Modification of Metabolic Responses to Surgery by Neural Blockade
Francesco Carli
Thomas Schricker
Catabolic Changes Induced by Surgical Stress
The endocrine, metabolic, and inflammatory response to surgery make up the constellation of physiologic changes termed the catabolic response to surgery. Accumulated evidence suggests that this phenomenon, if untreated, is accompanied by undesirable effects leading to morbidity and mortality. Pharmacologic, nutritional, and physical interventions have been used to prevent or attenuate catabolic illness. Regional anesthesia and, in particular, neuraxial blockade has been found to modulate some aspects, with potential implication for the anesthetic practice. Nevertheless, the realization that the pathophysiology of surgical stress is multifactorial requires a global perspective in the use of interventional strategies.
Although previous publications have covered in great detail all various aspects of the neuroendocrine and inflammatory response to surgery and the changes produced by regional anesthesia, the purpose of this chapter is to focus on clinically relevant alterations, with emphasis on the effectiveness of some of the most common regional anesthesia procedures.
Metabolic and Functional Consequences of Surgery
Hyperglycemia
The human body’s circulating concentration of glucose, contrary to other metabolic substrates such as ketone bodies, fatty acids, or amino acids, is controlled within a narrow range (Fig. 6-1). Metabolically healthy subjects maintain glycemia between 3.6 and 7.8 mmol/L irrespective of their physiologic state (fasting, feeding, exercise). Blood glucose levels increase during periods of stress, including sepsis, injury, and surgery (1).
The degree of intraoperative hyperglycemia depends on the type, severity, and extent of surgical tissue trauma (Fig. 6-2). In fasting patients undergoing elective intraperitoneal procedures, blood glucose levels typically increase to values between 7 and 10 mmol/L (2). During cardiac surgery the disturbance of glucose homeostasis is impressive, with blood glucose values frequently exceeding 15 mmol/L in nondiabetic (3,4) and 20 mmol/L in diabetic subjects (5).
The occurrence of hyperglycemia is related to stereotypical metabolic and endocrine alterations induced by the surgical insult: stimulated glucose production (6), decreased glucose utilization (7), enhanced renal absorption of filtered glucose (8), inadequate insulin secretion, and decreased insulin activity (9,10). Although hyperglycemia per se is usually restricted to the immediate perioperative period, metabolic derangements are severe enough to produce insulin resistance up to 2 weeks after abdominal surgery (9,10).
Glucose is toxic under certain conditions such as surgical stress, which triggers the release of mediators. These mediators serve two roles: on one hand, they inhibit the expression of the insulin-dependent membrane glucose transporter glut-4, which is mainly located in the myocardium and the skeletal muscle (Fig. 6-3). On the other hand, they stimulate the expression of the insulin-independent membrane glucose transporters glut-1, -2, and -3, which are located in the brain, endothelium, liver, and some blood cells. Although insulin-dependent cells are protected by insulin resistance, most of the circulating glucose enters cells that do not require insulin for uptake, resulting in a cellular glucose overload: once inside the cell, glucose either nonenzymatically glycosylates proteins such as immunoglobins and renders them dysfunctional or enters glycolysis and oxidative phosphorylation. That pathway generates excess superoxide molecules that bind to nitric oxide (NO), lead to the formation of peroxynitrate, and ultimately result in mitochondrial dysfunction and cell death (Fig. 6-3) (13).
Evidence has mounted that even moderate increases in blood glucose are associated with poor outcome. Patients with fasting glucose levels of over 7 mmol/L or random blood glucose levels of more than 11.1 mmol/L on general surgical wards showed an 18-fold increased in-hospital mortality, a longer length of stay, and a greater risk of infection (11). In a heterogenous group of critically ill patients, mortality was directly correlated with increasing glucose levels above 5 mmol/L (12). The lowest hospital mortality occurred in patients with a mean blood glucose of 4.5 to 5.5 mmol/L. Patients with cardiovascular disease appear to be particularly sensitive to changes in glycemia.
Overall studies in both the basic and clinical sciences are compelling in demonstrating that acute hyperglycemia is detrimental to patient outcome.
