Cardiac Output
Cardiac output is the amount of blood ejected from the left ventricle in 1 minute. At rest this is approximately 5000 ml. It is determined by heart rate and stroke volume.
Heart Rate
Factors influencing heart rate include baroreceptor activity, the Bainbridge effect, pyrexia, higher centres, intracranial pressure and oxygen and carbon dioxide levels in the blood.
Stroke Volume
Stroke volume is the amount of blood ejected from the left ventricle in one contraction. At rest this is approximately 70 ml. It is affected by heart rate, myocardial contractility, preload and afterload (Fig. 5.1).
- Heart rate: tachycardia reduces diastolic filling time resulting in a decreased stroke volume.
- Myocardial contractility refers to the ability of the heart to function independently of the changes in preload and afterload (Cooke and Thompson 2011). It is commonly referred to as the ‘force of contraction’. Inotropic drugs, e.g. dobutamine and adrenaline, can increase myocardial contractility. Table 5.1 lists factors affecting myocardial contractility.
- Preload (or end-diastolic volume/pressure) is the load that passively stretches cardiac tissue before the onset of ventricular contraction (Cooke and Thompson 2011). Starling’s law of the heart states that ‘the force of myocardial contraction is directly proportional to the initial fibre length’, i.e. stretched fibres contract more forcefully (not overstretched). Venous return is the main factor determining preload and, as the filling pressure rises, stroke volume increases. However, in an overstretched ventricle, excessive dilatation may result in a fall in stroke volume. In the clinical setting manipulation of the preload is the most efficient method of improving cardiac output because it is associated with only a minimal rise in oxygen consumption. Table 5.2 lists factors affecting preload.
- Afterload is the resistance to the outflow of blood provided by the vasculature which must be overcome by the ventricles during contraction. In the clinical setting a rise in afterload, particularly in the failing heart, results in a decrease in cardiac output (Cooke and Thompson 2011). Table 5.3 lists factors affecting afterload.
Table 5.1 Factors affecting myocardial contractility
Change | Factors |
Increased contractility | Drugs with inotropic properties, e.g. dobutamine, dopamine (dose related), digoxin, adrenaline, noradrenaline; circulating catecholamines; calcium; increased preload; hyperthyroidism |
Decreased contractility | Drugs with negative inotropic properties, e.g. lignocaine, amioderone; hypoxia; hypocalcaemia and calcium channel blockers; β-adrenergic blockers, e.g. atenolol; decreased preload; functional deficit, e.g. after myocardial infarction |
Table 5.2 Factors affecting preload
Change | Factors |
Increased preload | Volume gain, e.g. fluid overload; renal failure; vasoconstriction (may be caused by drugs, e.g. noradrenaline, adrenaline and dopamine (dose related)); heart failure; hypothermia and anxiety; bradycardia |
Decreased preload | Volume loss, e.g. haemorrhage, severe vomiting and polyuria; vasodilatation, e.g. anaphylaxis, septicaemia, pyrexia, neurogenic shock and drugs such as nitrates; impeded venous return, e.g. pulmonary embolism, pericardial tamponade; tachycardia (fall in diastolic filling time) |
Table 5.3 Factors affecting afterload
Change | Factors |
Increased afterload | Drugs with vasoconstriction properties, e.g. noradrenaline; cardiogenic shock; atherosclerosis |
Decreased afterload | Drugs with vasodilator properties, e.g. nitrates, nitroprusside; anaphylaxis; septicaemia; hyperthermia |
Peripheral Resistance
Peripheral resistance is the resistance that the vessels offer to the flow of blood, i.e. dependent on the extent of dilatation or constriction (Scanlon and Sanders 2007).
