Multimodal medical management of cancer- and non–cancer-related pain can involve the use of nonopioid medications, as well as opioid medications. Nonopioid medications, often referred to as adjuvant medications, can take the form of amine reuptake inhibitors, such as the tricyclic antidepressants (TCAs); neuronal membrane stabilizers, such as the sodium and calcium channel–blocking anticonvulsants; nonsteroidal anti-inflammatory drugs (NSAIDs); topical analgesics; and muscle relaxants. The neuronal membrane stabilizers and data associated with their use for primarily neuropathic pain (NP) are discussed in this chapter.
Based on extensive studies, NP appears to result from a cascade of multiple mechanisms following tissue injury. In broad terms, neural plasticity following nerve injury may result in peripheral nerve fibers communicating abnormal input to the central nervous system (CNS), which in turn may remodel CNS signaling. NP has a heterogeneous origin and may involve both the central and peripheral neural pathways following injury. Although neuroplasticity may help facilitate recovery, unfortunately, this is not always the case, and in fact, the downstream impact of the neuromodulatory changes associated with neuroplasticity, including abnormal stimulation or decreased inhibitory activity, may result in enhanced pain processing. Chronic pain develops when this neural stimulation continues beyond the range of expected healing and the triggering stimulus is no longer present. Moreover, ion channel functioning is affected as well by changes in signaling. Sodium and calcium channels play a fundamental role in the propagation of hyperexcitability in central and peripheral neurons. Accumulation of excessive or misplaced ion channels can lead to ectopic, spontaneous firing of sensory nerves and dorsal root ganglion cell bodies and result in hyperexcitability of the primary afferents and pain.
Research into the physiologic source and pharmacologic management of NP led to a focus on sodium and calcium channel blockade. Clinically available agents that act on these ion channels include the membrane-stabilizing agents typically used for the treatment of epilepsy. Many of these agents have been tried with varying success in patients with pain. Multiple classes of medications that fall under the membrane stabilizer classification are beneficial in the treatment of pain ( Table 39.1 ). These agents include the antiepileptic/anticonvulsants, local anesthetics, TCAs, and antiarrhythmic medications. As a group, they inhibit the development and propagation of ectopic discharges. The primary agents used for NP include antiepileptic/anticonvulsants, local anesthetics, and the TCAs, which are discussed in a separate chapter. Gabapentin and pregabalin, also anticonvulsants, are discussed separately under calcium channel modulators because their mechanism of action differs from that of the other agents typically used for epilepsy.
Membrane Stabilizer | Mechanism | Side Effects |
---|---|---|
Carbamazepine | Na channel blockade | Sedation, dizziness, gait abnormalities, hematologic changes |
Oxcarbazepine | Na channel blockade | Hyponatremia, somnolence, dizziness |
Lamotrigine | Stabilizes slow Na channel; suppress release of glutamate from presynaptic neurons | Rash, dizziness, somnolence |
Gabapentin/pregabalin | Binds to α 2 δ subunit of voltage-gated Ca channel | Dizziness, sedation |
Valproic acid | Na channel blockade; increases GABA | Somnolence, dizziness, gastrointestinal upset |
Topiramate | Na channel blockade; potentiates GABA inhibition | Sedation, kidney stones, glaucoma |
Mexiletine | Na channel blockade | Nausea, blurred vision |
Lacosamide | Na channel blockade | Dizziness, nausea, double vision, headache |
The effectiveness of medications for pain relief is often determined by using one of several standard outcome measures, each of which assesses the change in average daily pain intensity score on a 10-cm (100-mm) visual analog scale (VAS) or a 11-point Likert (0, no pain; 10, worst possible pain) numeric rating scale (NRS). Patients who report pain relief of 30% or greater (moderate benefit) are considered to have experienced a clinically meaningful result; patients who report pain relief of 50% or greater are considered to have experienced substantial benefit. An often-used tool to allow comparison between different drugs and diseases and more precisely judge the efficacy of an agent is the number needed to treat (NNT). The NNT is the number of patients needed to be treated with a particular drug to obtain one patient with a defined degree of relief. Usually, the parameter “NNT for greater than 50% pain relief” is used because it is easily understood and seems to be related to relevant clinical effect. The number needed to harm (NNH) is the number needed to treat with a certain drug before a patient experiences a significant side effect. The NNH of several drugs used for pain management is not yet known. Drugs with a low NNT/NNH ratio are superior to the drugs with a high NNT/NNH ratio.
