Management of Pain in the Critically Ill Patient
Armagan Dagal
Mario De Pinto
W. Thomas Edwards
Pain in critically ill patients should be systematically observed and regularly assessed. All means of analgesic interventions should be evaluated in a coordinated, individualized, and goal-oriented interdisciplinary manner.
Pain may stem from acute medical or surgical illness as well as preexisting medical conditions. Mechanical ventilation, placement of indwelling tubes and catheters, procedures performed such as placement of chest tubes, intracranial pressure (ICP) monitors, and turning and suctioning are also causes of pain [1,2]. Exposure to high levels of pain has negative psychologic and physiologic consequences, and its effective management is important in the maintenance of patient’s dignity [3,4,5].
Despite numerous improvement initiatives over the past two decades, pain is very common and often not treated appropriately in critically ill patients. It is estimated that as many as 70% of patients experience moderate-intensity procedure-related or postoperative pain during their stay in the hospital intensive care unit (ICU) [6,7,8,9]. Pain is frequently treated inappropriately because of fears of depressing spontaneous ventilation, inducing opioid dependence, and precipitating cardiovascular instability. Moreover, many clinicians often poorly understand the methods for assessing pain, the techniques for optimally treating it, and the benefits of its effective management. State-of-the-art pain management means not only decreasing pain intensity, but also reducing analgesics’ side effects, which may indeed facilitate patient recovery and is likely to shorten ICU and hospital stay [10,11,12]. Recent studies also suggest that effective acute pain management may help in reducing the development of chronic pain [13].
In 2005, the American Pain Society (APS) published the following guidelines for quality improvement in acute and cancer pain management [14]:
Recognize, identify, and treat pain promptly.
Involve patients and families in the pain management plan.
Improve treatment patterns.
Reassess and adjust the pain management plan as needed.
Monitor processes and outcomes of pain management.
The primary goal of this structured approach to pain management was to prevent pain through the administration of analgesics at regular intervals and before performing potentially painful procedures.
Implementation of the APS guidelines in 120 postcardiac surgery patients over a 3-month period revealed that 95% of them had effective pain relief during every ICU staff shift for the first 6 days after surgery [15]. Data also revealed dramatically improved side-effect profile and reduced length of hospital stay. Implementation of a similar pain management protocol in a medical ICU resulted in a decrease in ventilator days (from 10.3 to 8.9) and significant reductions of average hospital costs.
ICU pain management strategies may also incorporate the application of regional analgesia techniques (neuraxial and peripheral nerve blocks) when possible. Regional analgesia, when used appropriately, helps reduce the total amount of opioid analgesics necessary to achieve adequate pain control and the development of potentially dangerous side effects.
Evaluation of Pain
It is difficult to perform assessment of pain in the ICU. Structured approaches to pain assessment are mandatory for favorable patient outcome.
Pain assessment tools are useful to monitor for deterioration or improvements over time, and evaluate and titrate analgesic therapy appropriately [5,16].
There are several newly proposed methods available for pain assessment in the ICU. The chosen strategy should be adapted to the patient’s capacity to interact with the practitioner in order to provide assessment of static (rest) and dynamic pain (while moving the affected part or while taking deep breaths or coughing).
Assessment of pain should include determining cause, type, intensity, duration, site, and prior response to therapy. Categorization of pain into somatic, visceral, neuropathic in nature, or identification of specific sites, such as focal bone pain as opposed to allodynia or diffuse bowel distention, is important because it helps in determining the most effective type of intervention.
In general, appropriate assessment of pain improves the overall quality of pain management.
Subjective Pain Assessment
The Visual Analog Scale (VAS) is a 10-cm horizontal line, anchored by textual descriptors and/or pictures at each end. An end-point descriptor such as “no pain” (a score of 0) is marked at the left end and “worst pain imaginable” (a score of 10) is marked at the right end.
The Numerical Rating Scale (NRS) is a horizontal line with a scale from 0 to 10. Patients are asked to choose a number that relates to their pain intensity, where 0 represents no pain and 10 the worst imaginable pain. The NRS can be administered verbally or visually.