The smooth muscle in the arterioles is controlled by the vasomotor centre in the medulla. It is in a state of partial contraction caused by continuous sympathetic nerve activity, often referred to as ‘sympathetic tone’. An increase in vasomotor activity causes vasoconstriction of the arterioles, resulting in a rise in peripheral resistance. If the cardiac output remains constant the blood pressure will rise. In contrast, a decrease in vasomotor activity causes vasodilatation and a fall in peripheral resistance. If the cardiac output remains constant the blood pressure will fall. The most significant factors affecting vasomotor activity are:
- Baroreceptor activity helps to maintain the blood pressure at a constant level. Baroreceptors are located in the aortic arch, carotid arteries and carotid sinus. Baroreceptor activity inhibits the action of the vasomotor centre: a rise in blood pressure increases, and a fall decreases, baroreceptor activity. When moving from a lying to a standing position, the cardiac output will fall. However, baroreceptor activity ensures that the blood pressure remains constant. After prolonged bed rest this mechanism may be lost and the patient may faint.
- Carbon dioxide (CO2): a rise in blood carbon dioxide levels increases vasomotor activity, whereas a fall suppresses it. In ventilated patients care needs to be taken to avoid over-ventilation because this may lead to a fall in carbon dioxide levels with a corresponding fall in blood pressure.
- Sensory nerves can influence vasomotor activity, particularly those that are associated with pain. Mild pain can increase vasomotor activity resulting in a rise in blood pressure, whereas severe pain may decrease vasomotor activity and cause a fall in blood pressure.
- Respiratory centre: this lies next to the vasomotor centre; an increase in its activity, particularly on inspiration, will result in an increase in vasomotor activity, causing a rise in blood pressure.
- Oxygen (O2): a moderate fall in blood oxygen levels increases vasomotor activity directly and also indirectly via chemoreceptors.
- Higher centres: emotional excitement or stress results in a rise in vasomotor activity and a corresponding rise in blood pressure. In some situations inhibition of the vasomotor centre will occur resulting in vasodilatation and a fall in blood pressure, e.g. some people faint at the sight of blood.
There are other factors that affect peripheral resistance including:
- Angiotensin: inadequate renal blood flow leads to the release of the enzyme renin which causes the formation of angiotensin, a powerful vasoconstrictor.
- Blood viscosity: if the blood viscosity increases, e.g. in polycythaemia, peripheral resistance will also rise.
- Stimulation of α- and β2-receptors (found in the smooth muscle of the arterioles): stimulation of α-receptors, e.g. by noradrenaline, will cause vasoconstriction; stimulation of β2-receptors, e.g. by salbutamol, will cause vasodilatation.
SHOCK
Shock is a clinical state with characteristic signs and symptoms but can be defined as occurring when there is an imbalance between oxygen demand and supply (McLuckie 2009), resulting in inadequate tissue perfusion (Jenkins and Johnson 2010). A complex physiological phenomenon, shock is a life-threatening condition with a variety of causes. Without treatment it leads to cell starvation, cell death, organ dysfunction, organ failure and mortality rates of >50% (Jenkins and Johnson 2010).
Haemodynamic monitoring will assist nurses in recognising the early signs of shock, facilitate timely management, evaluate treatment response and potentially reverse the early stages of this deadly sequela.
The prognosis of shock will depend on the underlying cause, severity and duration of the shocked state. The patient’s age and pre-existing illness (co-morbidity) are also contributing factors. There are four major classifications of shock: cardiogenic, hypovolaemic, distributive and obstructive (Bridges and Dukes 2005).
Hypovolaemic Shock
Hypovolaemic shock is characterised by inadequate intravascular volume (Garretson and Malberti 2007). Causes of hypovolaemia are from either internal fluid shifts or external fluid losses (Diehl-Oplinger and Kaminski 2004). Fluid shifts from intravascular compartments to ‘third spaces’ (intracellular or extracellular compartments), which do not support the circulation, can be the result of intestinal obstruction, vomiting and diarrhoea, pancreatitis, peritonitis, burns and excessive diuretic therapy (Garretson and Malberti 2007) External fluid loss can be caused by haemorrhage, severe vomiting, osmotic diuresis, trauma and surgery (Kent and Dowd 2007).
