INTRODUCTION
Acute respiratory failure is a common acute critical condition, and initiation of mechanical ventilation is a required skill for all emergency physicians. The etiologies for respiratory failure are expansive, and the choice between invasive and noninvasive mechanical ventilation can be a challenging clinical decision. This chapter focuses on initiation of safe and effective invasive mechanical ventilation following endotracheal intubation. Chapter 6 focuses on respiratory failure requiring noninvasive mechanical ventilation.
Spontaneous ventilation draws air into the lungs under negative pressure, whereas mechanical ventilation uses positive pressure to provide airflow. In either case, the amount of negative or positive pressure required to deliver the tidal volume (Vt or TV) must overcome resistance to airflow. Positive-pressure ventilation alters normal pulmonary physiology by increasing airway and intrathoracic pressure, decreasing venous return to the thorax, and altering ventilation–perfusion matching in the lung.
TERMINOLOGY OF MECHANICAL VENTILATION
The following variables are important to best understand mechanical ventilation:
• Vt or TV is the volume of a single breath. During mechanical ventilation, the contemporary safe goal TV is ≤7 mL per kg of ideal body weight (IBW). It is important to note that IBW is more a function of patient height rather than weight or overall size. TVs may be reduced (to 6 or lower mL per kg IBW) in certain circumstances to minimize ventilator-induced lung injury (VILI) associated with excessive airway pressure that risk overdistention of functional lung units.
The airway conduits do not exchange gas and therefore represent anatomical dead space that accounts for a fixed volume of each tidal breath. The remaining TV participates in gas exchange and constitutes alveolar ventilation. As TV is reduced, anatomical dead space makes up a proportionally larger portion of each breath. It is important to increase minute ventilation through enhanced respiratory rate (RR) to balance the decrease in effective alveolar ventilation with TV reduction.
• RR or frequency (f ) is simply the number of breaths per minute. Usual starting RR is 12 to 20 breaths per minute in adults. Higher rates are typical in neonates, infants, and small children.
Given our attention to low-TV ventilation, even in patients without lung injury, minute ventilation is typically modified by increasing RR rather than TV. In addition to compensating for the relative proportion of dead space mentioned earlier, enhanced RR may be used to provide compensation for metabolic acidosis or enhanced carbon dioxide (CO2) production (e.g., fever/hyperthermia, sepsis, and hypermetabolic conditions).
In reactive airways diseases, the concept of permissive hypercapnia refers to the use of low RR (8 to 10 breaths per minute), allowing for adequate expiratory time for complete exhalation of the former TV to avoid trapped air that leads to dynamic hyperinflation and auto-Positive end-expiratory pressure (PEEP).
• Fractional concentration of inspired oxygen (FIO2) ranges from the concentration of oxygen in room air (0.21 or 21%) to that of pure oxygen (1.0 or 100%). When initiating mechanical ventilation, start with a FIO2 of 100% and reduce the FIO2 based on continuous pulse oximetry. During acute critical illness, titrating FIO2 to maintain SpO2 ≥95% is appropriate for most conditions. The lowest FIO2 required to maintain adequate oxygenation should be used.
• Inspiratory flow rate (IFR) is the rate at which a TV is delivered during inspiration. In an adult, this is typically set at 60 L per minute. Cases of reactive airways disease may require peak IFR to be increased to 90 to 120 L per minute in order to shorten the inspiratory time (Ti), and thus increase expiratory time and diminish dynamic hyperinflation.
• PEEP provides a static pressure to the airways during inspiration and expiration, and is typically set at a minimum of 5 cm H2O. PEEP increases alveolar recruitment, functional residual capacity, total lung volume, and pulmonary and intrathoracic pressure. When a patient is unable to meet oxygenation goals on FIO2 > 50%, PEEP is typically increased to augment mean airway pressure to improve oxygenation. However, excessive PEEP can lead to pathologic overdistention of healthy lung tissue contributing to ineffective gas exchange and VILI. Elevated intrathoracic pressure can also compromise venous return with consequent hemodynamic deterioration.
• Peak inspiratory pressure (PIP) and plateau pressure (Pplat): The PIP is the greatest pressure reached during the inspiratory cycle, and is a function of the ventilator circuitry, endotracheal tube (ETT), ventilator flow rate, and patient lung and thoracic compliance. It is useful for rapid assessment of a patient during acute changes, but does not accurately reflect lung compliance or the risk of VILI. Risk of pulmonary overdistention is better represented by the Pplat, measured at the end of inspiration (via an inspiratory pause). The inspiratory pause enables equilibration of pressure between the ventilator and lung units to measure the static pressure of the thoracic compartment. Pplat correlates with the risk of VILI, and current recommendations aim to maintain safe Pplat ≤ 30 cm H2O via reduction in TV when advanced lung disease is the cause for elevated Pplat.
