Monitoring Gastrointestinal Tract Function
Ruben J. Azocar
Laura Santos Pavia
Suresh Agarwal
Gastrointestinal system function is of paramount importance for the maintenance of the body’s homeostasis, which is not only limited to the important functions of digestion and absorption but also closely related to immune function. Monitoring the gastrointestinal tract function remains largely based on clinical exam and a few diagnostic tests. The majority of the tests that are available have been primarily used for research purposes and are not available at the bedside of the critically ill patient (Table 30.1).
This chapter examines the diagnostic modalities available, on an organ system basis, for assessing abnormalities in the critically ill patient.
Esophagus
Tests of Esophageal Motility and Lower Esophageal Sphincter Function
Impaired tubular esophageal motility is involved in the pathogenesis of gastroesophageal reflux disease (GERD) which might cause nosocomial pneumonias in the critically ill.
Esophageal manometry has been used extensively to study GERD in critically ill patients. One study, of 15 critically ill patients, demonstrated that low esophageal sphincter (LES) pressure (mean 2.2 ± 0.4 mm Hg) and poor motor response to reflux correlated with the presence of GERD. Furthermore, low LES pressures were associated with frequent reflux episodes (60% of untreated patients) and decreased esophageal motility [1].
In a more recent 24-hour manometric study, the authors demonstrated that propulsive esophageal motility is impaired in critically ill patients receiving sedation and postulated that 24-hour motility studies appear to be a valuable and feasible method to analyze and quantify esophageal motor disorders in critically ill patients [2].
Twenty-four–hour pH and impedance monitoring further elucidates the function of the LES and the amount of gastric reflux a patient is experiencing. Over a 24-hour period, the pH should not drop below 4 frequently or for a prolonged duration (6% of total time in the supine patient, 10% of total time in the upright patient).
Both barium swallow and real-time fluoroscopy yield functional and anatomic data about the esophagus and the swallowing mechanisms. Similarly, an isotope swallow, using a technetium-99 colloid and a gamma camera, may provide data regarding esophageal physiology.
Stomach
Tests of Gastric and Duodenal Motility
Delayed gastric emptying (GE) is common during critical illness. Patients receiving enteral nutrition are frequently assessed to evaluate feeding tolerance and prevent nosocomial pneumonias.
Traditionally this is done by quantification of gastric residual volumes (GRV), which despite being easy to perform, are a poor predictor of the patient’s ability to tolerate enteral nutrition. In addition, a recent article suggests that the use of residual volumes as a marker of risk for aspiration in critically ill patients has poor validity [3].
Reflectometry (RFT) of gastric contents seems to provide complementary information on the adequacy of gastric emptying [4] by differentiating gastric contents from feeding formula when measuring GRVs. This model implies the measurement of the Brix value (BV) of the gastric aspirate at several time points. The BV is the refractive index of a substance, which is the degree of deviation or refraction of a beam of light when passing obliquely through a solution [5]. Chang et al. [6] studied 36 patients receiving continuous enteral nutrition. Based on the data collected, the authors created and algorithm using BVs and GRV, which suggest values at which enteral feedings can be safety continued. RFT uses an inexpensive handheld instrument (refractometer) similar to a small telescope. A drop of the solution is placed in the viewing window and the BV is read thought the eyepiece. The use of RFT is simple, inexpensive, and quick, but it has not been compared with what is consider the gold standard, gamma scintigraphy.
Gamma scintigraphy is a quantitative method to measuring gastric motility by administering radiolabeled solid food (usually greater than 200 kcal) and measuring transit after 2 to 4 hours. The administration of liquids may not be relevant as liquids may empty from the stomach even as solid food remains behind. The feasibility of scintigraphy testing for the critically ill patient makes is difficult as it is often impractical to transport these individuals to the nuclear radiology suite for this study.
Breath tests are a novel and useful bedside technique to assess gastric emptying of both solids and liquids by using 13C- or 14C-labeled octanoic acid. The absorption of the labeled octanoic acid in the small intestine and subsequent metabolism in the liver produce 13CO2, which can be measured in the exhaled air. The delivery of the 13-octanoic acid into the duodenum is the rate-limiting step for these processes. As such, measurement of 13CO2 levels correlates with the rate of gastric emptying. Ritz et al. [7] founded that gastric emptying of a caloric-dense liquid meal is slow in 40 to 45 of unselected mechanically ventilated patients by using the 13-octanoic acid breath test. They concluded that this test is a useful bedside adjunct to measure gastric emptying in ventilated, critically ill patients.