Cardiogenic Shock
Cardiogenic shock is caused by severe heart failure (Leach 2004) usually secondary to ST-elevation acute myocardial infarction (STEMI) but can also follow acute mitral regurgitation, ventricular septal rupture, cardiomyopathies, congestive cardiac failure and trauma (Garretson and Malberti 2007; Kent and Dowd 2007). As a result of a reduced cardiac output, catecholamines (adrenaline and noradrenaline), renin and aldosterone are released, which cause a tachycardia and vasoconstriction, increasing afterload, myocardial workload and oxygen consumption. Haemodynamic readings will demonstrate tachycardia, systolic BP <90 mmHg cardiac index <2.1 l/min per m2, pulmonary artery wedge pressure >18 mmHg and increased systemic vascular resistance (SVR) (Kent and Dowd 2007).
Distributive Shock
Distributive shock arises from abnormality of the peripheral circulation and can be divided into three different types: neurogenic, anaphylactic and septic (McLuckie 2009). Despite an adequate circulating volume, the vasculature expands and hypovolaemia occurs due to plasma leakage from capillaries (McLuckie 2009).
Although cardiac output could rise, the uptake of oxygen is impaired; there is still a relatively low volume because the intravascular space has increased due to dilatation of the systemic vasculature. In sepsis and anaphylaxis capillaries become permeable because of circulating inflammatory mediators. This permeability causes fluid leakage from the vasculature to the interstitial space, further reducing intravascular volume. Neurogenic shock can be caused by damage to the spinal cord or brain stem, emotional trauma or drugs that cause a reduction of sympathetic impulses causing massive vasodilatation, hypotension, bradycardia and hypothermia (Kent and Dowd 2007).
Haemodynamic readings initially appear normal or show an increase in cardiac output, fall in SVR and low to normal pulmonary artery wedge pressure (PAWP) as a hyperdynamic state develops to compensate for reducing cardiac output.
Obstructive Shock
Obstructive shock is caused by circulatory obstruction (Leach 2004). Causes include pulmonary embolism, tension pneumothorax and cardiac tamponade.
Haemodynamic readings show a fall in cardiac output, fall in PAWP and rise in SVR. Pressures in the right side of the heart, pulmonary artery and left chambers equilibrate in diastole, whereas cardiac output falls, SVR rises and PAWP is variable depending on the cause of the obstruction.
NON-INVASIVE METHODS OF HAEMODYNAMIC MONITORING
This section discusses the various non-invasive methods of haemodynamic monitoring. A non-invasive monitoring device is illustrated in Fig. 5.2.
Assessment of Respiratory Rate
Respiratory rate is an early significant indicator of cellular dysfunction. This is a sensitive physiological indicator and should be monitored and recorded regularly. Rate and depth of respirations will initially increase in response to cellular hypoxia.
Assessment of Pulse and ECG
A rapid, weak, thready pulse is a characteristic sign of hypovolaemia (Bickley and Szilagyi 2009). A full bounding or throbbing pulse may be indicative of anaemia, heart block, heart failure or the early stages of septic shock – the hyperdynamic or compensatory stage (Jenkins and Johnson 2010). A discrepancy in the volume between central and distal pulses may be caused by a fall in cardiac output (and also cold ambient temperature).
ECG monitoring is an invaluable non-invasive method of continuous monitoring of the heart rate. It can provide the practitioner with an early sign of a fall in cardiac output. The principles of ECG monitoring were discussed in Chapter 4.
Assessment of Cerebral Perfusion
Altered mental state (Cooke and Thompson 2011), such as a deterioration in conscious level, confusion, agitation and lethargy, is an important determinant of cerebral perfusion and the presence of shock.
Assessment of Skin Perfusion
Decreased skin perfusion is often characterised by cool peripheries, skin mottling, pallor, cyanosis and delayed capillary refill time (CRT). The following procedure is suggested for the assessment of CRT:
- Explain the procedure to the patient.
- Elevate the digit to the level of the heart (or slightly higher). This will ensure the assessment of arteriolar capillary and not venous stasis refill.
- Apply sufficient pressure to cause blanching to the digit for 5 seconds and then release (Resuscitation Council UK 2006).
- Time how long it takes for the colour of the skin to return to the same colour of the surrounding tissues, i.e. CRT. The normal CRT is <2 s (Gwinnutt 2008).