Calcium Channel Modulators
The first-line treatment agents recommended for NP include the calcium channel modulators. The intracellular free calcium ion concentration is only 1 in 10,000 that of the extracellular environment, and influx of calcium through calcium channels has important depolarizing effects on neurons. Voltage-gated calcium channels can be divided into high-voltage–activated (HVA) and low-voltage–activated (LVA) channels. Electrophysiologic characteristics allow division into HVA and LVA channels, depending on the threshold of activation. The HVA group is further divided into types L, P/Q, N, and R. These groups require large membrane depolarization and are mainly responsible for entry of calcium and release of neurotransmitter from presynaptic nerve terminals. Low-voltage channels, such as the T type, regulate firing by participating in bursting and intrinsic oscillations. The spike and wave discharges from the thalamus with absence seizures are dependent on T-type calcium channels; these discharges are inhibited by valproic acid or ethosuximide. The N-type HVA calcium channels are thought to be largely responsible for release of neurotransmitter at synaptic junctions and become inactivated rather quickly. The P/Q-type calcium channel is so named because it was first described in the Purkinje cells of the cerebellum. The T-type channel, named after the transient currents elicited, starts to open with weak depolarization, near resting potential. L-type channels are found in high concentration in skeletal muscle and in many other tissues, such as neuronal and smooth muscle, where it has been most studied. The voltage-gated calcium channel is composed of five polypeptide subunits and is the target of many drugs. Calcium channels consist of an α protein, along with several auxiliary subunits; the α protein forms the channel pore.
Gabapentin (Neurontin, Gralise, Horizant)
The calcium channel modulators that are used for the treatment of NP, such as gabapentin and pregabalin, bind to the α 2 δ subunit of L-type voltage-gated calcium channels, and such binding results in decreased release of glutamate, norepinephrine, and substance P. Though structurally derived from the inhibitory neurotransmitter γ-aminobutyric acid (GABA), neither gabapentin nor pregabalin bind to or have activity at the GABA receptor. They also have no effect on the uptake or metabolism of GABA.
The standard initial dose of gabapentin is dependent on the particular gabapentin formulation used. For the first available preparation of gabapentin (Neurontin), it is 100 to 300 mg daily. Although the U.S. Food and Drug Administration (FDA)-approved dose of this preparation for the treatment of post-herpetic neuralgia (PHN) (the only painful condition for which this preparation is indicated) is 1800 mg, many clinicians will proceed with a gradual increase to a maximum of 3600 mg/day administered in three divided doses ( Table 39.2 ). To minimize the consequence of certain adverse effects such as sedation and dizziness, the initial dose is often given at bedtime. After 2 to 5 days, the dose is increased to 300 mg twice daily and, after another 2 to 5 days, to 300 mg three times daily thereafter. Subsequently, the dose can be increased by 300 to 600 mg every other week as tolerated until an effective dosage is obtained or the maximum daily dose is reached. The main dose-limiting side effects are fatigue, somnolence, and dizziness, which are often attenuated by gradual dose titration. Although gabapentin has few drug interactions, a reduced dosage is necessary in patients with renal insufficiency. However, starting dosages of gabapentin often do not provide immediate pain relief, and the slow titration requirements may result in adequate pain relief taking up to 2 months to achieve when given as immediate-release gabapentin, although when given as the extended-release formulation, therapeutic doses can be reached in approximately 2 weeks.