Gastroduodenal manometry has also been used to study the effects of critical illness in gastric motor activity. Nguyen demonstrated that in critical illness in addition to impaired proximal and distal gastric motor activity, the association between the two regions was also abnormal which interferes with meal distribution and affects GE [8]. Similar data was observed by Chapman et al. who noted that in critical illness there is slower GE probably associated by fewer anterograde waves
and more retrograde waves as recorded when measuring the antroduodenal motility [9].
and more retrograde waves as recorded when measuring the antroduodenal motility [9].
Table 30.1 Tests for Monitoring Gastrointestinal Function | ||||||||||||||||||||||||||||||
---|---|---|---|---|---|---|---|---|---|---|---|---|---|---|---|---|---|---|---|---|---|---|---|---|---|---|---|---|---|---|
|
The acetaminophen absorption test may also be used to assess gastric emptying, by administering 1,000 mg of acetaminophen and measuring serum concentrations of acetaminophen over a 1-hour period to construct an area under the curve (AUC) absorption model. This AUC is then compared to a known AUC model constructed from healthy volunteers. The utility of this test may be quite variable in the critically ill patient given differences in volume of distribution, hepatic metabolism, and renal clearance [5].
Other novel methods to assess GE include the use of ultrasound and gastric impedance monitoring (GIM). Ultrasound has used different equipment and different methods to assess GE, which has not allowed standardization or validation despite its obvious benefits of availability, lack of radiation and good interobserver agreement. GIM, which measures increases in impedance as the stomach fills, and declines as it empties, seems to be a promising tool. However, the time needed to complete and the requirement of a fasting state for baseline may interfere with its use in the clinical setting.
Tests of Mucosal Permeability and Ischemia
Microcirculatory dysfunction plays an important role in the pathogenesis of the systemic inflammatory response, sepsis, and shock. Global hemodynamic measurements do not assess
oxygen delivery at the microcirculatory level. Gut ischemia at this level causes changes in permeability leading to bacterial translocation that may initiate, perpetuate, and aggravate sepsis and multisystem organ failure (MOF). Many methods have been used to study the gut microcirculation. Unfortunately, most of them have failed to be applicable in the clinical setting or have flaws in the data collected.
oxygen delivery at the microcirculatory level. Gut ischemia at this level causes changes in permeability leading to bacterial translocation that may initiate, perpetuate, and aggravate sepsis and multisystem organ failure (MOF). Many methods have been used to study the gut microcirculation. Unfortunately, most of them have failed to be applicable in the clinical setting or have flaws in the data collected.
Tonometry
Although the diagnosis of bowel ischemia may be done by a variety of different methods, gastric tonometry is the simplest, most practical, and least invasive [10]. It attempts to determine the perfusion status of the gastric mucosa by measuring the local PCO2 [11]. As perfusion to the stomach decreases, the PCO2 in the tonometer will increase. Once cellular anaerobic respiration starts, the hydrogen ions titrate with bicarbonate, with the end result of more CO2 production by mass action. By estimating the PCO2 gap (the difference between gastric mucosa and arterial CO2) the gastric perfusion can be assessed [12]. Unfortunately, the use of the technique has not gained widespread popularity despite many clinical studies that have validated gastric tonometry as a valuable and easily accessible prognostic tool [13,14]. This may be explained by the possibility of error in the determination of the PCO2 and interoperator variability [15,16]. Other pitfalls include multiple local effects, including increased gastric secretions and refluxed duodenal contents; both of which can increase CO2 measurement and lead to false PCO2 measurement, and that this technique may only represent one region of perfusion [11].
Recently, the measurement of carbon dioxide in the sublingual mucosa by sublingual capnometry has been advocated as a monitor for tissue oxygenation and as an end-point for resuscitation [17]. Studies have demonstrated a good correlation between gastric mucosal and sublingual mucosa PCO2. In addition, sublingual mucosa PCO2 seems to respond faster to therapeutic interventions [18].
