Lung Volume Reduction Surgery
Alina Nicoara
Joseph P. Mathew
Key Points
1. For the anesthesiologist, lung volume reduction surgery is a challenging procedure and the tailoring of the anesthetic management requires profound knowledge of the pathophysiology of COPD, ventilatory mechanics in awake and anesthetized COPD patients and pain management in thoracic surgery. A variety of different approaches to LVRS have been proposed; these include median sternotomy, thoracosternotomy, standard thoracotomy and video-assisted thoracosopic surgery (VATS) with both unilateral and bilateral approaches.
2. Potential candidates for LVRS undergo extensive evaluation in order to mitigate perioperative risks and contain perioperative complications. Important physiologic variables when evaluating a patient are FEV1 and DLCO, the RV/TLC ratio, PCO2 and oxygen use. The ideal operative candidate should have an FEV1 of 20% to 35% predicted without very severe reductions in DLCO (<20% predicted), a RV/TLV more than 0.67, a PCO2 less than 45 mm Hg, and no or low level supplemental oxygen use.
3. Intraoperative management is centered on minimizing further insult due to induction of general anesthesia and institution of positive pressure ventilation. Ventilatory management during one-lung ventilation (OLV) aims to balance competitive priorities: maintaining adequate oxygenation, minimizing intrinsic PEEP, minimizing barotrauma and maximizing CO2 elimination.
4. Intraoperative hypotension may be due to sympathetic blockade from local anesthetics administered through the thoracic epidural catheter, vasodilatory effects of the induction agents, hypovolemia, myocardial ischemia, dynamic hyperinflation or infrequent but possible catastrophic causes such as tension pneumothorax.
5. Tracheal extubation immediately after surgery is an important aim after LVRS in order to minimize the risk of developing or exacerbating an air leak and avoid the deleterious hemodynamic effects of positive pressure ventilation. Adequate pain control achieved with minimal respiratory depression in LVRS patients is vital to the success of the surgical procedure. Inadequate pain control will result in splinting, poor respiratory effort, and inability to cough and clear secretions leading to airway closure, atelectasis, shunting and hypoxemia.
The patient is a 68-year-old male with advanced emphysema who is scheduled for lung volume reduction surgery (LVRS). He is an ex-smoker who has recently undergone preoperative pulmonary rehabilitation. He has concurrent coronary artery disease and hypertension. Medications include aspirin, valsartan and lovastatin.
Vital signs: BP 140/70, HR 72, and room air oxygen saturation 91%.
Laboratory examination is notable for blood urea nitrogen of 30 mg/dL and creatinine of 1.9 mg/dL. Pulmonary function tests reveal a FEV1 of 0.9 L (30% predicted), FVC of 3.6 L (50% predicted), TLC 7.1L (110% predicted) and DLCO 22% predicted.
Chronic obstructive pulmonary disease (COPD) is characterized by progressive and largely irreversible airflow limitation caused primarily by exposure to tobacco smoke, and less commonly by other noxious stimuli or by alpha1-antitrypsin deficiency. COPD is one of the leading causes of death and disability worldwide. It is expected that by the year 2020, COPD will become the third leading cause of death worldwide.1
The main goals of therapy in COPD patients are focused on relieving symptoms, preventing lung function decline, preventing exacerbations of the disease, and improving exercise capacity and quality of life. In spite of the advances in medical therapy, smoking cessation is the single most effective intervention shown to alter the rate of progression of COPD.2 Supplemental oxygen therapy in patients with severe COPD and hypoxemia has also been shown to have beneficial effects such as prolonged survival, improvement in cardiac function, and improved exercise tolerance.3
The failure of medical management of COPD to produce significant impact on outcomes has led to the development of lung volume reduction surgery (LVRS) in the past century. In selected patients with severe emphysema, LVRS was associated with prolonged survival, improvement in exercise capacity and quality of life, and improvement in lung function and dyspnea.4 Given the high perioperative mortality and morbidity associated with LVRS, the survival benefit depends on adequate patient selection and containment of perioperative complications associated with anesthesia and surgery.
