Malignant Hyperthermia
Tae W. Kim
Henry Rosenberg
▪ INTRODUCTION
Malignant hyperthermia (MH) is an inherited disorder that is manifested by exposure to potent volatile anesthetic agents and succinylcholine. The name was derived from early observations of patients who developed a rapid rise in body temperature during anesthesia. Early estimates of patient mortality associated with MH approached 80%. Fortunately, over the past half century, the mortality from MH has declined to less than 5%. The challenge for anesthesia providers and the anesthesia team is to recognize MH in its earliest stages and institute treatment promptly. Any delays in diagnosis or treatment have been shown to increase the risk of patient injury or even death. Patients with this condition are referred to as MH susceptible (MHS).
▪ EPIDEMIOLOGY
Cases of MH have been reported from almost every country and within most ethnic groups. Based on genetic studies, the prevalence of MH has been found to be as high as 1 in 3,000 patients. This means that 1 in every 3,000 individuals carries the genetic change that predisposes to MH. However, the incidence of MH events is much lower. Some studies report the incidence of MH at 1 in 5,000 anesthetics, while others estimate the incidence at 1 in 100,000 surgical procedures with a mortality of approximately 12%. Mortality rates may be higher in a subset of patients experiencing an MH event outside the hospital. Other risk factors that predict increased morbidity and mortality include patients with a muscular build and failure to detect the condition in a timely fashion. Based upon data from the North American Malignant Hyperthermia Registry and the Malignant Hyperthermia Association of the United States (MHAUS), about 1,000 cases of MH occur each year in the United States.
▪ PATHOPHYSIOLOGY
The underlying cause for MH susceptibility focuses on a channel in the muscle cell that regulates intracellular calcium release (called the ryanodine receptor) from the sarcoplasmic reticulum into the muscle cell cytoplasm (the myoplasm). The release of calcium leads to muscle contraction by promoting the muscle fibrils, actin and myosin, to interact and form bonds. Relaxation of muscles occurs by reversing this process with the uptake of calcium back into the sarcoplasmic reticulum. The process of contraction and relaxation of myofibrils requires energy. This energy is released through the breakdown of adenosine triphosphate (ATP). The energy that supports muscle contraction and relaxation also results in heat production. Under ordinary circumstances, the generation of heat is not significant.
In an MHS patient, the ryanodine receptor is abnormal due to a gene defect located on chromosome 19, which codes for the ryanodine receptor type 1. The resulting defective calcium channel allows for the uncontrolled release of calcium into the myoplasm. In addition, the cellular processes involved in sequestering the excess levels of myoplasmic calcium are quickly overwhelmed. The presence of excess calcium constantly stimulates muscle activity. This hypermetabolic state promotes consumption of all the available oxygen, nutrients, and energy (ATP). The exhaustion of substrates forces alternative pathways to sustain cellular activity, resulting in cellular damage and a mixed respiratory and metabolic acidosis.
Eventually, the cellular mechanisms that maintain homeostasis begin to fail and cellular
integrity becomes compromised. Potassium may leak from the cell into the bloodstream, leading to dangerously elevated levels. The weakening and eventual breakdown of muscle cells releases myoglobin into the bloodstream (rhabdomyolysis). The presence of brown or cola-colored urine may serve as a clinical indicator of free myoglobin in the blood that is toxic to the kidneys. A high urine output is required to avoid kidney failure. Hence, if the MH syndrome is not recognized and aggressively treated, the patient may suffer severe complications associated with high body temperatures, extreme acidosis, rhabdomyolysis, and elevated serum potassium, which may lead to arrhythmias and cardiac arrest. These changes may occur over a period of only 10 to 20 minutes.
integrity becomes compromised. Potassium may leak from the cell into the bloodstream, leading to dangerously elevated levels. The weakening and eventual breakdown of muscle cells releases myoglobin into the bloodstream (rhabdomyolysis). The presence of brown or cola-colored urine may serve as a clinical indicator of free myoglobin in the blood that is toxic to the kidneys. A high urine output is required to avoid kidney failure. Hence, if the MH syndrome is not recognized and aggressively treated, the patient may suffer severe complications associated with high body temperatures, extreme acidosis, rhabdomyolysis, and elevated serum potassium, which may lead to arrhythmias and cardiac arrest. These changes may occur over a period of only 10 to 20 minutes.