Laser Doppler Flowmetry
Laser Doppler flowmetry (LDP), which estimates gastric and jejunal blood perfusion by integrating red blood cell content and velocity, correlates well with absolute blood flow. The flowmeter consists of a laser source, a fiberoptic probe, and a photodetector with a signal-processing unit. The laser conducts through the tissue by a flexible fiberoptic guide. The probe contains an optic fiber for transmission of laser light to the tissue and two fibers for collecting the reflected scattered light. The signal-processing unit consists of a photodetector and an analog circuit to analyze the frequency spectrum of the scattered light. By determining the instantaneous mean Doppler frequency and the fraction of backscattered light that is Doppler shifted, the signal-processing unit provides a continuous output proportional to the number of red blood cells moving in the measuring volume and the mean velocity of these cells. Measurements are considered satisfactory if (a) the measurement is stable for 15 seconds; (b) the measurement is free of motion artifacts; (c) pulse waves can be clearly identified; and (d) the reading is reproducible. Although LDP is relatively easy to use and it is noninvasive, it does not account for blood flow heterogeneity, a major parameter of microcirculation [19].
Near-Infrared Spectometry
Near-infrared spectrometry (NIRS) has been used to measure local tissue blood flow and oxygenation at the cellular level [20]. Local oxygen delivery and oxygen saturation can be determined by comparing the differences in the absorption spectrum of oxyhemoglobin with its deoxygenated counterpart, deoxyhemoglobin [21]. Puyana et al. [22] reported using NIRS to measure tissue pH in a model of experimental shock and showed that NIRS gut pH correlated with the pH obtained by microelectrodes. This technology has progressed to the measurement of muscle tissue oxygenation and microcirculation by measuring thenar muscle oxygenation saturation with promising results [23].
Positron Emission Tomography
Positron emission tomography may also be used to evaluate regional blood flow. Fluoromisonidazole accumulation has been used to demonstrate abdominal splanchnic perfusion and regional oxygenation of the liver in pigs; however, the lack of portability of this technique makes it difficult to use for monitoring in the intensive care unit (ICU) [14].
Microdialysis
Microdialysis measurement of mucosal lactate is a novel way to assess gut mucosal ischemia. Tenhunen et al. [24] inserted microdialysis catheters into the lumen of the jejunum, the jejunal wall, and the mesenteric artery and vein of pigs. Subsequently, the animals were subjected to nonischemic hyperlactataemia or an episode of mesenteric ischemia and reperfusion. The lactate levels from the jejunal wall and the jejunal lumen were compared. The gut wall lactate was increased in both the nonischemic and the ischemic lactataemia whereas the lactate measured from the jejunal lumen only was altered significantly during true ischemia.
Microdialysates of other substances have also been measured, including glucose and glycerol, showing that, while lactate levels increase with ischemia, intestinal wall glucose levels drop with the same stressor. Glycerol was increased, but the changes were seen later than the changes in lactate [25]. Similarly, increases in the lactate/pyruvate ratio in both intraperitoneal or intraluminal placed microdialysis catheters have correlated with hypoperfusion [26]. As glucose from the splanchnic circulation is inhibited, pyruvate accumulates in the tissue and, in the setting of inadequate oxygen delivery, is broken down to lactate. Using glycerol as a marker, Sollingard et al. [27] suggested that gut luminal microdialysis could serve as a valuable tool for surveillance not only during ischemia, but also after the ischemic insult. This group has also suggested that gut luminal lactate measured by this technique correlates well with changes in the permeability of the intestinal mucosa after ischemia [28].
The assessment of the barrier function using colon submucosal microdialysis with a radioactive tracer substance has also been reported. No data comparing these results with local tissue chemistry have been reported [29].
These data support the idea that microdialysis could be a potentially useful method to monitor gut ischemia. However, even under investigational conditions, technical difficulties were reported in up to 15% of cases by either damage to the microdialysate membrane, dislocation of the probe, or incorrect placement [30].