Membrane Stabilizer | Initial Dosage | Titration | Max Therapeutic Dosage |
---|---|---|---|
Carbamazepine | 100-200 mg BID | Increase by 200-mg increments gradually | 1200 mg QD |
Oxcarbazepine | 600 mg daily BID | Increase by 300 mg | 1200-1800 mg TID |
Lamotrigine | 25-50 mg QHS | Increase by 50 mg every 1-2 wk | 300-500 mg QD |
Gabapentin ∗ | 100-300 mg QHS | Increase by 100-300 mg or 100-300 mg TID every 1-7 days as tolerated | 3600 mg (1200 TID) |
Gabapentin GR | 300 mg QHS | Day 1, 300 mg; day 2, 600; days 3-6, 900; days 7-10, 1200; days 11-14, 1500, then 1800 | 1800 mg QHS |
Pregabalin ∗ | 50 mg TID or 75 mg BID | Increase to 300 mg daily after 3-7 days, then by 150 mg/day every 3-7 days as tolerated | 600 mg QD (200 mg TID or 300 mg BID) |
Valproic acid | 250 mg BID | Increase by 250 mg weekly | 500 mg BID |
Topiramate | 50 mg QHS | Start at 50 mg BID after 1 wk, then increase 100 mg BID after 7 days | 100 mg BID |
Mexiletine | 150 mg QD | Increase to 300 mg in 3 days and then 600 mg | Maximum: 10 mg/kg daily |
Gabapentin has many uses in patients with multiple pain conditions. Studies have been performed on patients being treated for PHN, complex regional pain syndrome (CRPS), painful diabetic neuropathy (PDN), and other forms of NP, as well as for pain of controversial etiology, including opioid-induced hyperalgesia. In one study, patients with PHN being treated with opioids, TCAs, or both were identified and divided into two groups: 113 receiving gabapentin and 116 receiving placebo, in addition to their current baseline pain treatment regimen. For a period of 8 weeks patients were maintained on their respective therapies, with a 4-week titration of gabapentin to a maximum dose of 3600 mg/day. The results indicated that the patients who received gabapentin had a decrease of nearly 2 points in their VAS score for pain, as opposed to a decrease of just 0.5 in the placebo-treated patients ( P < 0.001). Along with a decrease in pain, patients also reported improvement in their 36-Item Short-Form Health Survey (SF-36) quality-of-life scores and noted improved functionality and feeling better with more restful sleep at night.
The analgesic effect of gabapentin in patients with PDN has also been evaluated. A randomized, double-blind, placebo-controlled (RCT) multicenter trial demonstrated a decrease of 2.5 in the VAS score in patients receiving gabapentin, up to 3600 mg/day, versus a decrease of 1.4 in patients in the control group ( P < 0.001). As with the PHN study, patients also had a favorable change in their SF-36 scores, with more restful sleep at night and overall improvement in functioning.
Gabapentin has also been studied in patients with lumbar spinal stenosis. In a pilot study, both patient groups received “standard care,” including physical therapy, lumbosacral bracing, and NSAIDs. The treatment group also received gabapentin, 900 to 2400 mg, administered in three divided doses. After 4 months, patients who received gabapentin reported improvement in pain scores, increased walking distance, and decreased sensory and motor deficits. These results suggest that in the appropriate setting, gabapentin may provide symptomatic benefit for certain patients with lumbar spinal stenosis. In a double-blind, randomized, placebo-controlled 8-week trial, patients enrolled included those with CRPS, PHN, radiculopathy, post-laminectomy syndrome, post-stroke syndrome, phantom limb pain, and other NP syndromes. Gabapentin was initially started at 900 mg/day for 3 days and then increased to a maximum of 2400 mg/day at the end of week 5. The conclusion of the study showed that gabapentin reduced pain and improved certain quality-of-life measures in these patients. Gabapentin has also been found to be effective in reducing the pain associated with multiple sclerosis, specifically, the paroxysmal pain with a throbbing, pricking, and cramping quality rather than the dull, aching pain experienced by patients with multiple sclerosis. Of interest is that gabapentin appears to improve the analgesic efficacy of opioids in patients with NP.
In studies of gabapentin for postamputation pain and phantom limb pain, it was found to be less effective than for other NP states. Nikolajsen and colleagues administered gabapentin to patients following limb amputation and found no effect on postamputation or phantom limb pain. In a small cohort-control study, gabapentin was found to be effective for the treatment of chemotherapy-induced, painful peripheral neuropathy. However, an earlier, larger RCT found no benefit with gabapentin therapy for the same condition.