▪ CLINICAL PRESENTATION
The vast majority of patients who are MHS have no signs of being at risk in ordinary life. Some may, in retrospect, have significant muscle cramping or even have suffered an episode of heatstroke, but these are nonspecific signs. Patients should be questioned about any family history of any muscular disorders, since some disorders predispose to MH susceptibility. Also, questioning a patient about previous experience with anesthesia and determining whether there is a family history of any untoward event after anesthesia is important in raising suspicion that such a patient may be at risk for MH. In fact, over half of the patients who experienced MH had two or more general anesthetics prior to their first MH crisis.
The onset time of MH has been found to vary based on the choice of halogenated anesthetic agent and the use of succinylcholine. The quickest onset of MH was elicited by a combination of halothane and succinylcholine on induction of anesthesia. However, halothane is no longer available for human use in many countries. In addition, a black box warning of succinylcholine has been placed in the package insert for succinylcholine. Nevertheless, MH has been noted to occur with any of the potent volatile anesthetics (Table 63.1). The onset of MH has been reported to occur after many minutes or hours of anesthetic administration or even in the early recovery period.
The clinical presentation of MH under anesthesia may be very subtle or quite obvious (Table 63.2). One of the earliest signs of MH is tachycardia. However, the earliest specific sign is a rapidly rising end-tidal CO2 despite attempts at hyperventilation. This will manifest as increasing levels of carbon dioxide on the capnograph, which is unresponsive to changes in minute ventilation. This is due to the accelerated production of CO2 during the hypermetabolic phase.
TABLE 63.1 MALIGNANT HYPERTHERMIA TRIGGERING AGENTS | ||||||||||||||||
---|---|---|---|---|---|---|---|---|---|---|---|---|---|---|---|---|
|
Muscle rigidity may be present in over 50% of MH cases, if the syndrome is allowed to continue, and is considered pathognomonic for MH, when associated with an unresponsive increase in end-tidal CO2. The earliest sign of muscle rigidity may be masseter muscle rigidity (clenching of the jaw), if succinylcholine is used during the induction period to help secure the airway. The classic “jaws of steel” make it impossible to open the mouth for laryngoscopy or placement of an airway. This rigidity may last several minutes and is NOT responsive to other neuromuscular blocking agents such as
vecuronium or rocuronium. A rapidly rising body temperature usually follows the other signs described, but once temperature begins to rise, core temperature may increase 1°C-2°C every 5-10 minutes, particularly in muscular individuals.
vecuronium or rocuronium. A rapidly rising body temperature usually follows the other signs described, but once temperature begins to rise, core temperature may increase 1°C-2°C every 5-10 minutes, particularly in muscular individuals.