Orthogonal Polarization Spectrometry and Sidestream Dark Field
Recently devices able to allow the microcirculation to be visualized directly have been used clinically [31]. Orthogonal polarization spectrometry (OPS) and the sidestream dark field
provide high-contrast images of the microvasculature. Both devices are based on the principle that green light penetrates a tissue and that then green light is absorbed by red blood cells (RBCs) hemoglobin contained in superficial vessels. Therefore, capillaries and venules can be visualized if they contain RBCs. The easiest assessment method is the microvascular flow index. The image is divided into four quadrants and the flow is characterized and scored as absent (0), intermittent (1), sluggish (2), or normal (3). The values of the quadrants are then averaged. Clinical studies suggest that this is a good method to assess microcirculation in critically ill patients. Those patients with more severe alterations have a higher mortality and that if these alterations persist they may lead to MOF [32,33,34]. In most studies, the sublingual circulation has been the site chosen. An attempt to use this method for gut ischemia by assessment of the villi microvasculature per se was not successful. Likely causes include blood flow redistribution, heterogenicity of the intestine microcirculation, and suboptimal OPS imaging, which resulted in large interobserver differences in the quantification of vessel density [35].
provide high-contrast images of the microvasculature. Both devices are based on the principle that green light penetrates a tissue and that then green light is absorbed by red blood cells (RBCs) hemoglobin contained in superficial vessels. Therefore, capillaries and venules can be visualized if they contain RBCs. The easiest assessment method is the microvascular flow index. The image is divided into four quadrants and the flow is characterized and scored as absent (0), intermittent (1), sluggish (2), or normal (3). The values of the quadrants are then averaged. Clinical studies suggest that this is a good method to assess microcirculation in critically ill patients. Those patients with more severe alterations have a higher mortality and that if these alterations persist they may lead to MOF [32,33,34]. In most studies, the sublingual circulation has been the site chosen. An attempt to use this method for gut ischemia by assessment of the villi microvasculature per se was not successful. Likely causes include blood flow redistribution, heterogenicity of the intestine microcirculation, and suboptimal OPS imaging, which resulted in large interobserver differences in the quantification of vessel density [35].
Small Intestine
Tests of Intestinal Absorption
Clinically, the recognition of malabsorption in the ICU is associated with a variety of signs and symptoms. On physical exam, abdominal distention, abdominal pain, and increased flatulence may be present. Isolated carbohydrate malabsorption may result in increased gas production, which can lead to flatulence, bloating, and abdominal distention. Likewise, diarrhea may indicate a problem with absorption of nutrients, but again it is nonspecific and other potential causes should be examined. Steatorrhea may indicate pancreatic insufficiency. It is also important to elicit the past medical history since it can provide useful information in regards to primary (i.e., lactose intolerance) or secondary (i.e., chronic pancreatitis) malabsorptive problems.
Malabsorption can be detected by a variety of tests. Stool analysis may provide information regarding carbohydrate and fat malabsorption. Bacterial fermentation of malabsorbed carbohydrates may result in an acidic fecal pH. Eherer and Fordtran [36] found that when diarrhea was caused by carbohydrate malabsorption (lactulose or sorbitol), the fecal fluid pH was always less than 5.6 and usually less than 5.3. Other causes of diarrhea rarely caused fecal pH to be as low as 5.6 and never caused a pH less than 5.3.
Another measurement is of fecal osmolarity. Assuming the fecal osmolality is similar to that of the serum, the fecal osmotic gap can be calculated. A sample is taken from the stool supernatant and if the value is greater than 50 to 100 mOsm, it would suggest the presence of an unmeasured solute. Although this solute may be a malabsorbed carbohydrate, other compounds, such as sorbitol, or ions, such as sulfates, may yield similar results.
Steatorrhea is defined as the presence of at least 7 g of fat in a 24-hour stool collection [37]. Sudan II stain is a simple screen testing and it is helpful to detect those patients with mild degrees of steatorrhea (7 to 20 g per 24 hours). The gold standard is represented by quantitative fecal fat analysis [38]. Stool is collected over 2 to 3 days while the patient ingests 75 to 100 g of fat within 24 hours. Normal values are less than 7 g per day. However, this test is laborious and may not help with differentiating diagnoses.
