Abstract
Shock is defined as failure of the circulatory system resulting in a level of perfusion to tissues, which is inadequate to meet the oxygen demands of cellular metabolism. Haemorrhagic shock is most commonly associated with trauma. Haemorrhage is a leading cause of preventable death in trauma and over the past two decades there has been an increasing understanding of the pathophysiological processes that occur in major haemorrhage associated with trauma. This has been fundamental to the development of the current approach to management of traumatic shock, known as damage control resuscitation (DCR). DCR encompasses three key resuscitative strategies, permissive hypotension, haemostatic resuscitation (the use of blood products as primary resuscitative fluids) and damage control surgery. The implementation of DCR alongside the creation of trauma networks has been revolutionary in the management of the shocked trauma patient.
After reading this article, you should be able to:
- •
describe the physiological response to haemorrhage
- •
describe the lethal triad of trauma
- •
describe the clinical management of traumatic shock
- •
explain the role and benefits of the trauma team
Physiology of shock
Shock
Shock is defined as failure of the circulatory system resulting in a level of perfusion to tissues, which is inadequate to meet the oxygen demands of cellular metabolism. The predominant aetiology of shock in trauma is haemorrhage. It is important, however, to recognize that other causes of shock may be present in patients who have sustained major trauma. These include cardiogenic shock secondary to blunt force chest trauma, obstructive shock due to cardiac tamponade and/or tension pneumothorax and neurogenic shock in the context of spinal cord injury. This article will focus on haemorrhagic shock in trauma.
Physiological response to haemorrhage
The observed physiological response to acute haemorrhage is dependent on both the degree of haemorrhage and the pre-morbid physiological state of the patient. Younger patients without comorbid disease will compensate more effectively and for longer periods of time than the elderly and those with significant underlying cardiovascular disease. In the healthy patient, blood loss that does not exceed 15% of the circulating volume is unlikely to result in notable changes in either heart rate or blood pressure. As blood loss approaches 30% of the circulating volume, an increase in heart rate and decrease in pulse pressure will be observed, but at this level arterial blood pressure will continue to be preserved due to a combination of increased cardiac output as well as vasoconstriction in both the arterial and venous systems. Once blood loss exceeds 40% of the circulating volume (approximately 2 litres in the 70-kg patient) shock becomes decompensated and a fall in arterial blood pressure associated with an increase in pulse, respiratory rate, cessation of urine production and deterioration in the patient’s conscious level will be observed. These measurable physiological signs will be associated with a failure of tissue oxygen delivery and a resultant shift to anaerobic metabolism at cellular level.
The lethal triad
The interplay between acidosis, hypothermia and trauma-induced coagulopathy has been termed the lethal triad due to the universally poor prognosis that this combination of physiological abnormalities conveys if left uncorrected.
Acidosis
Hypoperfusion of tissues results in inadequate cellular oxygen delivery causing a shift from aerobic to anaerobic forms of metabolism producing lactate and free radicals, which contribute to a developing metabolic acidosis. In addition, lack of adenosine triphosphate (ATP) availability as a result of the switch to relatively inefficient forms of anaerobic metabolism compromises cell membrane integrity resulting in cellular oedema. This obstructs adjacent capillaries, worsening oxygen delivery and impairing the clearance of ischaemic metabolites, which begin to damage cells, initiating inflammatory cascades. The resulting inflammatory mediators produced act as negative inotropes, reducing cardiac output and further impairing oxygen delivery to tissues. Sustained acidosis will inhibit the function of both platelets and clotting factors, with the resulting coagulopathy further worsening blood loss.
Hypothermia
Environmental exposure, the removal of clothing and the administration of cold intravenous fluids or blood all serve to put patients who have experienced major trauma at risk of becoming hypothermic. In addition, the switch to anaerobic forms of metabolism decreases endogenous heat production. Hypothermia results in increased vasoconstriction, which will further impair tissue perfusion and worsen acidosis. Severe hypothermia will also have a negative effect on platelet function and inhibit enzymes required for the clotting cascade, worsening coagulopathy and increasing blood loss.