For the anesthesiologist, lung volume reduction surgery is a challenging procedure and the tailoring of the anesthetic management requires profound knowledge of the pathophysiology of COPD, ventilatory mechanics in awake and anesthetized COPD patients and pain management in thoracic surgery. This chapter summarizes the impact of anesthesia and surgery on lung function in COPD patients, preoperative evaluation and criteria for optimal patient selection and intraoperative and postoperative anesthetic management.
PATHOPHYSIOLOGY OF COPD
The cardinal abnormality in COPD is an irreversible reduction in maximal expiratory flow due to chronic bronchitis, emphysema, or both. Chronic bronchitis is defined as cough and sputum production for most days over 3 months for 2 consecutive years. It is thought to result from the innate immune response to inhaled toxic particles and gases, particularly to tobacco smoke, which results in inflammation of the epithelium of the central airways and mucus-producing glands. The airway inflammation is associated with increased mucus production, reduced mucociliary clearance, and increased permeability of the airspace epithelial barrier.5 While hyperproduction of mucus may not have a significant impact on smokers with normal lung function, in patients with severe COPD it contributes to the further decline of airflow during expiration.
Emphysema is defined as enlargement of the airspaces distal to the terminal bronchioles due to destruction of the alveolar walls. Destruction of the normal lung parenchyma causes a reduction of the elastic recoil resulting in a progressive deterioration of the maximal expiratory airflow. Emphysema may exist in a centrilobular or a panlobular form. The centrilobular (also known as centriacinar) form results from dilatation or destruction of the respiratory bronchioles, is more closely associated with tobacco smoking and has predominantly an upper lobe distribution.6 The panlobular (also known as panacinar) form, results in more even dilatation and destruction of the entire acinus, is associated with alpha1-antitrypsin deficiency and has predominantly a lower lobe distribution (Figures 15–1, 15–2, 15–3).
Figure 15–1. A chest radiograph of a patient with diffuse severe emphysema.
Figure 15–2. A chest radiograph of a patient with severe emphysema and upper lobe predominance.
Figure 15–3. A computed tomograph of a patient with pan-lobular emphysema left greater than right.
On pulmonary function testing, the expiratory airflow limitation is first identified as a reduction in the ratio of forced expiratory volume in 1 second (FEV1) to forced vital capacity (FVC). The diagnosis of COPD as established by the Global Initiative for Chronic Obstructive Lung Disease (GOLD) requires an FEV1/FVC ratio of less than 0.7. Patients are then stratified into four categories from mild to very severe disease based on the severity of FEV1 impairment7 (Table 15–1).
Table 15–1. Physiologic Classification of the Severity of Chronic Obstructive Pulmonary Disease*
Expiratory airflow limitation results in air trapping and hyperinflation with increases in total lung capacity (TLC) and residual volume (RV), and an elevated RV/TLC ratio. As the disease progresses and the recoil of the lungs becomes diminished, the functional residual capacity (FRC) moves rightwards toward the flat portion of the compliance curve of the lungs, to the detriment of pulmonary mechanics. Therefore, the patient with hyperinflation has difficulty with inspiration because the respiratory system moves on a relatively flat portion of its compliance curve and consequently there is increased elastic work of breathing. As COPD progresses, static hyperinflation gets progressively worse, and the operational lung volumes increase toward a threshold that may even result in resting dyspnea.8 Further elevation in operational lung volumes, superimposed on static hyperinflation occurs during exercise when ventilatory requirements are increased. Due to airflow obstruction and shortened expiratory time during exercise, expiration cannot be completed and inhalation is triggered due to the urge to inspire before FRC is reached. This respiratory pattern leads to “stacking” of breaths and a gradual hyperinflation of the lungs, known as dynamic hyperinflation. Similar exacerbations of hyperinflation occur with increased respiratory rate associated with anxiety and hypoxemia.