Loss of Protein
The cumulative net nitrogen losses after elective abdominal operations range between 40 and 80 g of nitrogen; complications that delay the use of the gastrointestinal tract may result in nitrogen losses of up to 150 g (Fig. 6-4) (14). Patients suffering from multiple injury and septic shock lose more than 200 g of nitrogen, whereas nitrogen losses after severe burns can exceed 300 g. The principal underlying defect appears to be an accelerated rate of protein breakdown and amino acid oxidation, along with an insufficient increase in protein synthesis (15,16,17). Endogenous amino acid oxidation and amino acid release from the muscle after abdominal surgery have been shown to increase by 90% and 30%, respectively, whereas whole body protein synthesis increases by 10% only (17). The magnitude of this alteration is substantial considering the fact that muscle tissue represents approximately 45% of body weight and contributes as much as 20% to total body protein synthesis. The clinical importance of this catabolic pattern can be appreciated more readily when one remembers that 1 g of nitrogen is the equivalent of 30 g of hydrated lean tissue. Therefore, a loss of 50 g of nitrogen, as seen after uncomplicated cholecystectomy, would be the equivalent of 1,500 g of lean tissue. The latter point is of utmost clinical relevance, as the length of time for return of normal physiologic function after discharge from the hospital is related to the extent of loss of lean body mass during hospitalization (18). Because protein represents both structural and functional body components, erosion of lean tissue also may lead to devastating consequences such as delayed wound healing (19), compromised immune function, and diminished muscle strength that result in prolonged convalescence and increased morbidity (20,21).
Lipotoxicity
Free fatty acid (FFA) levels during and after surgery are increased as a result of prolonged preoperative fasting, sympathoadrenergic responses to surgical injury, and the lipolytic
action of heparin administered for the prevention of thrombosis (22). High levels of FFA have been shown to depress myocardial contractility (23), inhibit glycolytic flux, and increase myocardial oxygen consumption without a concomitant increase in myocardial work (24,25). Furthermore, fatty acids may impair calcium homeostasis and increase the production of free radicals, leading to electrical instability and ventricular arrhythmias. Elevated fatty acids also have been found to cause endothelial dysfunction and impair endothelium-dependent vasodilatation through the inhibition of endothelial nitric oxide synthase (eNOS) (26).
action of heparin administered for the prevention of thrombosis (22). High levels of FFA have been shown to depress myocardial contractility (23), inhibit glycolytic flux, and increase myocardial oxygen consumption without a concomitant increase in myocardial work (24,25). Furthermore, fatty acids may impair calcium homeostasis and increase the production of free radicals, leading to electrical instability and ventricular arrhythmias. Elevated fatty acids also have been found to cause endothelial dysfunction and impair endothelium-dependent vasodilatation through the inhibition of endothelial nitric oxide synthase (eNOS) (26).
Table 6-1 Hormonal effects on protein loss and blood glucose | ||||||||||||||||||||||||
---|---|---|---|---|---|---|---|---|---|---|---|---|---|---|---|---|---|---|---|---|---|---|---|---|
|
Functional Impairment
Muscle fatigue is characterized by a decreased ability to carry out activities of daily living together with an element of depression and muscle weakness (Table 6-2) (27). After major surgery, fatigue and tiredness can last for 4 to 6 weeks. Although the mechanism for muscle weakness has not been elucidated, it appears to be a combination of impaired nutritional intake, the inflammatory-metabolic response, immobilization, and a subjective feeling of fatigue (28). A decrease in handgrip strength has been found to be related to the magnitude of surgical stimulus, and can last up to 3 to 4 weeks. A decrease in vital capacity has also been reported to be particularly relevant after upper abdominal surgery (29,30). Assessment of energy expenditure after surgery shows a marked decrease in adaptability to exercise manifested as increased heart rate and circulating levels of lactate (Table 6-1).
Table 6-2 Functional changes after surgery | ||||||||||||||||
---|---|---|---|---|---|---|---|---|---|---|---|---|---|---|---|---|
|
Catabolic Response to Acute Pain
Severe acute surgical pain results in an abnormal response characterized by sympathetic over-reactivity and increased heart rate, systemic vascular resistance, cardiac output, and coronary vasoconstriction. An isolated painful stimulus response cannot be entirely separated from that associated with surgical injury, and attempts have been made to identify the neuroendocrine and metabolic changes associated with experimental pain in the absence of surgery. Following electrical stimulation of the abdominal wall, a painful response (visual analogue scale 8 out of 10) elicits a stress response with significant increase in cortisol, catecholamines, and glucagon, and a decrease in insulin sensitivity and glucose uptake (31). This response continues for a certain period of time that is directly related to the time and extent of injury. As it is difficult to dissociate the inflammatory component from the nociceptive stimulus, it is assumed that endocrine and humoral responses are directly integrated.