The Faces Pain Scale (FPS) was first developed by Wong and Baker and is recommended for those aged 3 and older. An explanation is given to the patient that each face is a person who feels happy because he or she has no pain or sad because he or she has some or a lot of pain. The patient is then asked to choose the face that best describes how they feel from six possible options.
It has been shown that the NRSs have the least variance and may be the preferred tool overall. Mechanically ventilated and sedated patients will be unable to use the VAS ruler or other self-report pain assessment tools. Once sedation has ceased, some patients may be alert enough to use a VAS ruler. This should be attempted as an option for these patients. If psychomotor abilities are impaired at this point, an NRS or FPS may prove to be more helpful.
Objective Pain Assessment
When the patient is critically ill, sedated, and/or ventilated, pain severity can be estimated only by observing the behavioral and physiologic responses to pain:
The Behavioral Pain Scale (BPS) is the earliest and most widely tested pain assessment tool for sedated patients. The BPS was developed by Payen et al. There are three component domains: “facial expression,” “upper limb movement,” and “compliance with ventilation.” Patients are scored from 1 to 4 on each section, giving a total score between 3 (no pain) and 12 (maximum pain) [17].
The Critical Care Pain Observation Tool (CPOT) was designed by Gelinas et al. The CPOT has four domains: “facial expression,” “body movement,” “muscle tension,” and “compliance with ventilation.” Patients are scored in each section between 0 and 2, giving an overall score of 0 (no pain) to 8 (maximum pain).
The Non-Verbal Pain Scale (NVPS) was developed by Odhner et al. The NVPS incorporates three behavioral domains and two physiologic domains. The behavioral domains are “face,” “activity (movement),” and “guarding.” The first physiologic domain considers vital signs and the second incorporates other physiologic indicators including skin color and temperature, perspiration, and pupillary changes. Again, specific descriptors are given to enable the assessors to rate a patient’s pain from 0 to 2 within each domain, giving a total pain score between 0 (no pain) and 10 (maximum pain).
None of these tools can be regarded as gold standard and they require further evaluation and research to investigate the impact of their use on pain management in clinical practice. Nonetheless, they offer a consistent and systematic approach that might improve pain management in ICUs.
Formulation of a Treatment Plan
It is important to understand the characteristics of the pathologic process responsible for pain in order to establish the most effective therapy.
Character and Site
Pain can be categorized as follows:
Nociceptive pain: It occurs in response to a noxious stimulus and continues only in the presence of a persistent noxious
stimulus. It is transmitted through nonmyelinated C-sensory fibers and small myelinated A-fibers via the dorsal root ganglion and spinothalamic pathways in the spinal cord to the thalamus, periaqueductal gray, and other centers in the brain [19]. Nociceptive pain is often dull, aching, sharp, or tender.
Somatic pain: It is due to nociceptive signals arising from the musculoskeletal system.
Visceral pain: It is due to a disease process or abnormal function of an internal organ or its covering (parietal pleura, pericardium, and peritoneum). It can be frequently associated with nausea, vomiting, sweating, and changes in heart rate and blood pressure.
Inflammatory pain occurs in response to tissue injury and the subsequent inflammatory reaction. In order to help healing of the injured body part, the sensory nervous system undergoes a profound change as a result; normally innocuous stimuli now produce pain, and responses to noxious stimuli are both exaggerated and prolonged [20]. This is secondary to plasticity in nociceptors and central nociceptive pathways [21,22]. Ablation of a specific set of nociceptor neurons, such as the one expressing the tetrodotoxin-resistant sodium channel Nav1.8, eliminates inflammatory pain, but leaves neuropathic pain intact, indicating a fundamental difference in the neuronal pathways responsible for these pain states [23,24].
Neuropathic pain: It can be burning, tingling, or electric in character. Patients with neuropathic pain may describe positive or negative neurologic phenomena. Positive phenomena include spontaneous pain (arising without stimulus) and evoked pains (abnormal response to stimulus). Negative phenomena include impaired sensation to touch or thermal stimuli. Neuropathic pain is initiated or caused by a primary lesion or dysfunction in the central or peripheral nervous system (CNS or PNS).