A sluggish (delayed) CRT (>2 s) suggests poor peripheral perfusion. Other factors that can prolong CRT include a cold ambient temperature, poor lighting and old age (Resuscitation Council UK 2006). CRT as an assessment tool must be used with caution because it is not a specific monitoring aid and used only as a guide.
Assessment of Urine Output
Urine output can indirectly provide an indication of cardiac output. In health, 25% of the cardiac output perfuses the kidneys. When renal perfusion is adequate, urine output should exceed 0.5 ml/kg per hour (Snyder 2009). Decreased urine output may be an early sign of hypovolaemia because, when cardiac outputfalls, so does renal perfusion (Gonce Morton 2009). Once urine output is less than approximately 500 ml/day, the kidneys are unable to excrete the waste products of metabolism; uraemia, metabolic acidosis and hyperkalaemia will then develop (Gwinnutt 2008).
In the critically ill patient, acute renal failure is usually caused by inadequate renal perfusion pressure (prerenal failure) caused by, for example, hypovolaemia (Gwinnutt 2008). If diuretics have been administered, e.g. frusemide urine output is not helpful in assessing cardiac output. If the patient is catheterised, ensure that the tube is not blocked or kinked.
Arterial Blood Pressure Measurements
Arterial blood pressure (ABP) is the force exerted by the circulating volume of blood on the walls of the arteries (Wallymahmed 2008). Changes in cardiac output or peripheral resistance can affect the blood pressure. A patient with a low cardiac output can maintain a normal blood pressure by vasoconstriction, whereas a patient who is vasodilated may be hypotensive despite a high cardiac output, e.g. in sepsis. Mean arterial pressure (MAP) is an average pressure reading within the arterial system (Garretson 2005) and is a useful indicator because it can approximate perfusion to essential organs such as the kidneys. MAP is recognised as the main therapeutic endpoint for a patient with sepsis (Giuliano 2006). MAP is also thought to be more relevant because it is least dependent upon measurement site or technique and least altered by dampening, and determines tissue flow by autoregulation (Sturgess and Morgan 2009).
‘The adequacy of blood pressure in an individual patient must always be assessed in relation to their premorbid value’ (Hinds and Watson 2008). Table 5.4 provides an indication of ‘expected’ systolic and diastolic blood pressure measurements. Hypotension can lead to inadequate perfusion of vital organs. Hypertension increases myocardial workload and can precipitate cerebral vascular accidents.
Table 5.4 Normal intracardiac pressures
Reproduced with kind permission of Routledge from Woodrow (2000, p. 212).
Parameter | Normal range |
Central venous | 0 to +8 mmHg (right atrial level) |
Right ventricle | 0 to +8 mmHg diastolic |
+15 to +30 mmHg systolic | |
Pulmonary capillary wedge pressure | +5 to +15 mmHg |
Left atrium | +4 to +12 mmHg |
Left ventricle | +4 to +12 mmHg diastolic |
+90 to +140 mmHg systolic | |
Aorta | +90 to +140 mmHg systolic |
+60 to +90 mmHg diastolic | |
+70 to +105 mmHg mean |
Cardiac output is related to pulse pressure, which is the difference between the systolic and diastolic pressures, usually 40–60 mmHg (Gonce Morton 2009). Following a fall in cardiac output the pulse pressure will narrow, resulting in a thready pulse. In the early stages of septic shock, the cardiac output can rise, resulting in a wide pulse pressure and bounding pulses.
Factors Influencing Blood Pressure Measurements
There are numerous factors that can influence blood pressure, e.g. nicotine, anxiety, pain, position of patient, medications, illicit drugs, alcohol and exercise. It is important to ensure that a standardised approach is used to minimise the impact of extraneous variables on blood pressure (Wallymahmed 2008).
There are no recommendations of which arm to use but initially the BP should be taken in both arms (Heart Foundation 2008). Wide discrepancies between right and left arm blood pressure measurements may be indicative of an aortic aneurysm.