Although these metabolic changes occur after surgery in all patients undergoing major surgery and experiencing pain, there are certain patient populations in whom the catabolic response is exaggerated.
Metabolically Challenged Patients
Diabetes Mellitus
Depending on the type of the procedure, the prevalence of type 2 diabetes in surgical patients ranges from 12% to 28% (Fig. 6-5) (32). Type 2 diabetic patients experience a higher mortality and morbidity in response to surgical treatment and have a more prolonged convalescence than those who are nondiabetic (33). Insulin resistance is associated with catabolic changes in protein and glucose metabolism (34,35). Poorly controlled type 2 diabetic subjects show increased protein loss (36), although protein homeostasis in the presence of good glucose control appears to be preserved (Figs. 6-6 and 6-7) (37).
Because the metabolic and endocrine alterations observed in patients with type 2 diabetes are similar to those induced by surgical tissue trauma per se (“diabetes of the injury”), it was
previously held that the metabolic abnormalities after surgery are accentuated in diabetic patients (38,39). Recently, evidence was provided that the catabolic response to colorectal surgery is indeed increased in patients with type 2 diabetes mellitus as reflected by a 50% greater protein loss, glucose production, and glucose plasma concentration (40). Feeding is poorly tolerated by the diabetic patient, because it is followed by an exaggerated hyperglycemic response (Table 6-3).
previously held that the metabolic abnormalities after surgery are accentuated in diabetic patients (38,39). Recently, evidence was provided that the catabolic response to colorectal surgery is indeed increased in patients with type 2 diabetes mellitus as reflected by a 50% greater protein loss, glucose production, and glucose plasma concentration (40). Feeding is poorly tolerated by the diabetic patient, because it is followed by an exaggerated hyperglycemic response (Table 6-3).
Cancer
Malnutrition and depletion of lean body mass are characteristic of patients with cancer. Many clinical and biochemical indices have been used to characterize the nutritional status of surgical patients, but all techniques have limitations (41); anthropometric and body composition measurements must be treated with caution in subjects who are dehydrated and who have edema or ascites (42). Serum proteins are pathophysiologic markers and not specific for the nutritional state (43), because they are affected by influences other than malnutrition or catabolism, including inflammation with redistribution and dilution.
![]() Figure 6-6. Circulating levels of blood glucose in diabetic and nondiabetic patients with surgical and medical pathologies. |
Quantitative assessment of preoperative protein catabolism in surgical patients becomes relevant because the frequency of extreme forms of undernutrition seems to decline. Contrary to earlier studies reporting incidences of up to 40% on hospital admission (44), more recent reports indicate that severe malnutrition occurs in only 6% to 20% of hospitalized patients (45). Less than 5% of patients undergoing surgery for colorectal cancer are malnourished. The development of malnutrition represents a systemic response to the tumor. Anorexia and reduced intake, rather than changes in energy expenditure, result in negative energy balance (46).
Evidence suggests a link between the occurrence of weight loss and alterations in whole body protein catabolism. Protein loss in well-nourished, weight-stable cancer patients was
normal, whereas it was significantly increased in malnourished, weight-losing subjects suffering from gastrointestinal malignancy (47). These results confirm earlier observations that, in cancer cachexia, a maladaptation to the starved state occurs, with a continued mobilization of protein and calorie reserves in the face of a decreased intake (48).
normal, whereas it was significantly increased in malnourished, weight-losing subjects suffering from gastrointestinal malignancy (47). These results confirm earlier observations that, in cancer cachexia, a maladaptation to the starved state occurs, with a continued mobilization of protein and calorie reserves in the face of a decreased intake (48).
