic cross-clamping). If a greater than 50 % reduction of the amplitude is observed, the surgeons should make repeated short-term releases of the clamp during the surgery so as to avoid long-term ischemia or should change to another bypass route [49]. This test can also be modified when monitoring via tc-MEPs or tc-SCPs. On the other hand, when no or only minimal recovery in the SCP is noted during the final observation, motor dysfunction corresponding to the amplitude decrease can be predicted. Because there are concerns that heparinization during aortic surgery causes an epidural hematoma, an epidural catheter electrode should be inserted at least 1 h before the surgery and extracted 1 day later when the effect of the heparin has dissipated.
Cardiovascular surgery is usually performed under moderate or deep hypothermia , which makes interpretation of waveform changes more complicated. The waveform of the SCP under moderate hypothermia responds very sensitively to a drop in body temperature with characteristic responses in the waveform under such conditions being prolongation of the latency, widening of the duration, and augmentation of the amplitude (Fig. 6.8) [49].
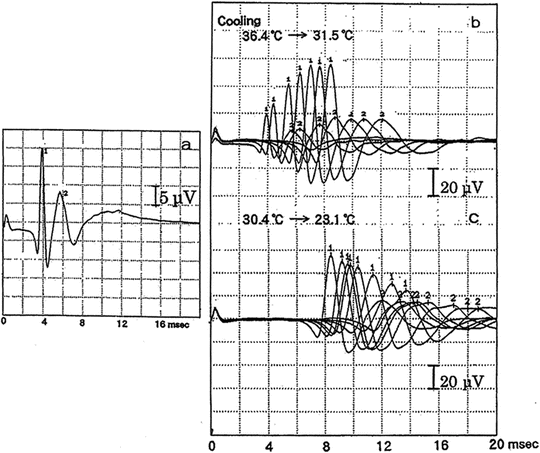
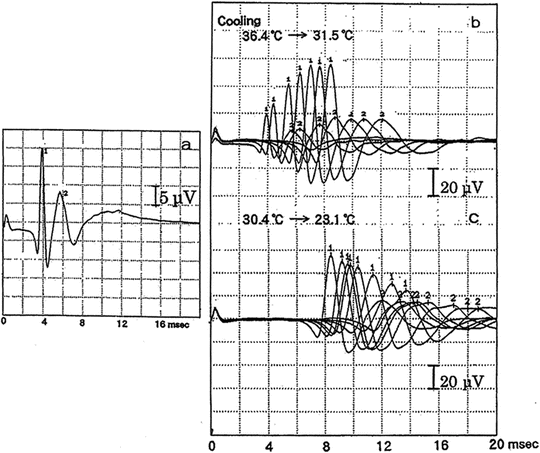
Fig. 6.8
Waveform changes of descending SCPs under moderate hypothermia . A 56-year-old patient underwent thoracoabdominal aortic surgery under hypothermic cardiopulmonary bypass. Descending SCPs were recorded from the epidural space at the T12/L1 vertebral level, and the spinal cord was supramaximally stimulated at the C6/7 vertebral level with epidurally inserted electrodes. Anesthesia was maintained with fentanyl and midazolam, and rectal body temperature was measured. Subsequent serial changes of N1 and N2 waves were superimposed: (a) before cooling; (b) SCPs during cooling process; (c) SCPs during rewarming process. Prolongation of the latency, widening of the duration, and augmentation of the amplitude are noted under moderate hypothermia. Reprinted with permission from Kondo et al. [49]
Interestingly, the change in the amplitude was biphasic when the body temperature was lowered even further: the amplitude gradually increased until around 30 °C, and then began to decrease under deeper hypothermia . The precise mechanisms of the biphasic response are still not clear. Under profound hypothermia (below 20 °C), the amplitude decreases or disappears. Under even lower body temperature of approximately 10 °C, the N1 wave is split into two peaks (not illustrated in Fig. 6.7) [49]. These changes recover to the baseline level immediately upon rewarming. Thus, body temperature measurements are indispensable for the interpretation of the waveform changes in the SCP monitoring.
Conclusion
In conclusion, besides basic routine monitoring of SSEPs and tc-MEPs [47], combined monitoring of other parameters such as tc-MEP or tc-SCP and MEPs may provide an accurate monitoring of spinal cord function during surgical manipulations of the spine or nearby structures depending on individual surgeries [50–52].
Recording of the spinal cord potentials recorded from the epidural space may add more precise monitoring of spinal cord functions in certain cases.
Appendix: Techniques and Physiology
Introduction
The Catheter Electrodes
Human spinal cord potentials (SCPs) can be recorded from the epidural space using the same catheter used for continuous epidural block. The epidural catheter electrode can be made simply by insertion of a stainless steel wire through the epidural catheter approximately 5 mm beyond its tip (Fig. A6.1a). This simple catheter has been used for the recording of spinal cord potentials in patients during surgical operations or for the stimulation of the spinal cord in patients with various spinal cord diseases [54]. An epidural catheter with three orifices on the side and three platinum wire electrodes was developed in our laboratory for multiple applications (Fig. A6.1b, c), including monitoring of spinal cord potentials, measurement of epidural pressure and epidural tissue blood flow, epidural spinal cord stimulation, and epidural injection of drugs [55].