Central neuropathic pain most commonly results from spinal cord injury, stroke, or multiple sclerosis [25].
Peripheral neuropathic pain can be caused by [26] the following:
Trauma (e.g., complex regional pain syndrome (CRPS) and chronic postsurgical pain)
Infection (e.g., postherpetic neuralgia and HIV-induced neuropathy)
Ischemia (e.g., diabetic neuropathy and central “poststroke” pain)
Cancer (e.g., invasion and compression of peripheral nerve structures)
Chemically induced (e.g., chemotherapy-induced neuropathy)
Neural damage to either the PNS or the CNS provokes maladaptive responses in nociceptive pathways that drive spontaneous pain and sensory amplification. This maladaptive plasticity leads to persistent changes and, therefore, needs to be considered a disease state of the nervous system in its own right, independent of the etiologic factor(s) that triggered it. Studies suggest that peripheral and central sensitization mechanisms are also involved. In the PNS, they include altered gene expression and changes in ion channels that lead to ectopic activity. In the CNS, the regulation of many genes also changes. In addition, synaptic facilitation and loss of inhibition at multiple levels of the neuraxis produce central amplification. Neuronal cell death and aberrant synaptic connectivity provide the structural basis for persistently altered processing of both nociceptive and innocuous afferent input. Highly organized neuroimmunologic interactions as a result of neural damage play an important part in the development of persistent neuropathic pain. Genetically determined susceptibility is also likely to unveil the risk of developing neuropathic pain [24].
Hyperalgesia (the lowering of pain threshold and an increased response to noxious stimuli), allodynia (the evocation of pain by non-noxious stimuli), hyperpathia (explosive pains evoked in areas with an increased sensory threshold when the stimulus exceeds the threshold), dysesthesia (spontaneous or evoked unpleasant abnormal sensation), and paresthesia (spontaneous or evoked abnormal sensation) are typical elements of neuropathic pain.
Medical Management
Consequences of inadequate sedation and analgesia in the ICU may result in excessive pain and anxiety, agitation, self-removal of tubes and catheters, violence toward caregivers, myocardial ischemia, patient-ventilator asynchrony, hypoxemia, and pain-related immunosuppression. In contrast, excessive and/or prolonged sedation can lead to skin breakdown, nerve compression, delirium, unnecessary testing for altered mental status, prolonged mechanical ventilation and associated problems such as ventilator-associated pneumonia (VAP), and perhaps post-traumatic stress disorder (PTSD). Balanced treatment using both nonpharmacologic and pharmacologic methods are imperative for pain management in the ICU [27,28]. Improvement in quality of care results in a reduction of the time spent on mechanical ventilation and length of stay in the ICU.
Nonpharmacologic Treatments
Nonpharmacologic interventions are easy to provide, safe, and economical. They may include attention to proper positioning of patients to avoid pressure points, stabilization of fractures, and elimination of irritating physical stimulation (e.g., avoiding traction on the endotracheal tube).
Several mechanisms have been proposed to explain how to inhibit or modulate the ascending transmission of a noxious stimulus from the periphery or, conversely, to stimulate descending inhibitory control from the brain [29].
They include the following:
Gate control theory
“Busy-line” effect
Production of endogenous opioids at the periaqueductal gray, reticular activating system, and spinal gate
Activation of monoaminergic neurons in the thalamus, hypothalamus, and brain stem
Activation of second-order neurons in the dorsal horn, selective inhibition of abnormally hypersensitive neurons in the dorsal horn, and increased release of γ-aminobutyric acid (GABA) in spinal neurons
Descending inhibition from supraspinal centers via the pretectal zone and posterior columns
Stimulation-produced analgesia (SPA) is a term that describes noninvasive or minimally invasive techniques such as acupuncture, electroacupuncture (EA), transcutaneous electric nerve stimulation (TENS), acupressure and spinal cord stimulation (SCS), peripheral nerve stimulation (PNS), deep-brain stimulation, and motor cortex stimulation. Evidence suggests that these modalities are useful as a sole or supplementary analgesic technique for both acute and chronic painful conditions [29].
Peripherally applied heat causes local vasodilation that promotes circulatory removal of biomediators of pain from the site of injury, whereas cold application decreases the release of pain-inducing chemicals [30].
