Mechanical Complications of Myocardial Infarction
Annabel A. Chen-Tournoux
Michael A. Fifer
Pathophysiology Underlying Myocardial Stunning and Its Time Course Following Ischemia and Reperfusion
Within 8 to 10 seconds after occlusion of an epicardial coronary artery, myocardial oxygen supply is exhausted, resulting in a shift from aerobic to anaerobic metabolism. High-energy phosphates (creatine phosphate and adenosine triphosphate [ATP]) become depleted, whereas hydrogen ions, lactate, and other metabolic products accumulate, causing intracellular pH to fall to 5.8 to 6.0 within 10 minutes of the onset of ischemia [1]. In addition, adenosine monophosphate (AMP) is degraded to adenosine, which diffuses into extracellular fluid, depleting the intracellular adenine nucleotide pool. The ischemic myocardium stretches instead of shortens during systole, corresponding to regional wall motion abnormalities observed with imaging modalities, such as echocardiography. Electrocardiogram (ECG) changes appear as well. Disruption of the cell membrane allows protein leakage out of the cell, producing serologic evidence of myocyte injury. If blood flow is restored within 15 minutes of coronary occlusion, myocyte injury is reversible. Glycolysis ceases after approximately 40 minutes of severe ischemia, after which time injury becomes irreversible and myocytes are not salvageable by reperfusion. Even with earlier restoration of blood flow, however, a phenomenon called myocardial stunning is observed.
First described in a dog model by Heyndrickx et al. [2] in 1975, stunning is defined as prolonged contractile dysfunction occurring after relief of a discrete episode or episodes of ischemia. Importantly, the dysfunction associated with stunning is completely reversible. By definition, myocardial perfusion must be restored to normal or near normal to distinguish stunning from myocardial dysfunction due to continued ischemia (hibernation). The severity and duration of stunning depend on
multiple factors, such as the extent of the original ischemic insult, the adequacy of restored flow, the presence of preexisting collateral vessels, and prior ischemic preconditioning. In general, the myocardium is stunned for a period longer than that of the ischemic insult, often requiring hours to days to regain function [3].
multiple factors, such as the extent of the original ischemic insult, the adequacy of restored flow, the presence of preexisting collateral vessels, and prior ischemic preconditioning. In general, the myocardium is stunned for a period longer than that of the ischemic insult, often requiring hours to days to regain function [3].
Although early restoration of flow is necessary for myocardial survival, reperfusion is also thought to underlie the pathogenesis of stunning, through the development of oxidative stress and/or impaired calcium homeostasis [4]. In the first 5 minutes of reperfusion, there is marked hyperemia, with a 400% to 600% increase in flow, returning to the baseline level after 15 to 20 minutes. Because levels of oxygen-free radicals peak at 4 to 7 minutes, most of the free radical-induced injury responsible for stunning is thought to occur in the initial moments following reperfusion. Blunted calcium transients and dysfunction of the ryanodine receptor and the sarcoplasmic reticulum calcium ATPase (SERCA-2), which would lead to impaired myocyte excitation–contraction coupling, have also been described following ischemia–reperfusion. Other possible mechanisms of reperfusion injury involved in myocardial stunning include microvascular injury, endothelial cell dysfunction, and activation of neutrophils, platelets, and the complement system.
Stunning is observed in clinical scenarios in which the heart is reperfused after transient ischemia, whether it be global, as with cardioplegia during cardiac surgery or transplant harvest, or regional, as with acute coronary syndromes, percutaneous coronary interventions (PCI), or exercise-induced angina. In patients with coronary disease, stunning from repeated episodes of demand ischemia may lead to chronic left ventricular (LV) dysfunction.
Stunned, but viable, myocardium may be identified by echocardiographic, scintigraphic, and magnetic resonance imaging techniques [5]. Because ischemic myocytes have different rates of injury and recovery, the timing of improvement after acute myocardial infarction (MI) is variable and often unpredictable. The major clinical implication of stunning is that even brief periods of ischemia may be associated with prolonged contractile dysfunction. Moreover, because this dysfunction may be fully reversible, continued hemodynamic support, with intra-aortic balloon counterpulsation and/or inotropic agents such as catecholamines or phosphodiesterase inhibitors, may be indicated. Importantly, inotropic stimulation does not appear to worsen cell injury as long as the reperfused artery is patent. Finally, myocardial stunning has implications for the timing of evaluation of LV function to guide therapeutic decisions after MI. For example, LV ejection fraction assessment for implantable cardiac defibrillator implantation is generally deferred for at least 1 month following MI.