TABLE 63.2 CLINICAL PRESENTATION | ||||||||
---|---|---|---|---|---|---|---|---|
|
▪ LABORATORY FINDINGS
Elevated end-tidal carbon dioxide is an early sign of MH. Initially, respiratory acidosis predominates, then as the syndrome progresses, metabolic acidosis becomes more prominent. This should prompt the anesthesia provider when considering MH to obtain an arterial blood gas for confirmation of the presence or absence of a respiratory and/or metabolic event and its severity. Hypoxemia is usually NOT a problem, although in severe cases mild desaturation may be noted. Potassium levels should be assessed with each blood gas sample as life-threatening hyperkalemia may occur during an MH crisis. Creatine kinase (CK or creatine phosphokinase, CPK) is a marker of muscle damage with serum concentrations reflecting the extent of tissue injury. The CK levels should be monitored until the concentration decreases to within normal values. Serum myoglobin should also be assayed as well as urine myoglobin. Since testing for myoglobin levels may not be readily available or the results quickly obtained, another simple and rapid test for myoglobin can be performed by testing the patient’s urine sample for hemoglobin. The use of a hemoglobin urine test strip is based on the shared positive reaction to the presence of myoglobin in the urine. If positive and there are no red blood cells reported in the microscopic examination of the urine, then the test is presumed positive for the presence of myoglobin. Coagulation studies for disseminated intravascular