Modifications of the ICU environment, such as creating units with single rooms, decreasing noise, and providing music and appropriate lighting that better reflect a day–night
orientation [31], may help patients achieve normal sleep patterns and also improve pain control. For the cognitively intact ICU patients, provision of sensory and procedural information may improve their ability to cope with the discomfort.
orientation [31], may help patients achieve normal sleep patterns and also improve pain control. For the cognitively intact ICU patients, provision of sensory and procedural information may improve their ability to cope with the discomfort.
Pharmacologic Treatments
The pharmacologic characteristics of the ideal analgesic medication include easy titration, rapid onset and offset of action without accumulation, and no side effects.
Nonsteroidal Anti-Inflammatory Drugs
Cyclooxygenase (COX) is located in all cells. It metabolizes arachidonic acid to generate prostaglandin H2. A number of enzymes further modify this product to generate bioactive lipids (prostanoids) such as prostacyclin, thromboxane A2, and prostaglandins D2, E2, and F2. Three isoforms COX-1, COX-2, and COX-3 have been described. COX-1 is ubiquitous and constitutive. COX-2 is present in areas of inflammation and located in inflammatory cells. COX-3 is a splice variant, found centrally, and its inhibition is thought to be responsible for the action of acetaminophen [32].
It is now recognized that COX-2 is expressed in normal endothelial cells in response to shear stress and its inhibition is associated with suppression of prostacyclin synthesis. Inhibition of COX-2 results in prothrombotic inclination on endothelial surfaces and an increase in sodium and water retention, leading to edema, as well as exacerbations of heart failure and hypertension. Loss of the protective effects of COX-2 upregulation in the setting of myocardial ischemia and infarction leads to a larger infarct size, greater thinning of the left ventricular wall in the infarct zone, and an increased tendency to myocardial rupture [33,34].
Blockade of the proinflammatory mediators by nonsteroidal anti-inflammatory drugs (NSAIDs) reduces the inflammatory response (and subsequent pain). Classically, their effect is anti-inflammatory, analgesic, and antipyretic because of the direct inhibition of prostaglandin production. Adding NSAIDs to intravenous (IV) opioid-based patient-controlled analgesia (PCA) reduces opioid consumption by 30% to 50% and results in a significant reduction in the incidence of nausea, vomiting, and sedation [35].
On the other hand, the nonspecific blockade of COX inhibits the physiologic role of COX-1 and results in clinically significant deterioration of renal function and risk of development of peptic ulceration and upper gastrointestinal (GI) hemorrhage, bronchospasm, and platelet dysfunction. A meta-analysis published in 2002 showed that the risk of GI hemorrhage is related to the patient and drug-related factors, and is irrespective of the type of NSAID used. Patients who smoke, those with history of GI hemorrhage, and those taking anticoagulants are at increased risk [36].
Current evidence indicates that selective COX-2 inhibitors have important adverse cardiovascular effects that include increased risk for myocardial infarction, stroke, heart failure, and hypertension. The risk for these adverse effects is likely to be greatest in patients with a history of or at high risk for cardiovascular disease. In these patients, COX-2 inhibitors for pain relief should be used only if there are no alternatives and then only in the lowest dose and shortest duration necessary [37]. Currently, celecoxib is available for clinical use worldwide, whereas parecoxib is available only outside the United States.
Opioid-sparing properties of NSAIDs have not been studied in critically ill patients, so it is unclear if potential benefits outweigh potential risks such as GI bleeding or renal failure. Therefore, until more evidence for such agents becomes available, the clinician must carefully judge the risks and benefits on an individual basis.