Table 40.1 National Registry of Myocardial Infarction: All-Cause In-Hospital Mortality for Patients with Acute Myocardial Infarction | |||||||||||||||||||||||||||||||||||||||||||||
---|---|---|---|---|---|---|---|---|---|---|---|---|---|---|---|---|---|---|---|---|---|---|---|---|---|---|---|---|---|---|---|---|---|---|---|---|---|---|---|---|---|---|---|---|---|
|
Diagnosis, Treatment, and Outcome of Shock Due to Left Ventricular Pump Failure
Approximately 5% to 8% of patients with ST-segment elevation MI (STEMI) and 2.5% of patients with non–ST-segment elevation MI develop cardiogenic shock (CS), the leading cause of death in patients hospitalized with MI (Table 40.1). CS is broadly defined as a state of end-organ hypoperfusion due to cardiac failure. Clinical evidence of systemic hypoperfusion includes altered mental status, cold clammy skin, and oliguria. Hemodynamic parameters of CS include persistent (≥ 1 hour) hypotension (systolic blood pressure < 80 to 90 mm Hg or mean arterial pressure 30 mm Hg lower than baseline) not responsive to fluid or requiring inotropic or vasopressor support to be maintained; low cardiac index (< 1.8 L per minute per m2 without support or 2.0 to 2.2 L per minute per m2 with support); and adequate or elevated filling pressures (LV end-diastolic pressure > 15 mm Hg or right ventricular [RV] end-diastolic pressure > 10 to 15 mm Hg).
In the absence of mechanical complications, the primary insult in CS associated with MI is LV dysfunction due to extensive infarction or ischemia. Although the magnitude of myocardial insult does not correlate perfectly with the development of CS [6], LV function nevertheless remains a prognostic factor in CS [7].
The observation of normal to low systemic vascular resistance among many patients with CS [8] suggests an important role for inappropriate vasodilation in CS. Indeed, neurohormonal and cytokine abnormalities consistent with the systemic inflammatory response syndrome (SIRS) have been observed (Fig. 40.1) [6]. For example, cytokines with myocardial depressant activity, such as tumor necrosis factor (TNF)-α and interleukin (IL)-6, increase over 24 to 72 hours after MI. MI is also associated with abnormal NO metabolism [9] and increased expression of inducible nitric oxide (NO) synthase; NO excess causes vasodilation, depressed myocardial contractility, and interference with catecholamine action in CS. Despite the
growing recognition of SIRS associated with CS, therapies targeting it remain unproven at this time.
growing recognition of SIRS associated with CS, therapies targeting it remain unproven at this time.
Most cases of CS after acute coronary syndrome develop after hospital presentation, with a median time of 10 to 11 hours (STEMI) and 76 hours (non–ST-segment elevation MI) [10]. Predictors for CS have varied among different studies over time and include older age; prior MI, heart failure, diabetes, hypertension, or cerebrovascular disease; failed reperfusion; lower blood pressure and glomerular filtration rate; and higher heart rate and serum glucose at presentation [6,11]. The only way to prevent CS appears to be very early reperfusion therapy for MI, whether through PCI or thrombolysis.
Outcome in CS is closely related to the patency of the infarct-related artery, in both retrospective analyses [12] and the prospective, randomized SHOCK (should we emergently revascularize occluded coronaries in cardiogenic shock) trial. In this multicenter study, patients with acute MI and CS were randomly assigned to early (within 6 hours) percutaneous or surgical revascularization (152 patients) or initial medical stabilization with subsequent revascularization permitted 54 hours after randomization (150 patients) [13,14]. Fibrinolysis was recommended in the initial medical stabilization group, and intra-aortic balloon counterpulsation was recommended in both treatment groups. Although there was an excess of death in the early revascularization group in the first 5 days, likely related to procedural complications, early revascularization improved survival at 6 months and 1 year (46.7% vs. 33.6%; p < 0.03), a benefit that remained stable at 3 and 6 years [15]. Although the benefit of revascularization increases the earlier it is achieved, there is a survival benefit as long as 48 hours after MI and 18 hours after shock onset. The benefit of early revascularization is similar for different subgroups (patients with diabetes, women, patients with prior MI, early vs. late shock) and whether revascularization is achieved with PCI or coronary artery bypass graft surgery [16]. Among patients undergoing PCI, registry data indicate that stenting and glycoprotein IIb/IIIa inhibitors are independently associated with improved outcomes [17]. On the basis of these results, emergency revascularization is recommended (class I) for patients younger than 75 years with MI and CS, who are determined to be suitable candidates [18]. If revascularization is not available, fibrinolysis and intra-aortic balloon pump placement followed by transfer to another facility is recommended.