Trauma-induced coagulopathy
Trauma-induced coagulopathy (TIC) may be defined as a multifactorial global failure of the coagulation system to sustain adequate haemostasis after haemorrhage resulting from major trauma. It is characterized by a primary endogenous process, acute traumatic coagulopathy (ATC), which may then be further worsened by resuscitation-induced coagulopathies. ATC is common, occurring in up to a quarter of trauma patients, with changes in coagulation (hypocoagulation and hyperfibrinolysis) being observed within minutes of injury. The aetiology of ATC is linked to protein C activation, which occurs following the generation of thrombomodulin (TM) on the endothelium in response to tissue hypoperfusion, combined with thrombin formation in response to tissue trauma. Activated protein C inactivates coagulation factors and promotes fibrinolysis. Platelet function is also observed to be impaired early in the period following injury. Haemodilution of clotting factors due to the administration of intravenous fluids or packed red cells will further worsen coagulopathy, while acidosis and hypothermia will impair platelet function and inhibit clotting factor activity. Clotting factor consumption may also play a role.
Clinical management of haemorrhagic shock due to trauma
Damage control resuscitation (DCR)
The overall aims of DCR are to minimize blood loss, maximize tissue oxygenation and optimize outcome. The intention is that DCR begins at the point of injury, continuing as far as the care that the patient receives in the intensive care unit following surgery, with treatment at all times being targeted towards achieving these aims. This involves rapid control of major haemorrhage and the use of permissive hypotension in the pre-hospital setting, early haemostatic resuscitation, prompt imaging followed by appropriate damage control surgery and postoperative optimization in an intensive care setting.
Haemorrhage control
In patients who have developed haemorrhagic shock secondary to major trauma, haemorrhage control must be prioritized. Recognition of this fact has led to the widespread adoption of the <C>ABCDE pneumonic for the management of major trauma with <C> representing control of catastrophic haemorrhage. This acknowledges the fact that on-going exsanguinating haemorrhage will result in death before uncorrected airway or breathing issues. In the pre-hospital setting, haemorrhage control may be achieved through simple measures such as applying sustained pressure to compressible haemorrhage, the application of haemostatic dressings, the use of tourniquets to occlude arterial blood flow to limbs and the application of traction splints and pelvic binders, or through novel techniques such as resuscitative endovascular balloon occlusion of the aorta (REBOA).
In the hospital setting, haemorrhage control should be achieved through damage control surgery and/or interventional radiology procedures, where the priority is to achieve rapid haemostasis and limit contamination rather than the definitive surgical repair of injuries. This is then followed by a period of metabolic optimization in an intensive care environment prior to any further operative intervention. This approach is derived from a recognition that patients are more likely to die as a result of the metabolic consequences of their injuries rather than due to their incomplete initial surgical repair.
Permissive hypotension
Aggressive crystalloid fluid resuscitation in patients who have sustained major trauma has been shown to exacerbate haemorrhage and worsen outcome. Increasing the arterial blood pressure through the administration of intravenous fluid risks clot disruption, resulting in further bleeding. In addition, haemodilution of clotting factors due to the administration of intravenous fluids will further worsen coagulopathy. Administration of large volumes of cold fluid will also worsen hypothermia. As such, current accepted military and civilian pre-hospital care practice is that fluid should be administered in 250-ml aliquots in order to maintain a palpable radial pulse (approximating a systolic arterial blood pressure of 80–90 mmHg). Hypotensive blood pressure targets for penetrating trauma have a good evidence base; however, for blunt trauma the evidence is limited. Evidence from animal models suggests that permissive hypotension can be reasonably maintained for up to 1 hour following injury, after which normotension should be targeted. Permissive hypotension is contraindicated in patients with head injuries and in those who are pregnant. Mean arterial pressure ≥80 mmHg should be maintained in patients with combined haemorrhagic shock and severe traumatic brain injury (TBI) who have a GCS ≤8.