Acetaminophen
Acetaminophen is an analgesic and antipyretic. It may also have anti-inflammatory properties. The mechanism of action of acetaminophen remains unknown. The greater sensitivity of cells containing COX-3 to acetaminophen is frequently cited as indicating that the target of action of acetaminophen is COX-3. Recent research indicates that acetaminophen inhibits prostaglandin synthesis in cells that have a low rate of synthesis and low levels of peroxide. When the levels of arachidonic acid are low, acetaminophen appears to be a selective COX-2 inhibitor. Acetaminophen has predominant effects on the CNS because the peroxide and arachidonic acid levels in the brain are lower than at peripheral sites of inflammation [38]. It is available in oral, rectal, and parenteral formulations. The parenteral formulation is not yet available in the United Sates although approval of the Food and Drug Administration (FDA) is pending. Acetaminophen is an effective adjuvant to opioid analgesia, and a reduction in opioid requirement by 20% to 30% can be achieved when combined with a regular regimen of oral or rectal acetaminophen.
It has been shown that 1 g of acetaminophen significantly reduces postoperative morphine consumption over a 6-hour period. Doses greater than 1,000 mg have been reported to have a superior effect when compared to lower doses. IV acetaminophen has been shown to reduce PCA morphine requirements after spinal surgery [39] and hip arthroplasty.
Its side-effect profile is comparable to placebo [40]; hypersensitivity reactions are rare. Major concerns with acetaminophen administration relate to the potential for hepatotoxicity, which, however, is extremely rare following therapeutic dosing [41]. In patients with severe liver disease, the elimination half-life can be prolonged. A reduced dose of 1 g three times a day with short duration of therapy is recommended. Prospective studies administering acetaminophen to patients consuming alcohol have found no increased evidence of liver injury [42]. In a recent study, nonallergic hypotension has been reported in a cohort of ICU patients on therapeutic doses of acetaminophen. The authors indicated brain injury and sepsis as the potential risk factors for this type of hypotensive reaction [43].
Opioids
For the critically ill patient, opioids remain the main pharmacologic method for the treatment of pain. Despite their extensive side-effect profile, there are no therapeutic alternatives available currently (Table 24.1).
Opiates refer to the nonpeptide synthetic morphine-like drugs while the term opioid is more generic, encompassing all substances that produce morphine-like actions. Opioids can be loosely divided into four groups:
Naturally occurring, endogenously produced opioid peptides (e.g., dynorphin and Met-enkephalin)
Opium alkaloids, such as morphine, purified from the poppy (Papaver somniferum)
Semisynthetic opioids (modifications to the natural morphine structure) such as diacetylmorphine (heroin), hydromorphone, oxycodone, and oxymorphone
Synthetic derivatives with structure unrelated to morphine, which include the phenylpiperidine series (e.g., pethidine and fentanyl), methadone series (e.g., methadone and dextropropoxyphene), benzomorphan series (e.g., pentazocine), and semisynthetic thebaine derivatives (e.g., etorphine and buprenorphine)
Table 24.1 Guidelines for Front-Loading Intravenous Analgesia | ||||||||||||||||||||||||||||||||
---|---|---|---|---|---|---|---|---|---|---|---|---|---|---|---|---|---|---|---|---|---|---|---|---|---|---|---|---|---|---|---|---|
|
Snyder et al. in 1973 reported on the presence of specific binding sites for opioids, providing the first evidence of distinct receptors for opioids. There are several types of opioid receptors. They differ in their potency, selective antagonism, and stereospecificity of opiate action. With a recent addition, the opioid receptor subtypes are listed as μ(MOP), κ(KOP) and δ(DOP) and nociception/orphanin FQ (N/OFQ) peptide receptor (NOP).
Opioids bind to the CNS and peripheral tissue receptors. μ1-Receptors mediate analgesia, whereas μ2-receptor binding produces respiratory depression, nausea, vomiting, constipation, and euphoria. κ-Receptor activation causes sedation, miosis, and spinal analgesia. In addition to analgesia, opioid receptors may provide mild-to-moderate anxiolysis. Opioids have no reliable amnestic effect on patients. Opioid administration is associated with a dose-dependent, centrally mediated respiratory depression. The respiratory rate is reduced, whereas the tidal volume is initially preserved. The ventilatory response to hypoxia is eradicated and the CO2–response curve is shifted to the right. Opioids facilitate patients’ compliance to the ventilator due to their cough-suppressant effects. Despite minimal cardiovascular effects in normovolemic patients, they may generate hypotension via decreased sympathetic tone, and thus may decrease heart rate and systemic vascular resistance in critically ill patients. Additionally, opioids increase venous capacitance, thereby decreasing venous return. Hypotension clearly is more pronounced in hypovolemic patients.