In the SHOCK trial, lack of benefit with early revascularization was noted for patients 75 years and older, possibly due to imbalances in baseline ejection fraction. Later studies, including the SHOCK registry [19], have shown a consistent benefit of revascularization in elderly patients selected for it. Thus, an individualized approach weighing the risks and benefits of an aggressive revascularization strategy is warranted for elderly patients.
Multivessel or left main disease is extremely common in patients with MI and CS. Coronary bypass surgery is recommended for extensive disease [18], although PCI of the infarct-related artery may be initially necessary to stabilize the patient.
In addition to early revascularization, supportive therapy with inotropic agents and vasopressors (and avoidance of negative inotropes and vasodilators) is critical. Diuretics or intravenous fluids may be required, depending on the intravascular volume status. Routine antithrombotic therapy for MI includes aspirin, heparin, and if immediate surgery is unlikely, clopidogrel. Oxygen supplementation is standard and mechanical ventilation may be necessary. Intensive insulin therapy is also recommended in critically ill patients [18].
Hemodynamic management of CS may be guided by pulmonary artery catheter monitoring and echocardiography. Such monitoring also allows detection of mechanical complications such as papillary muscle or ventricular septal rupture (VSR).
The principal mechanical therapy for CS is intra-aortic balloon counterpulsation, which augments coronary perfusion and reduces cardiac afterload. For some patients who require a bridge to recovery or subsequent transplantation, short-term support may be offered in the form of LV assist device (LVAD) or extracorporeal life support [20]. Comparisons of percutaneous LVAD to intra-aortic balloon counterpulsation (IABP) have shown similar mortality rates [21,22,23].
Independent predictors of mortality in CS have varied in different studies over time, and include older age; history of hypertension, MI, or heart failure; lower blood pressure and worse renal function on presentation; failed reperfusion; and low LV ejection fraction [7,11,12,15]. Revascularization provides benefit at every level of risk, and registry studies in the United States and Europe have indicated significant decline (approximately 60% to 48%) in mortality from CS in recent years, in parallel with increasing revascularization with PCI [11,24,25].
Right Ventricular Infarction
Right ventricular infarction (RVI) has been recognized as a distinct entity since the initial description of its unique clinical and hemodynamic features in 1974 [26]. Most cases of RVI are due to proximal occlusion of a dominant right coronary artery, and RVI has been described in up to 50% of patients with inferior MI. (Very rarely, RVI may accompany anteroseptal MI due to a culprit left anterior descending artery lesion.) RVI leads to RV hypokinesis and decreased delivery of LV preload across the pulmonary vasculature. In addition, acute RV dilation in the face of the restraining effects of the pericardium leads to elevated intrapericardial pressure and leftward shifting of the interventricular septum, further compromising LV filling [27].
Early recognition of RVI is crucial because of its implications for management and prognosis, so that involvement of the RV should be considered in all patients with inferior MI. Clinical indicators of RVI include hypotension in the setting of clear lungs and elevated jugular venous pressure, although the latter may not be evident if the patient is relatively hypovolemic. Conversely, a volume-depleted patient may exhibit sensitivity to preload reduction, such as with the use of nitrates or diuretics. Patients may also display evidence of interventricular dependence, such as Kussmaul’s sign (distention of jugular veins during inspiration), more classically associated with pericardial disease.
Several ECG signs indicate RV involvement: ST-segment elevation in lead 3 greater than in lead 2, ST-segment elevation in lead V1, and ST-segment elevation in right-sided precordial lead V4R, the latter being the most predictive [28] (Fig. 40.2). These ECG abnormalities may resolve quickly (50% within 10 hours) [29], underscoring the importance of obtaining a right-sided ECG on presentation for all patients with inferior MI. RVI may be associated with bradyarrhythmias (sinoatrial or atrioventricular [AV] block) and tachyarrhythmias (atrial fibrillation and ventricular tachyarrhythmias). Echocardiography reveals RV dilation and hypokinesis and abnormal septal motion, along with inferior LV hypokinesis, and possibly other complications of RVI, such as tricuspid regurgitation, VSR, RV mural thrombus and pulmonary embolism, and right-to-left shunting across a patent foramen ovale. A small study suggests that late-enhancement magnetic resonance imaging has superior sensitivity to detect RVI compared with physical examination, ECG, and echocardiography [30]. Finally, right heart catheterization demonstrating a right atrial pressure equal or greater than 10 mm Hg or greater than 80% of the pulmonary capillary wedge pressure supports the diagnosis of RVI [26].