coagulation should be ordered as well, especially in cases where the body temperature has risen to a critical temperature of >41.5°C. Testing for serum calcium levels is unnecessary, since serum concentrations are generally unchanged. After the acute episode is controlled, serial CK levels should be followed every 6-12 hours until the results plateau and begin to return to normal. If the syndrome continues, then blood gases and electrolytes need to be monitored at frequent intervals. In addition, body temperature should be continuously monitored.
▪ INTRAOPERATIVE MANAGEMENT
Successful management of an MH crisis requires a carefully coordinated plan involving many individuals, including operating room nurses, surgeons, anesthesia providers, anesthesia technicians, and a variety of ancillary personnel. Time and coordination are essential. Simulations of an MH crisis have repeatedly demonstrated that the anesthesia provider is rapidly overwhelmed with the number of tasks that must be accomplished. A well-trained anesthesia technician can be invaluable during an MH crisis.
Once the diagnosis of MH is made, the anesthesia provider will need to discontinue the anesthetic gas and begin hyperventilating the patient with 100% oxygen. If the surgery is not completed, the situation will require switching to an intravenous (IV) anesthetic technique. This will require multiple infusion pumps for the individual drugs. Increasing the fresh gas flow of oxygen to the highest level will help decrease the anesthetic gas concentration within the anesthesia machine, but