The loss of acinar structure and disruption of the alveolar-capillary structure lead to ventilation-perfusion (V/Q) mismatch and impairment of gas exchange. Also, as the hyperinflation develops nonuniformly, the normal parenchyma may become compressed and underexpanded, causing a further increase in the V/Q mismatch. Due to the higher diffusability of carbon dioxide (CO2), the CO2 elimination is well-preserved until V/Q abnormalities are severe. As the disease advances, there is an increase in physiologic dead-space secondary to under-perfused alveoli, and the subsequent impairment of CO2 clearance results in hypercapnic respiratory failure. Also, air trapping and hyperinflation place the diaphragm and other inspiratory muscles at severe mechanical disadvantage, producing alveolar hypoventilation and contributing to hypercapnia. Chronic CO2 retention occurs slowly and a compensated respiratory acidosis is noted on arterial blood gas analysis. Acute CO2 retention, however, is a sign of impending respiratory failure and occurs with superimposed respiratory infection and bronchospasm.
Pulmonary hypertension is a frequent complication in the natural history of COPD. It progresses over time and its severity correlates with the degree of airflow obstruction and the impairment of pulmonary gas exchange. However, the rate of progression is slow and the degree of pulmonary hypertension in COPD is of low-to-moderate magnitude, rarely exceeding 35 to 40 mm Hg9 of mean pulmonary artery pressure. Usually right ventricular function is mildly impaired with preservation of cardiac output. Acute exacerbations in right ventricular afterload in the settings of hypoxia, hypercapnia, or compression of intraalveolar vessels due to dynamic hyperinflation may result in right ventricular failure. Elevated mean alveolar pressure associated with dynamic hyperinflation may compress intra-alveolar vessels, increasing pulmonary vascular resistances and right ventricular output impedance. With progressive right ventricular dysfunction, left shifting of the interventricular septum can impair cardiac output further due to ventricular interdependence.10 Patients with severe COPD and pulmonary hypertension show structural and functional changes in pulmonary muscular arteries and precapillary vessels that explain the irreversible increase of pulmonary vascular resistance. Long-term oxygen therapy may slow down the progression of pulmonary hypertension, however, pulmonary artery pressures rarely return to normal and the structural abnormalities of the pulmonary vessels remain unaltered.9
COPD is also associated with high rates of other comorbid illnesses such as cardiovascular disease, malnutrition, peripheral muscle weakness and osteoporosis. Cardiovascular disease is a major cause of death in patients with COPD. There are several reasons for the association between COPD and cardiovascular disease including a major shared risk factor (smoking), use of beta-agonist medications that may stimulate the cardiovascular system, and systemic inflammation.11,12 Enhanced systemic inflammation may also explain nonpulmonary COPD characteristics such as skeletal muscle dysfunction, cachexia, and malnutrition.8
SURGICAL TECHNIQUES FOR LUNG VOLUME REDUCTION SURGERY
Brantigan developed the concept of LVRS in the 1950s when he described resection of 30% of the hyperinflated lung and autonomic denervation through thoracotomy in an attempt to improve expiratory flow.13 LVRS was not performed widely as it was associated with a very high surgical mortality at that time. In 1995, Cooper and colleagues reported dramatic improvements in pulmonary function and no mortality in 20 patients undergoing simultaneous bilateral LVRS using a median sternotomy with resection of 30% of each lung.14
Surgical Approach
A variety of different approaches to LVRS have been proposed; these include median sternotomy, thoracosternotomy, standard thoracotomy, and video-assisted thoracosopic surgery (VATS) with both unilateral and bilateral approaches (Figure 15–4). The areas for surgical removal are identified before surgery by computed tomography and radionuclide ventilation-perfusion scanning. Methods for sealing the site of resected lung include the use of staples or laser (neodymium-yttrium aluminum garnet or Nd-YAG).15
Figure 15–4. A chest radiograph (A) and computed tomograph (B) of a patient with a left giant bulla for unilateral lung reduction.