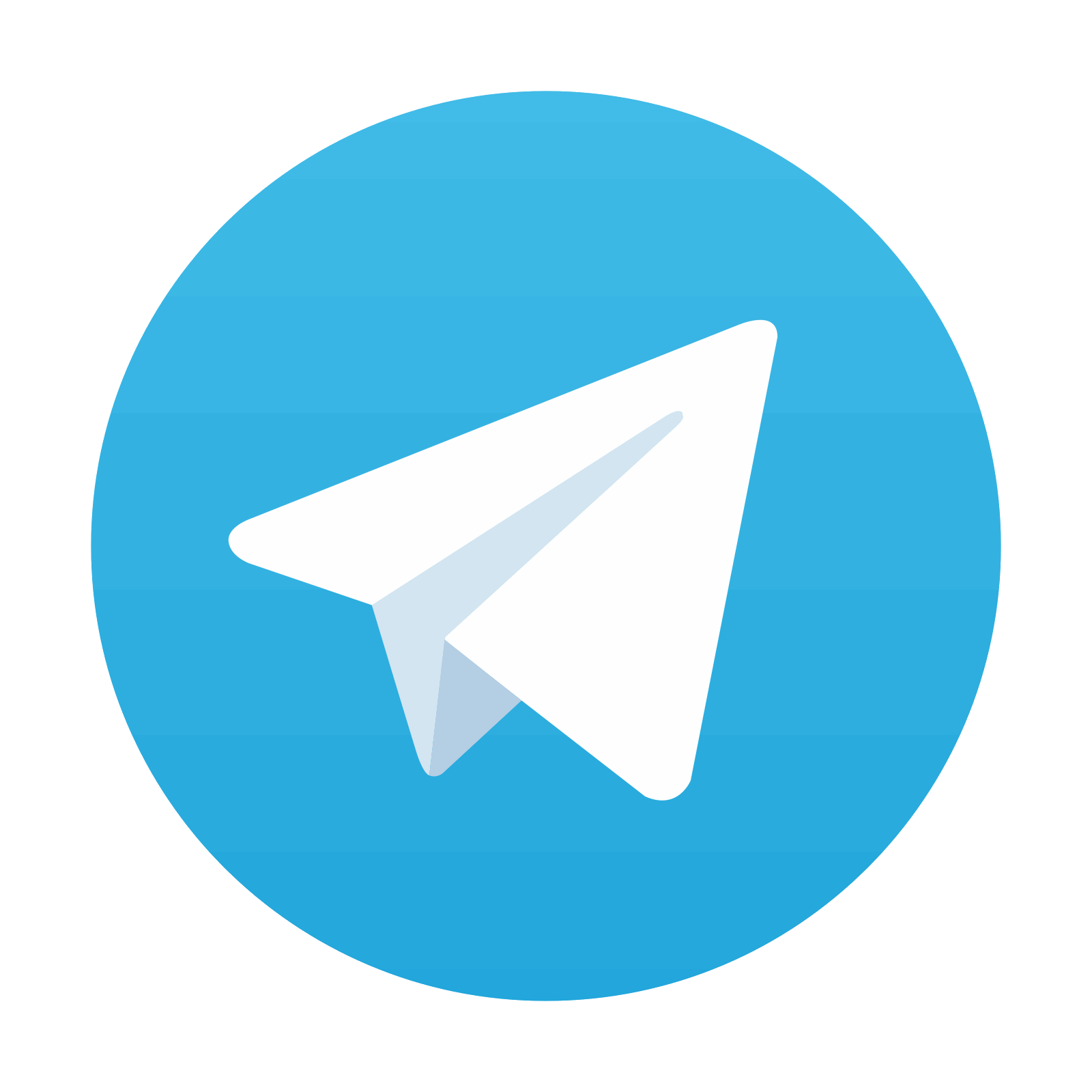
Stay updated, free articles. Join our Telegram channel

Full access? Get Clinical Tree
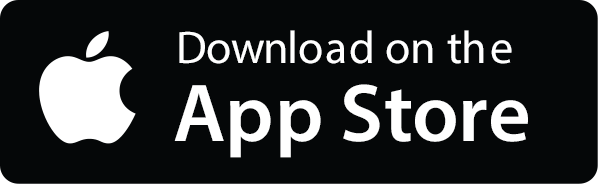
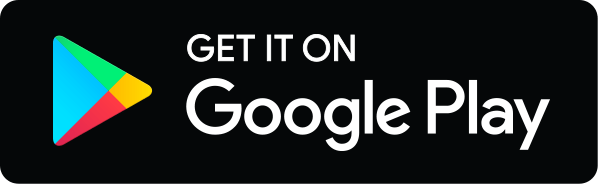