VENTILATION MODES
There are a variety of modes of invasive mechanical ventilation, and the key to understanding the differences between these modes centers on three variables: the trigger, the limit, and the cycle.
• The trigger is the event that initiates inspiration: either patient effort or machine-initiated positive pressure.
• The limit refers to the airflow parameter that is used to regulate inspiration: either airflow rate or airway pressure.
• The cycle terminates inspiration: via delivered set volume in volume-control ventilation (VCV), pressure delivered over a set time period in pressure-control ventilation (PCV), or by patient termination of inspiratory effort in pressure support ventilation (PSV).
• The best mode in a given circumstance depends on the needs of the patient.
Commonly used ventilation modes are as follows:
• Control mode ventilation (CMV) is almost exclusively relegated to the operating room in sedated and paralyzed patients, but an understanding of this mode provides appreciation of the support provided through other modes. In CMV, all breaths are triggered, limited, and cycled by the ventilator. The clinician sets the TV, RR, IFR, PEEP, and FIO2. The ventilator then delivers the prescribed TV (the cycle) at the set IFR (the limit). Even if the patient wanted to initiate an additional breath, the machine would not respond. In addition, if the patient has not completely exhaled before initiation of the next breath, the machine would generate the required pressure to deliver the full TV breath. For these reasons, CMV is only used in those patients who are sedated and paralyzed.
• Assist control (AC) is the preferred mode for patients with acute respiratory failure. The clinician sets the TV, RR, IFR, PEEP, and FIO2. In contrast to all other modes, the trigger that initiates inspiration can be either patient effort or an elapsed time interval. When either of these occurs, the ventilator delivers the prescribed TV. The ventilator synchronizes set RRs with patient efforts, and if the patient is breathing at or above the set RR, then all breaths are patient initiated. The work of breathing (WOB) is primarily limited to the patient’s effort to trigger the ventilator and can be altered by adjusting the sensitivity threshold.
• Synchronized intermittent mandatory ventilation (SIMV with or without PS) is commonly misunderstood and can lead to excessive patient WOB. The physician sets the TV, RR, IFR, PEEP, and FIO2. Importantly, the trigger that initiates inspiration depends on the patient’s RR relative to the set RR. When the patient is breathing at or below the set RR, the trigger can be patient effort or elapsed time. In these cases, the ventilator operates similar to an AC mode. If the patient is breathing above the set RR, the ventilator does not automatically assist the patient efforts, and the TV is determined by effort and resistance to airflow through the ETT and ventilator circuit. In these instances, WOB can be excessive.
Addition of PSV to the SIMV mode provides a set inspiratory pressure that is applied during patient-initiated breaths, which exceed the set RR. Appropriate PSV balances the inherent resistance of the artificial airways and supports the patient’s physiologic situation to limit undue WOB. Insufficient PSV is associated with high RR and low TV, also known as rapid, shallow breathing. Sustained tachypnea greater than 24 breaths per minute is a helpful marker to consider evaluating if the PSV level is appropriate to the patient condition. SIMV provides no clear benefit over AC mode ventilation. Although previously used as a weaning mode wherein the set rate is progressively decreased to allow the patient to assume increased WOB, the absence of additional PSV substantially increases WOB and is frequently overtaxing. Spontaneous breathing trials using minimal PSV, without SIMV, is the current standard approach to assess readiness for liberation from mechanical ventilation.
• Continuous positive airway pressure (CPAP) is not a true mode of invasive mechanical ventilation. It is equivalent to PEEP in that it provides a static positive airway pressure throughout the entire respiratory cycle.
In a fashion similar to SIMV, PS can be added to CPAP to function as an assisted form of ventilation. In the CPAP-PS mode, the patient determines the RR, initiating and terminating each breath. The TV is dependent on patient effort and the degree of PS relative to the resistance of the airway circuit. This mode should never be used in patients at risk for hypoventilation or apnea, because there is no mandatory backup rate to support the patient in case of failure.
VENTILATOR TV DELIVERY
Volume-Control Ventilation
In this method of delivering a breath, the operator sets the TV of each breath. The pressure required to deliver this volume varies by the flow rate selected, the resistance of the airway circuit and lungs, and compliance of lungs and thorax. In adults, the initial peak flow is usually set to 60 L per minute.