Combination therapies for NP have also proved successful. In a crossover RCT, patients received daily active placebo, sustained-release morphine, gabapentin, or a combination of gabapentin and morphine for 5 weeks. Fifty-seven patients underwent randomization (35 with PDN and 22 with PHN) and 41 completed the trial. Mean daily pain at the maximally tolerated dose of the study drug was reduced from 5.72 at baseline to 4.49 with placebo, 4.15 with gabapentin, 3.70 with morphine, and 3.06 with the gabapentin-morphine combination ( P < 0.05 for the combination vs. placebo, gabapentin, and morphine). In an extremely important and well-performed trial, combination therapy with gabapentin and the TCA nortriptyline was found to be highly effective in the treatment of NP resulting from diabetes and varicella zoster. Although this study was not designed to examine the manner of interaction (e.g., drug-drug synergism vs. simple additivity), the results are highly suggestive of a synergistic analgesic effect. Patients achieved greater pain relief with a combination of low dosages of gabapentin (600 mg orally three times daily) and nortriptyline (50 mg orally at bedtime) than with either medication given alone at high doses. Importantly, patients receiving combination therapy had good analgesia without the significant side effects experienced by those treated with monotherapy. This trial, supported by the Canadian Institutes of Health, is a rare study in that the investigators had no influence from pharmaceutical companies and two inexpensive generic medications were studied.
A new gastric-retentive formulation of gabapentin (Gralise) has recently been approved by the FDA for PHN. It is intended to provide a simpler dosing paradigm than needed with the traditional generic gabapentin through the use of a polymer-based technology that allows gastric retention of the pill for extended delivery of the active medication. In a blinded RCT examining the analgesic benefit of single-day versus twice-a-day dosing in patients with PHN, the gastric-retentive formulation, when given twice per day, was more effective than placebo with an NNT of 5.9. The single-day dosing was not significantly different from placebo and had an NNT of 7.3, although a more recent multicountry RCT showed a significant improvement in pain after a single dose of 1800 mg given in the evening. Another formulation of gabapentin, gabapentin enacarbil (Horizant), has been developed and was initially approved for the treatment of restless legs syndrome. It is an actively transported prodrug form of gabapentin that allows twice-a-day dosing because of increased stability in bioavailability in comparison to the standard formulation of gabapentin. In a recent RCT, this prodrug formulation was found to be effective in the treatment of PHN when given twice per day. This drug is now approved by the FDA for the treatment of PHN as well as restless leg syndrome.
Pregabalin (Lyrica)
Pregabalin, like gabapentin, is used for the treatment of NP and acts by binding to the α 2 δ subunit of L-type voltage-gated calcium channels, which results in decreased neuronal excitation. With respect to NP, pregabalin is approved by the FDA for the treatment of PHN, PDN, and spinal cord injury–associated pain. Initial pregabalin dosing is 150 mg/day given in two or three divided doses or 25-50 mg given at bedtime in elderly patients. Upward dose titration can be completed after 3 to 7 days to 300 mg/day and subsequently increased to a maximum dose of 600 mg/day within 2 weeks of initiation. Similar to gabapentin, dosing of pregabalin must be decreased in patients with reduced kidney function. Advantages of pregabalin over gabapentin include a more rapid onset of pain relief; linear pharmacokinetics with low intersubject variability ; fewer dose-related side effects, thereby allowing faster upward dosage titrations; and twice-daily versus three times a day dosing. Additionally, maximum benefit often occurs after 2 weeks of treatment at target doses of 300 to 600 mg/day versus up to 2 months in gabapentin-treated patients.
In patients with PHN, a trial that included 370 patients was conducted to evaluate doses of 150, 300, or 600 mg/day versus placebo. The RCT demonstrated reduced mean pain scores and improvement in sleep interference. Patients responded at all dosages, with the greatest response noted with 600 mg/day. Patients responded as early as the first week, and beneficial effects were sustained throughout the 13-week study duration. Adverse effects were generally mild to moderate, and 13% of patients withdrew from the study, most commonly because of dizziness or somnolence.
In a randomized, double-blind study, the effects of pregabalin on PDN were evaluated. A total of 395 patients were randomized to receive 150, 300, or 600 mg/day. In patients who received 600 mg/day, 46% reported greater than 50% improvement in pain scores from baseline, and the NNT to achieve this response was 6.3. Pregabalin also improved pain-related sleep interference and was well tolerated overall, with an NNH of 10.3 in patients treated with 600 mg/day.
Pregabalin was evaluated in a 12-week multicenter study in patients with central NP secondary to spinal cord injury. A total of 137 patients were randomized either to a flexible-dose regimen of 150 to 600 mg/day or to placebo and were allowed to continue an existing stable pain regimen. Pregabalin was found to be significantly more effective in relieving central NP than placebo was.