Factors Affecting Accuracy of Blood Pressure Measurements
The accuracy of blood pressure measurement may be affected by the following factors:
- Cuff width: if this is too narrow the blood pressure reading will be falsely high whereas if it is too wide it will be falsely low (British Hypertension Society 2006). The European Standard recommends that the width of the bladder should be 40%, and the length 80–100%, of the limb circumference (British Hypertension Society 2006).
- Position of the arm: the arm should be supported in a horizontal position at the level of the heart. Incorrect positioning during the procedure can lead to errors of as much as 10% (Heart Foundation 2008).
- Deflating the cuff too quickly: cuff should be deflated at 2–3 mm/beat (Heart Foundation 2008).
Complications
Complications associated with non-invasive blood pressure devices include limb oedema, friction blisters and ulnar nerve palsy if the cuff is placed too low on the upper arm (British Hypertension Society 2006). If the patient is being heparinised or has a systemic coagulopathy, over-inflation or frequent inflations could cause excessive bruising.
GENERAL PRINCIPLES OF MONITORING WITH TRANSDUCERS
Transducers enable the pressure readings from invasive monitoring of the patient to be displayed on a monitor, i.e. arterial lines or central venous pressure (CVP) lines. To maintain patency of the cannula and tubing and prevent back-flow of blood, a bag of (0.9%) saline should be connected to the transducer tubing and kept under continuous pressure of 300 mmHg (i.e. greater than arterial pressure), thus facilitating a continuous flush at 3 ml/h.
Best Practice – Monitoring with Transducers
Check flush bag each shift – if it runs too low the line will clot off
Ensure that the pressure bag remains inflated at 300 mmHg – to ensure continuous flushing
If flat trace check for breaks in the circuit and air – rectify safely and flush line
If trace remains flat withdraw blood while manipulating limb
Always check the patient – asystole causes a flat trace
Ensuring Accuracy
The following precautions will help to ensure accuracy of measurements:
- Keep the transducer level with the zero reference point, usually the midaxilla. Always use the same reference point in order to ensure meaningful comparison.
- Limit the use of three-way taps.
- Remove any air bubbles from the system.
- Calibrate the transducer to atmospheric pressure prior to and regularly during use. This should be undertaken following the manufacturer’s recommendations and is typically as follows:
1. Switch the three-way tap in the tubing open to air (atmospheric pressure) and off to the patient.
2. Press the zero button on the monitor and observe for 0 to be displayed.
3. Switch the three-way tap off to air and open to the patient.
4. Ensure that the transducer is at the zero reference point and observe for the pressure trace on the monitor.
Principles of Arterial Pressure Monitoring
Indications for insertion of an arterial line include the requirement for continuous monitoring of arterial blood pressure in critically ill patients (Garretson 2005), when using potent vasoactive drugs such as adrenaline and noradrenaline, and frequent blood sampling, e.g. blood gas and acid–base analysis.