Opioid-induced ileus is a common problem in critically ill patients.
Morphine
Morphine has poor lipid solubility and thus has a relatively slow onset of action (5 to 10 minutes). The standard IV dose is 5 to 10 mg and the approximate half-life is 3 hours. However, with repeated dosing or continuous infusions, half-life kinetics become unreliable. Morphine is conjugated by the liver to metabolites that include morphine-6-glucuronide, a potent metabolite with 20 times the activity of morphine. Both morphine and morphine-6-glucuronide are eliminated by the kidney; therefore, renal dysfunction results in a prolonged drug effect.
Morphine may also cause hypotension due to vasodilatation (secondary to the release of histamine).
Fentanyl
Fentanyl is highly lipid soluble with rapid onset of action (1 minute) and rapid redistribution into peripheral tissues, resulting in a short half-life (0.5 to 1.0 hour) after a single dose. The duration of action with small doses (50 to 100 μg) is short as a result of redistribution from the brain to other tissues. Larger or repeated doses, including the doses delivered via a continuous infusion, alter the context-sensitive half-time and result in drug accumulation and prolonged effects of the drug. The hepatic metabolism of fentanyl creates inactive metabolites that are renally excreted, making this drug a more attractive choice in patients with renal insufficiency. Fentanyl causes minor hemodynamic changes and does not affect inotropy.
Hydromorphone
Hydromorphone is a semisynthetic opioid that is five- to tenfold more potent than morphine, but with a similar duration of action. It has minimal hemodynamic effects, lacks a clinically significant active metabolite, and causes minor to no histamine release [44]. Recently published data (Chang et al.) suggest
that patients who received IV hydromorphone have a greater decrease in pain than those given an equianalgesic dose of IV morphine [45].
that patients who received IV hydromorphone have a greater decrease in pain than those given an equianalgesic dose of IV morphine [45].
Methadone
Methadone is a synthetic opioid agent with properties similar to morphine. It can be given enterally and parenterally. Methadone is an attractive choice for opioid analgesia due to its long half-life and low cost. It produces N-methyl-D-aspartate (NMDA) antagonism, which makes it ideal for neuropathic pain. Although methadone is not the drug of choice for an acutely ill patient whose hospital course is rapidly changing, it is a good alternative for the patient who has preexisting opioid tolerance or prolonged ventilatory wean. It may help facilitate the tapering of opioid infusions [46,47]. Metabolized in the liver, 40% of the drug is eliminated from kidney and free from active metabolites. It does not accumulate in renal failure.
Oxycodone
Oxycodone is effective for postoperative pain management. It has a higher bioavailability and a slightly longer half-life than oral morphine. When transferring patients from parenteral morphine to oral oxycodone, the dose should be based on a 1:1.5 ratio (i.e., 1 mg IV morphine = 0.5 to 0.7 mg oral oxycodone). Individual patient variability and incomplete cross-tolerance requires careful titration [48].
The use of controlled-release oxycodone (OxyContin) is indicated for the treatment of moderate-to-severe pain when continuous analgesia is required for prolonged periods. The release of oxycodone from the OxyContin capsule is biphasic; there is a rapid initial absorption phase within 37 minutes followed by a slow absorption phase over 6.2 hours. Peak pain relief for OxyContin capsules occurs at approximately 1 hour and lasts for 12 hours, with peak plasma concentrations at 2 to 3 hours after administration.