Tranexamic acid
Following the results of the multi-national CRASH-2 trial published in 2013, it has become routine practice to administer tranexamic acid (TXA) to patients who have sustained major trauma. This study showed that in adult trauma patients who either had, or were at risk of, significant bleeding and within 8 hours of injury TXA (loading dose 1 g over 10 minutes then infusion of 1 g over 8 hours) significantly reduced both death due to bleeding and all-cause mortality at 28 days when compared to placebo. The study also found that the effect of TXA on death due to bleeding varied according to the time from injury to treatment with early treatment (≤1 hour from injury) conveying more favourable outcomes. In 2019 the CRASH-3 trial confirmed that TXA is safe in patients with TBI and that treatment within 3 hours of injury reduces head injury-related death in patients with a moderate TBI.
Transfusion strategies
The overarching aims of any transfusion strategy employed in the bleeding trauma patient are to minimize further blood loss while maximizing tissue oxygenation. Administering large volumes of fluid other than blood products has been shown to be cause clot disruption and haemodilution of clotting factors. There is also an association between large volume crystalloid fluid resuscitation and multiple organ failure in the critical care unit. Prior to obtaining haemorrhage control a systolic arterial blood pressure of 80–90 mmHg should be targeted, with normotension the goal once haemostasis is achieved. Once initial surgical control is obtained the patient may be normo- or even hypertensive due to high sympathetic tone. This can be cautiously obtunded by administering small aliquots of opiates, e.g. 0.5–1 μg/kg fentanyl, or by increasing the concentration of volatile anaesthetic agent. Blood products are then used to correct the resultant decrease in blood pressure, with the aim of restoring a normal circulating volume.
Much research has been dedicated to establishing the optimal ratio of blood products to administer to the bleeding trauma patient in the initial stages of resuscitation. Current evidence suggests that aiming for a 1:1:1 ratio of packed red cells (PRCs), fresh frozen plasma (FFP) and platelets is most effective in achieving haemostasis and reducing mortality. Within the UK this approach is advocated by a number of guidelines, including those published by the National Institute for Health and Care Excellence (NICE), the British Society of Haematology and the Association of Anaesthetists. Many institutions now utilize major/massive haemorrhage protocols, which once activated, generate a standardized response aimed at facilitating the rapid delivery of large numbers of appropriate blood products to patients while reducing the cognitive burden on the trauma resuscitation team.
Following the initial phase of resuscitation, further administration of blood products can be guided by coagulation studies, and viscoelastic tests such as thromboelastography (TEG) and rotational thromboelastography (ROTEM).
The use of fresh whole cold stored blood is being investigated as both a pre-hospital and early in-hospital method for replacing red cells, clotting factors and platelets.
Managing coagulopathy
A number of factors contribute to the development of coagulopathy in the patient who has sustained major trauma. Following the initial phase of resuscitation point-of-care coagulation monitoring using TEG or ROTEM to guide transfusion has been shown to reduce blood product administration and improve outcome. While they have limitations, conventional coagulation studies also provide useful information, and in many centres may be the only option available in the absence of point-of-care coagulation monitoring. Fibrinogen is one of the first clotting factors to fall and a low fibrinogen is associated with increased mortality. A fibrinogen 1.5–2 g/l have been suggested as the target for substitution with either cryoprecipitate or fibrinogen concentrate ( Table 1 ).

Full access? Get Clinical Tree
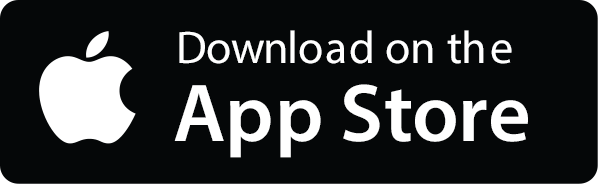
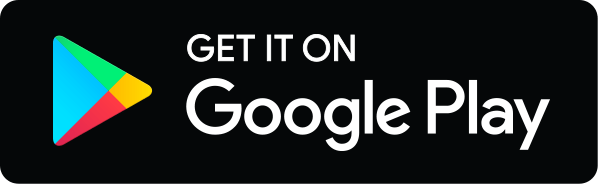