VCV also allows selection of the flow characteristics of a delivered breath. The waveform may be square or decelerating (Fig. 7-1). A square wave delivers the TV at a constant peak flow throughout inspiration. This waveform usually generates a higher peak pressure than the decelerating waveform, but has the advantage of a shorter Ti, providing more time for expiration. A decelerating flow wave delivers the initial TV at a selected flow that decelerates as the breath is delivered. Because resistance to flow normally increases during inspiration, the decelerating waveform generally results in lower PIP. This approach increases Ti at the expense of expiratory time, which may potentiate dynamic hyperinflation and auto-PEEP. For this reason, the IFR set for decelerating flow wave is usually higher than that used in a square wave flow pattern. When setting up the ventilator, one can try different flow patterns to determine which offers the best patient synchrony.
• FIGURE 7-1. Volume-Control Ventilation (VCV). The lower trace demonstrates a square flow waveform first followed by a decelerating waveform. Note that the peak pressure generated by the square waveform exceeds that of the decelerating waveform. The third waveform demonstrates breath initiation before expiratory flow reaches zero. This is an example of breath stacking that leads to dynamic hyperinflation and auto-PEEP.
Pressure-Control Ventilation
PCV should not be confused with PSV ventilation, described previously. The limit during PCV is a set airway pressure. Instead of TV, the cycle during PCV is a set Ti. Some PCV ventilator models require a set RR and inspiratory to expiratory (I:E) ratio. Ti is then calculated by the ventilator based on these settings. The clinician specifies an inspiratory pressure and an inspiratory–expiratory (I:E) ratio predicted to provide a reasonable TV and RR, based on the patient’s expected resistance and compliance. TV may vary from breath to breath based on airway resistance, lung compliance, and patient effort, but it should generally meet the same ≤7 mL per kg IBW goal discussed earlier.
In this mode, the peak flow of the administered tidal breath and the flow waveform vary according to airway and lung characteristics. Early in inspiration, the ventilator generates a flow rate that is sufficiently rapid to reach the preset pressure and then automatically alters the flow rate to stay at that pressure, and cycles off at the end of the predetermined Ti. The flow waveform created by this method is a decelerating pattern (Fig. 7-2). A normal I:E ratio is 1:2. If the RR is 10 breaths per minute evenly distributed over a minute, each cycle of inspiration and expiration is 6 seconds. With an I:E ratio of 1:2, inspiration is 2 seconds, and expiration is 4 seconds.
The I:E ratio is usually determined by simply observing the pressure and flow waveforms on the ventilator monitor. After the inspiratory pressure is adjusted to meet the target TV, the Ti is adjusted while monitoring end-expiratory flow. Terminal end-expiratory flow should approach zero to confirm exhalation is complete, and avoid retained intrathoracic volume. Small fractions of retained TV compounded by the ventilator rate can quickly lead to dynamic hyperinflation with increased intrathoracic pressure known as auto-PEEP that affects venous return, respiratory mechanics, and can put the patient at risk for barotrauma (Fig. 7-3). In contrast, overly brief Ti can lead to low TVs and hypoventilation.
• FIGURE 7-2. Pressure-Control Ventilation (PCV). These waveforms demonstrate the differing waveform characteristics between volume-control (VCV) and PCV. Note that PCV generates lower peak pressures than VCV.
• FIGURE 7-3. Pressure-Control Ventilation and Inspiratory–Expiratory Ratio. The first waveform demonstrates inspiratory time that is so short that the tidal volume is likely insufficient. The second and third waveforms demonstrate how prolonged inspiratory time may contribute to breath stacking and auto-PEEP.
INITIATING MECHANICAL VENTILATION
The patient who is spontaneously breathing possesses a complex series of physiologic feedback loops that control the volume of gas moved into and out of the lungs each minute (minute ventilation). They automatically determine the RR and the volume of each breath necessary to effect gas exchange and maintain homeostasis. Patients dependent on ventilators have no such servo control and must rely on individualized ventilator settings to meet their physiologic needs. In the past, this required frequent blood gas determinations. Now we rely more heavily on noninvasive techniques such as pulse oximetry and end-tidal CO2 monitoring.