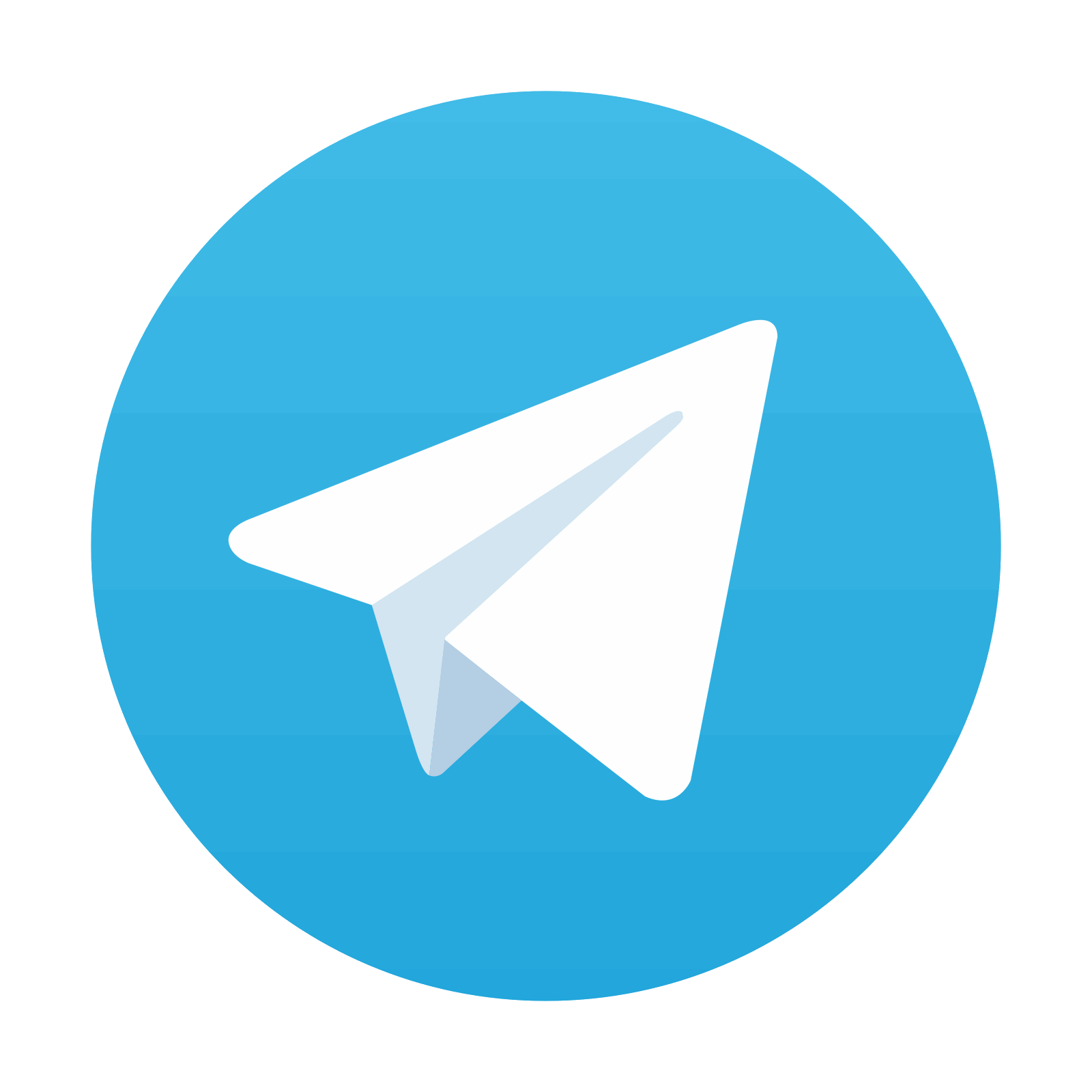
Stay updated, free articles. Join our Telegram channel

Full access? Get Clinical Tree
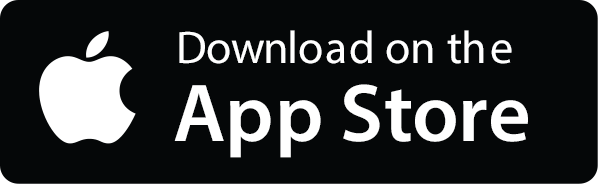
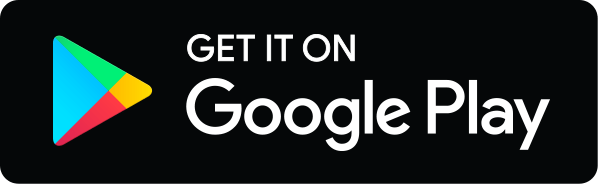