Pregabalin has also been studied for use in patients with “refractory” NP of various origins. A 15-month open-label study was conducted in 81 patients with PHN and PDN refractory to treatment, including gabapentin, a TCA, and a third medication (e.g., other anticonvulsant, opioid, specific serotonin reuptake inhibitor, tramadol). Patients took 150 to 600 mg/day for 3-month intervals and then had a 3- to 28-day “drug holiday.” As evaluated by VAS scores, patients had a clinically meaningful and sustained reduction in pain intensity during the treatment cycle, with return of pain during “drug holidays.” In patients with an unsatisfactory response to other medications, pregabalin may be considered as an adjunctive therapy.
The advantage of pregabalin is its early response and favorable side effect profile. The most common adverse effects include somnolence and dizziness, and they occur more frequently with higher doses. When discontinuing pregabalin, it should be tapered down gradually over at least a 1-week period to minimize symptoms, including insomnia, nausea, headache, and diarrhea.
Zonisamide (Zonegran)
Zonisamide is indicated as adjunctive therapy for partial seizures in adults and became available in the United States in 2000. It acts by blocking T-type calcium channels and sodium channels; its action also increases release of GABA. The initial dose is 100 mg/day for 2 weeks with increases of 200 mg/wk to a target of 600 mg/day. There have been case reports on its usefulness for post-stroke pain and headache. A randomized, double-blind, placebo-controlled pilot study of the efficacy of zonisamide for the treatment of PDN revealed that pain scores on the VAS and Likert (psychometric response) scales decreased more in the zonisamide group than in the placebo group, but these differences did not reach statistical significance. Side effects included ataxia, decreased appetite, rash, and renal calculi (as a result of the carbonic anhydrase inhibitor effect). Zonisamide is contraindicated in those with sulfonamide allergy because it is a sulfonamide derivative, and the drug is approximately 40% bound to plasma proteins. Children have an increased risk for oligohidrosis and susceptibility to hyperthermia. The exact role of zonisamide in the management of patients with NP is yet to be elucidated, and further research is needed.
Ziconotide (Prialt)
Ziconotide is a ω-conopeptide (previously known as SNX-111) that is administered intrathecally because of its peptidic structure. It is derived from the venom of a marine snail (genus Conus ). Ziconotide blocks calcium influx into N-type calcium channels in the dorsal horn laminae of the spinal cord, thus preventing afferent conduction of nerve signals. Administration is via an intrathecal infusion pump, and dosing should be started low, at a recommended dose of 2.4 µg/day (0.1 µg/hr). Because of a lag time, it should be titrated up slowly at intervals of no more than two to three times per week to a recommended maximum of 19.2 µg/day. Ziconotide does not cause tolerance, dependence, or respiratory depression, and adverse effects primarily involve the CNS and include dizziness, ataxia, confusion, and headache.
Ziconotide has been evaluated in randomized, double-blind, placebo-controlled trials for severe, chronic, treatment-refractory pain in patients with or without cancer. Patients experienced a significant improvement in mean pain scores and global pain relief. The response rate was higher in patients receiving a maximum of 21.8 µg/day; however, pain relief was accompanied by a high incidence of adverse effects that resulted in frequent interruptions of the trial. A slow titration schedule with a lower maximum infusion rate was associated with significantly lower dropout rates but also resulted in a more modest treatment effect. At the conclusion of one trial, nearly 90% of patients elected to continue receiving ziconotide. Rare, but serious adverse effects include hallucinations; thus, ziconotide is not recommended for use in patients with a history of psychosis. Elevations in creatine kinase (CK) were noted in some studies to be related to ziconotide. The etiology remains unclear, and CK levels should be monitored periodically.
The role of ziconotide in the management of chronic pain has yet to be fully elucidated. Currently, ziconotide is approved for the management of severe chronic pain in patients in whom intrathecal therapy is warranted and who are intolerant of or refractory to other treatments, including intrathecal opiates; however, this medication should be used cautiously because of its poor side effect profile.
Nimodipine (Nimotop)
Nimodipine has been shown to decrease the dose of morphine needed for relief of cancer pain in 9 of 14 patients. In a colorectal surgery population, concomitant calcium channel blocker therapy did not decrease opioid requirements. Nimodipine taken concurrently with antiretroviral medications demonstrated a trend toward improvement or stabilization of human immunodeficiency virus (HIV)-associated neuropathy when compared with placebo.