Table 6-3 Blood glucose and protein loss during fasted and IV infusion of dextrose | ||||||||||||||||||||||||
---|---|---|---|---|---|---|---|---|---|---|---|---|---|---|---|---|---|---|---|---|---|---|---|---|
|
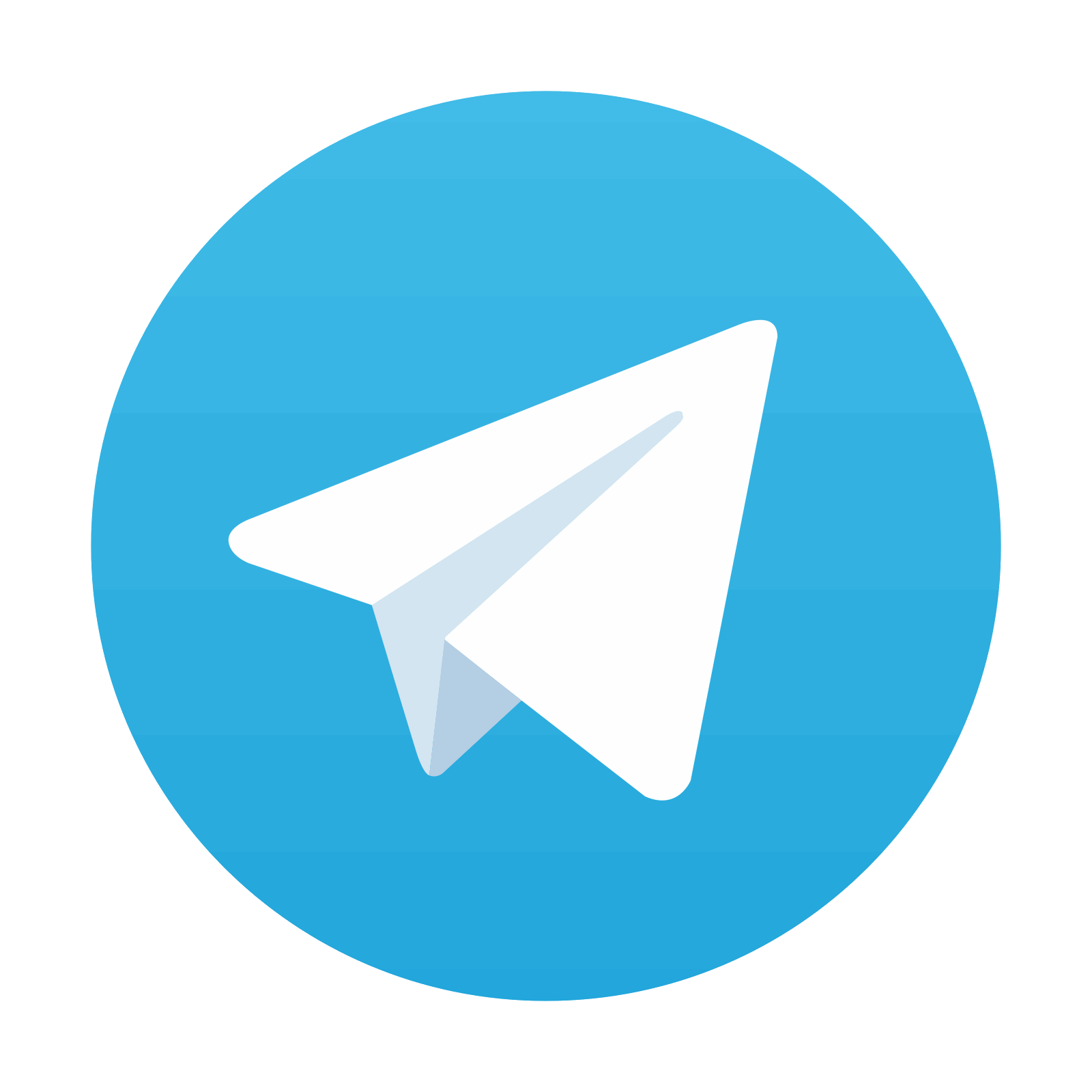
Stay updated, free articles. Join our Telegram channel

Full access? Get Clinical Tree
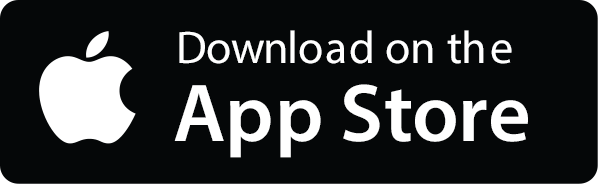
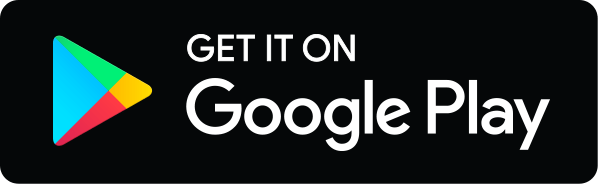