significant amounts may still be present for 20 minutes and longer with the newer anesthesia machines. Although not recommended by the MHAUS, some practitioners prefer abandoning the contaminated anesthesia machine and switching to manual ventilation with a different oxygen source and bag-valve-mask resuscitator.
The most important task is to begin treatment with dantrolene, cooling devices, and medications as clinically indicated. The surgeon must also be notified to help coordinate a timely ending to the surgery. In addition, if not already being monitored, core temperature monitoring must be established: esophageal, rectal, bladder, and as a last resort axillary. Skin temperature is unreliable in an MH crisis. The declaration of an MH crisis should mobilize all available personnel, along with an MH cart containing dantrolene sodium, to the operating room. Dantrolene is the only antidote for treating MH. The drug is relatively insoluble and requires the aid of many individuals to dissolve the dantrolene and prepare it for injection. Dantrolene is packaged in a glass vial in a lyophilized form resembling an orange cake (Fig. 63.1). Each dantrolene vial requires 60 mL of bacteriostatic free sterile water for injection. Complete dissolution of dantrolene is visually confirmed by a clear rusty
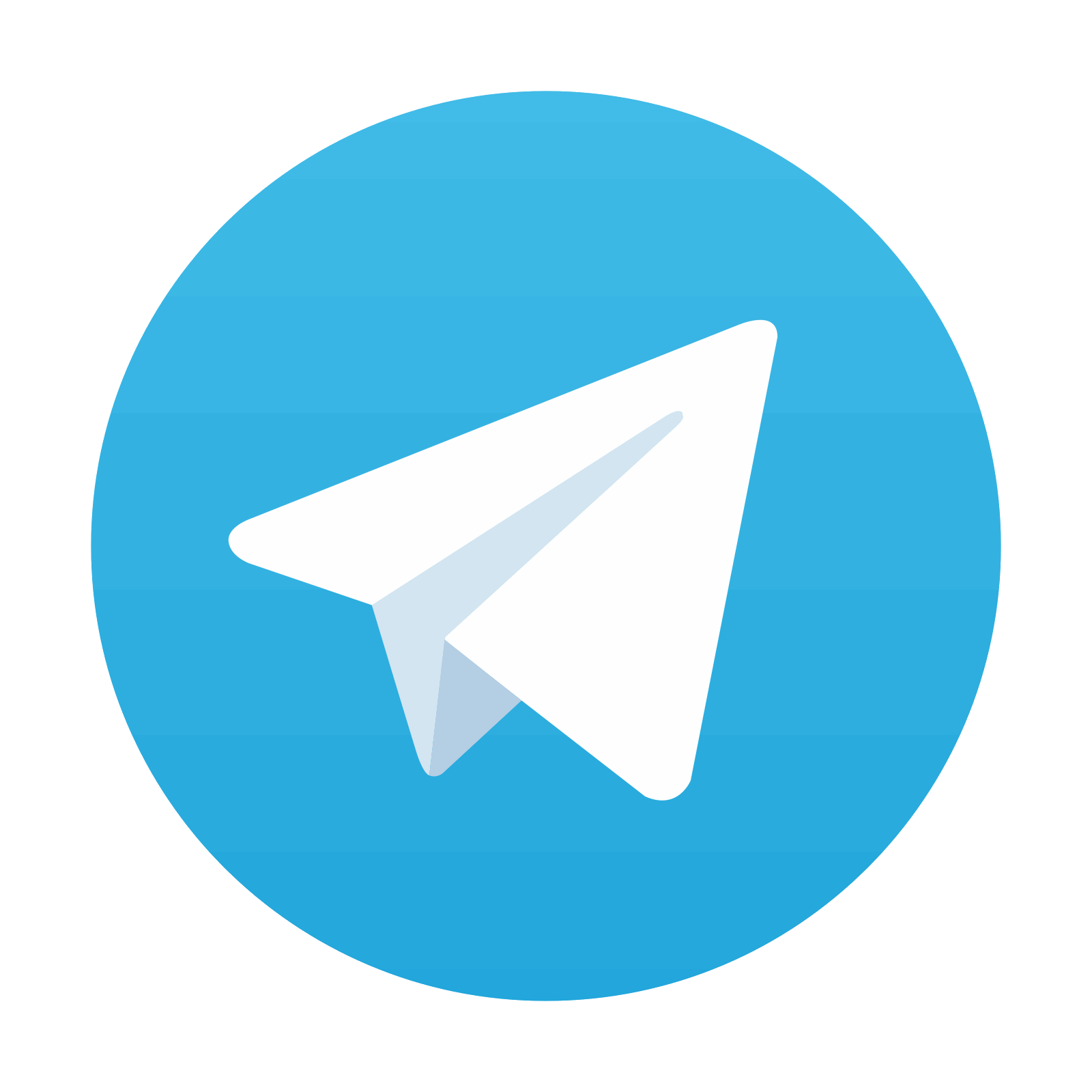
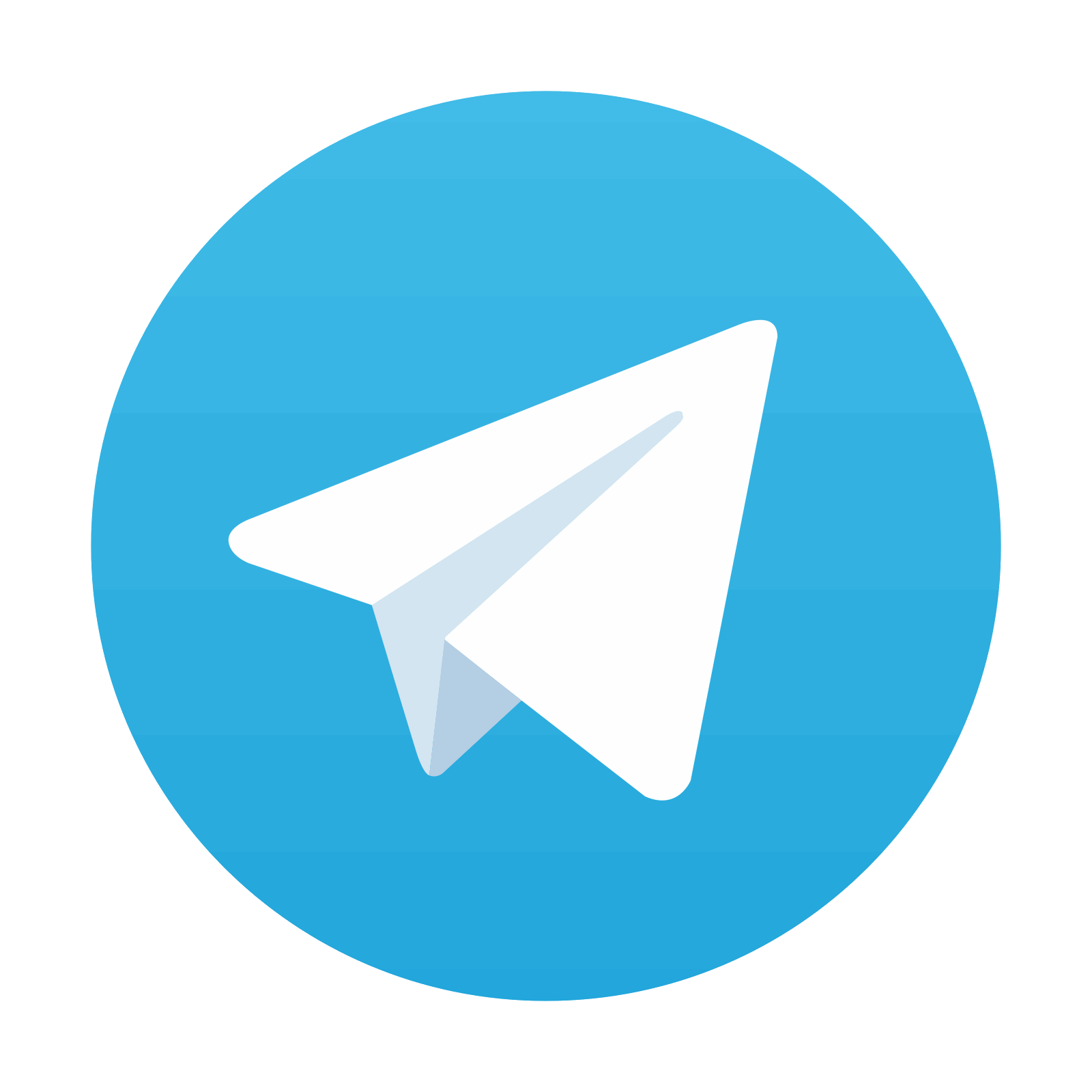
Stay updated, free articles. Join our Telegram channel

Full access? Get Clinical Tree
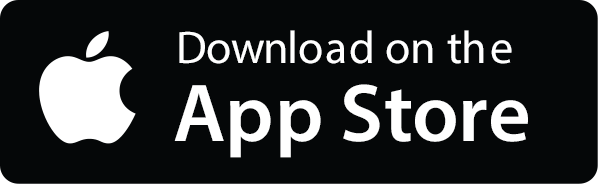
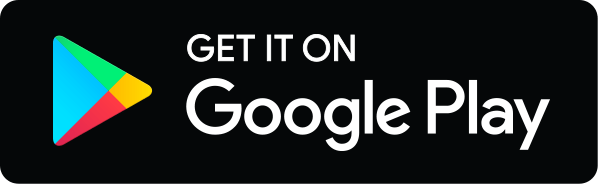