Best Practice – Monitoring an Arterial Line
Ensure arterial line is clearly labelled ‘arterial’
The transducer should be level with the phlebostatic axis (bisection of the fourth intercostal space at midpoint between anterior and posterior chest wall)
Limb should be exposed and constantly observed for signs of a decrease in perfusion and disconnection of the cannula (Garretson 2005)
Use transparent dressing so site can be monitored for signs of infection
Ensure that monitor alarms are set following local protocols
If flat trace observed, once asystole excluded, identify and rectify cause of problem
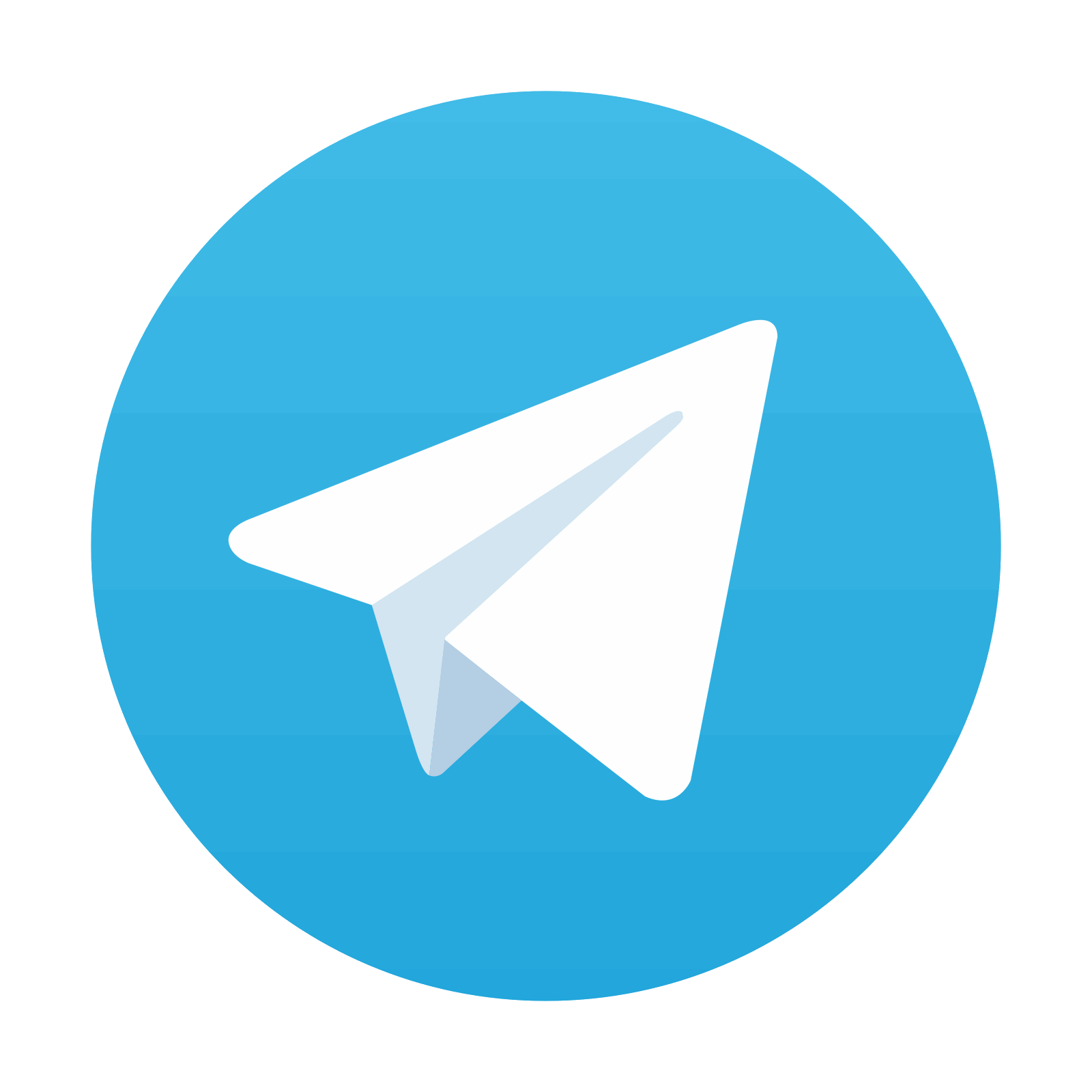
Stay updated, free articles. Join our Telegram channel

Full access? Get Clinical Tree
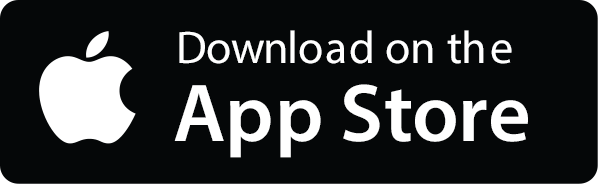
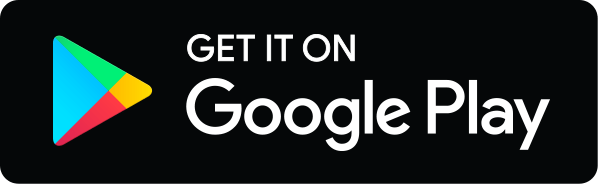