Sodium Channel Blockers
Sodium channel blockers are used as primary therapy or adjunctive treatment of processes such as trigeminal neuralgia (TN), CRPS, PDN, radicular extremity pain, chemotherapy-induced peripheral neuropathy, and PHN. When using these agents, as with all membrane stabilizers, it is crucial to be knowledgeable of the proper dosages, toxicities, and their effects when coadministered with other drugs. As a general rule, the dose should be titrated to patient comfort within safety standards.
When neurons are depolarized and approaching an action potential, the voltage-gated sodium channels quickly change conformation in response and permit the flow of sodium ions. Activation of sodium channels (and other voltage-gated ion channels) derives from the outward movement of charged residues because of an altered electrical field across the membrane. Sodium channels play an essential role in the action potentials of neurons and other electrically excitable cells. The flow of sodium ions is terminated by inactivation of the channel in a few milliseconds (fast inactivation). Sodium channels can cycle open and close rapidly, which may result in seizures, NP, or paresthesias. The structure of the channel is essentially a rectangular tube, with its four walls formed from four subunits, the four domains of a single polypeptide. A region near the N-terminus protrudes into the cytosol and forms an inactivating particle. It has been demonstrated that a short loop of amino acid residues, acting as a flap or hinge, blocks the inner mouth of the sodium channel and results in fast inactivation. The highly conserved intracellular loop is the inactivating gate that binds to the intracellular pore and inactivates it within milliseconds. Site-directed antibody studies against this intracellular loop have prevented this fast inactivation.
The voltage-gated sodium channel can be divided into an α subunit and one or more auxiliary β subunits. At least nine α subunits have been functionally characterized—Na v 1.1 through Na v 1.9. The sodium channels 1.2, 1.8, and 1.9 are preferentially expressed on peripheral sensory neurons, where they are important in nociception and may be a future target for channel-specific analgesics. Seven of the nine sodium channel subtypes have been identified in sensory ganglia, such as the dorsal root ganglia and trigeminal ganglia. Na v 1.7 is also present in large amounts in the peripheral nervous system. Na v 1.2 is expressed in unmyelinated neurons and Na v 1.4 and Na v 1.5 are muscle sodium channels. Sodium channel mutations that cause well-recognized syndromes have been described. A mutation in Na v 1.4 is responsible for hyperkalemic periodic paralysis, and an inherited long QT syndrome can be caused by a mutation in Na v 1.5.
Increased expression of sodium channels has been demonstrated in peripheral and central sensory neurons in patients with NP; it is one mechanism for the observed hyperexcitability of pain pathways. Anticonvulsants that modulate the gating of sodium channels are phenytoin, lamotrigine, carbamazepine, oxcarbazepine, and zonisamide, with some evidence for topiramate and valproic acid. It is important to note that at clinical concentrations, the sodium channel is only weakly blocked when hyperpolarized. When the neuronal membrane is depolarized, there is a much greater inhibition of the channel. Binding of the channel by anticonvulsants is slow in comparison to local anesthetics. The slow binding of anticonvulsants ensures that the kinetic properties of normal action potentials are not altered. Generally, anticonvulsants have no role in the treatment of acute pain, although they have demonstrated efficacy in chronic pain conditions. Interestingly, local application of phenytoin and carbamazepine has an antinociceptive effect that is more potent than that of lidocaine. It has been demonstrated that phenytoin, carbamazepine, and lamotrigine bind to a common recognition site on sodium channels, and it is probably the result of their two phenol groups, which act as binding elements. At normal resting potentials, these medications have little effect on action potentials. In addition to the fast current of the open channel, there is also a persistent sodium current. This current, carried by persistent openings, is a small fraction of the fast current but may have an important role in regulating excitability. There is evidence that a number of anticonvulsants, such as phenytoin, valproate, and topiramate, also act by blocking the persistent sodium current, which is separate from the fast sodium current.