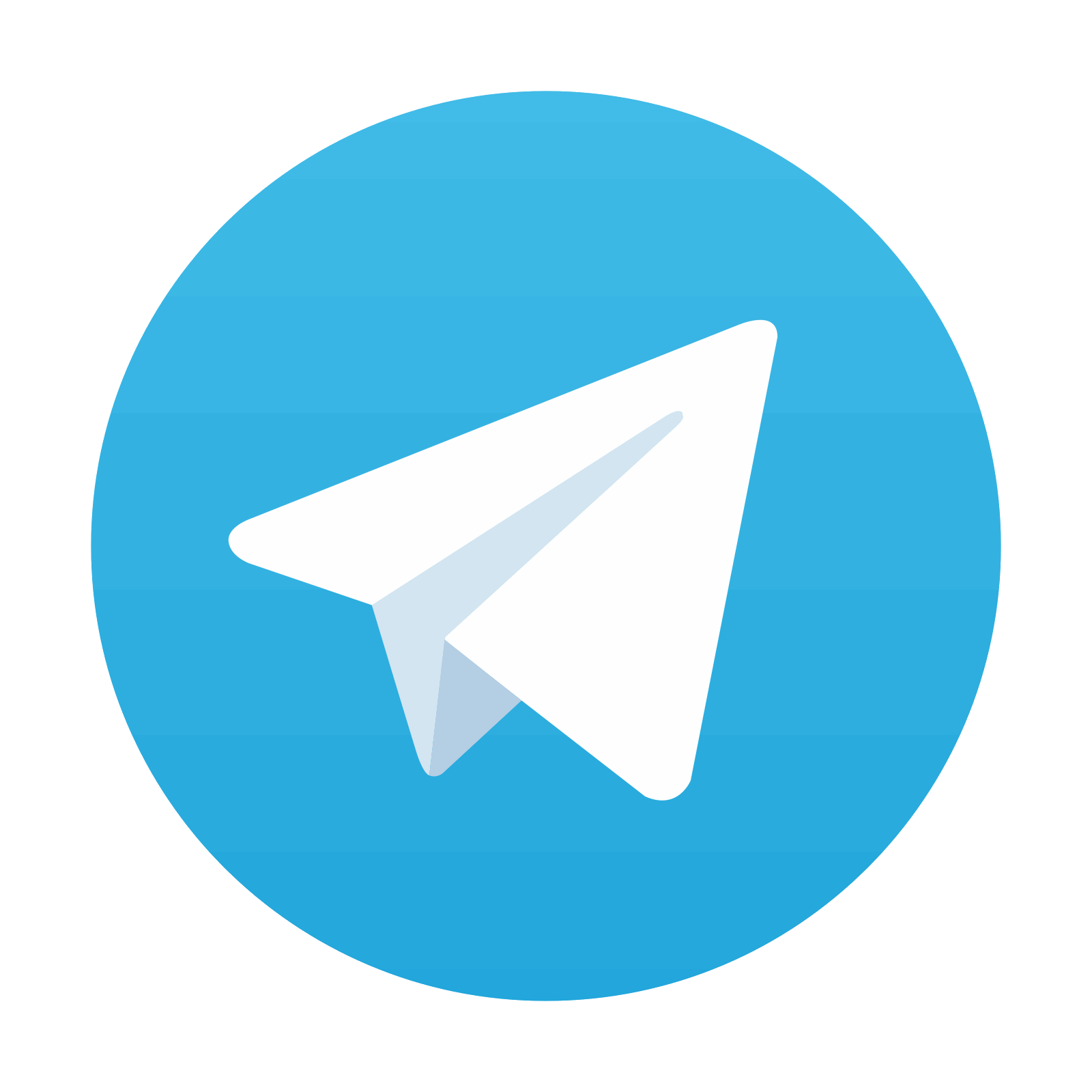
Stay updated, free articles. Join our Telegram channel

Full access? Get Clinical Tree
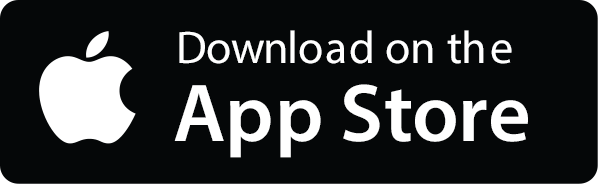
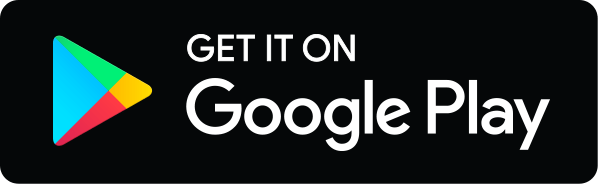