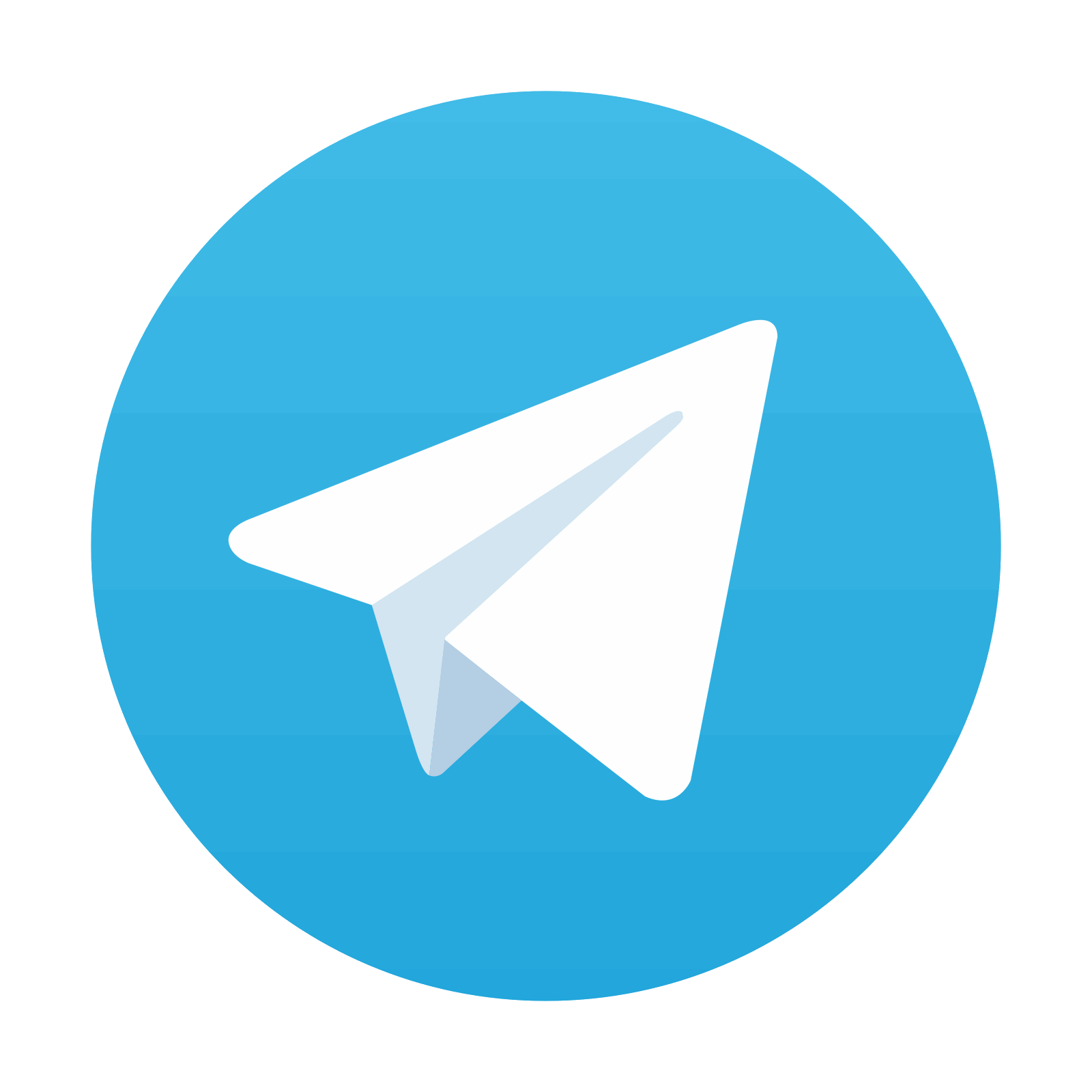
Stay updated, free articles. Join our Telegram channel

Full access? Get Clinical Tree
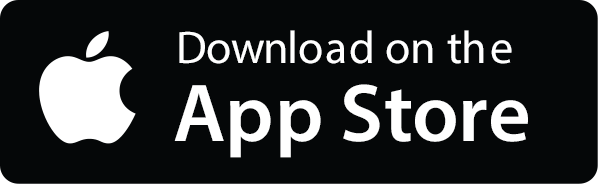
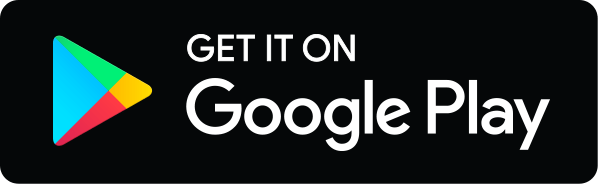