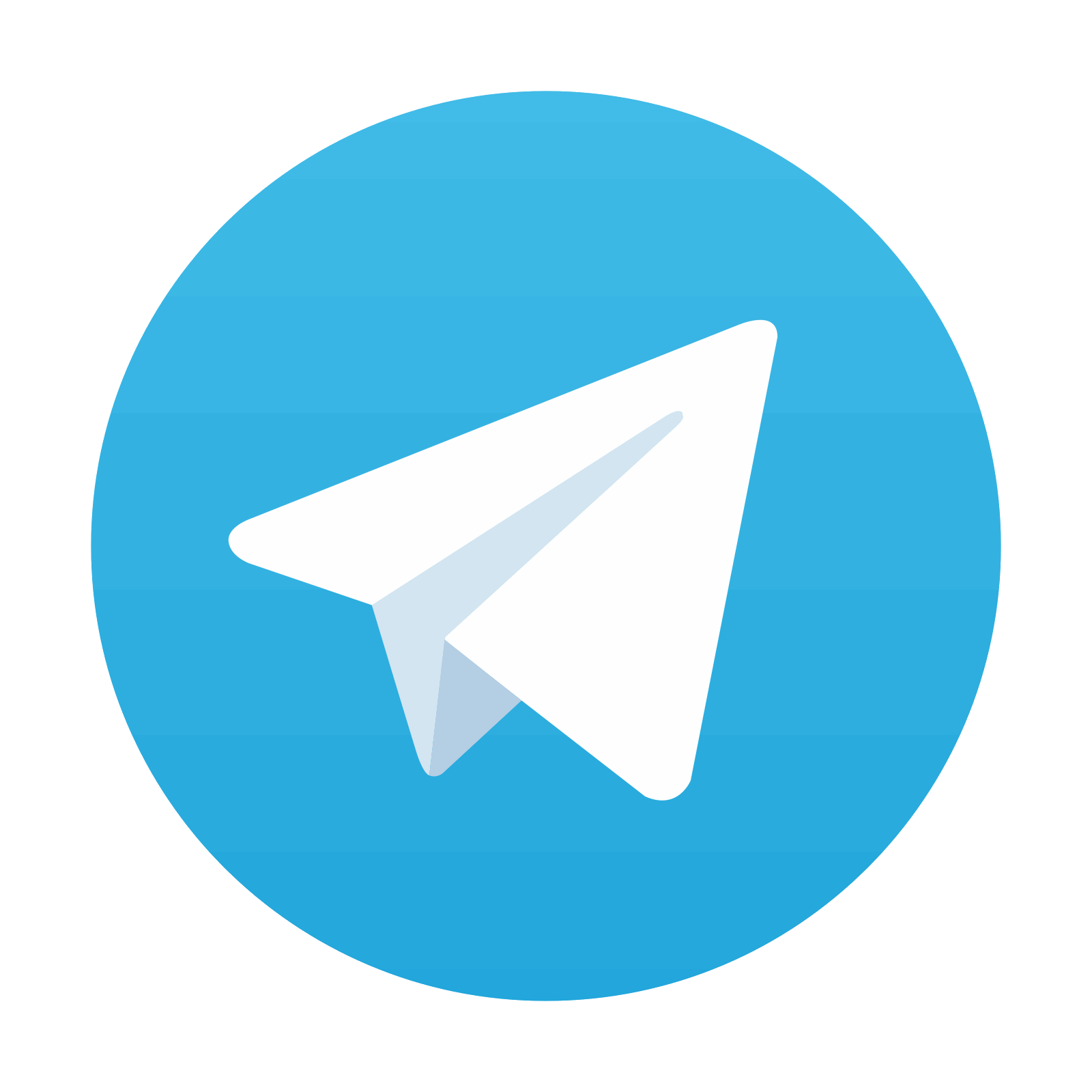
Stay updated, free articles. Join our Telegram channel

Full access? Get Clinical Tree
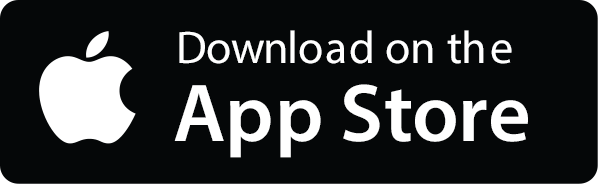
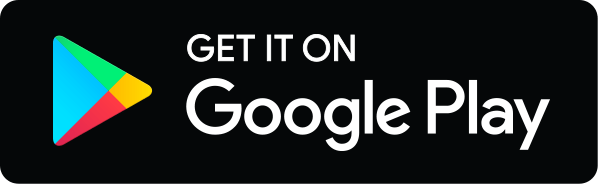