MEDIAN STERNOTOMY VS VATS
Questions regarding the high perioperative mortality in LVRS patients shown in an analysis of the Medicare claims data (23% at 1 year)16 and the cost effectiveness and high rehospitalization rates associated with LVRS led to the funding of the National Emphysema Treatment Trial (NETT), the largest randomized trial of LVRS performed to date. The NETT was designed to compare short- and long-term outcomes of best medical therapy for emphysema with best medical therapy plus LVRS. It also included randomized and nonrandomized comparisons of the median sternotomy and VATS for LVRS. This subanalysis showed that functional results as well as morbidity and mortality were comparable for LVRS by VATS or median sternotomy. The VATS approach, however, allowed earlier recovery at a lower cost than median sternotomy.17 Most studies comparing median sternotomy and VATS have yielded conflicting results. Wisser et al compared median sternotomy versus bilateral VATS in a sequential, nonrandomized study and showed similar postoperative morbidity and mortality and no difference in functional and physiologic results.18 In contrast, Roberts et al compared the complications associated with bilateral LVRS through VATS or median sternotomy. They found that although the operating time was greater for the VATS group, the median sternotomy group had a higher incidence of life-threatening complications, longer stay in the intensive care unit, ventilator days, and percent requiring reintubation.19 In a study predating the NETT, Kotloff et al compared the short-term outcomes following bilateral LVRS performed through median sternotomy (80 patients) and VATS (40 patients). All patients in both groups were extubated at the completion of surgery, but 17.5% of patients in the median sternotomy group and 2.5% in the VATS group subsequently required reintubation at some point during the postoperative course. There was no significant difference in duration of air leaks or length of hospital stay and the functional outcomes achieved with either technique were similar. Thirty-day operative mortality was 4.2% for the median sternotomy group and 2.5% for the VATS group. However, total in-hospital mortality was 13.8% for the median sternotomy group, while it remained 2.5% for the VATS group.20
UNILATERAL VS BILATERAL
Bilateral VATS for lung volume reduction is preferred over the unilateral approach. Multiple studies have shown that the bilateral procedure produces greater overall improvement than a unilateral procedure.21,22 McKenna et al compared unilateral and bilateral VATS procedures and showed that the bilateral procedure provided greater oxygen independence (68% vs 35%), prednisone independence (86% vs 56%), greater improvement in the FEV1 and an improvement in the perceived degree of dyspnea than the unilateral procedure with comparable mortality and morbidity.22 However, given the improvement associated with unilateral surgery, unilateral operation may still be offered in some patients who are not candidates for a bilateral operation due to prior thoracic intervention or inappropriate anatomy for bilateral LVR. Similar functional outcome with sustained improvement of FEV1, 6-minute walk test and gas exchange was shown in other studies.23,24 The excellent results seen in the postoperative period with the use of bilateral LVRS may not lead to improved long-term survival. A large multi-institutional retrospective study comparing long-term survival in patients undergoing either bilateral or unilateral thoracoscopic lung volume reduction found that there was no significant difference between the two groups in regard to operative mortality or late death at 1 year, 2 years, or 3 years.25
STAPLES VS LASER
McKenna et al also showed in a prospective blindly randomized study that patients in whom lung volume reduction surgery is done with staples and buttressing with bovine pericardium have a lower morbidity (fewer delayed pneumothoraces), as well as greater improvement in oxygen independence, lung function, and overall lifestyle and dyspnea scale, than patients treated with contact laser (Nd-YAG).26
POSITIONING
Usually, after induction of anesthesia and intubation, the patient is positioned supine with the arms supported above the head utilizing an ether screen. This position offers satisfactory exposure not only for VATS but median sternotomy, as well as anterior/lateral thoracotomy on both sides.27 For the VATS approach three ports are used on each side, two in the submammary crease and one axillary port. A horseshoe-shaped specimen is removed from the superior portion of the lung avoiding direct tissue handling in order to prevent damage to friable lung tissue with its attendant risk of postoperative air leak.27
Mechanisms for Improvement in Respiratory Function after LVRS
The possible mechanisms by which LVRS might provide benefit are not known with certainty. Numerous studies are now available regarding the short-term results of LVRS on pulmonary function, however the results are sometimes conflicting and cover a large range of reported values due to difference in study design, patient selection, surgical technique, presence or absence of pulmonary rehabilitation or pulmonary bronchodilator administration at the time of measurement.
As mentioned above, LVRS results in marked improvement in several parameters characterizing lung function. An overview of the results available in the literature shows that in the majority of patients, LVRS leads to an improvement of FEV1 accompanied by an increase in the FVC. Moreover, there is a decrease in total lung capacity (TLC) and residual volume (RV).20,22–24,26,28–31 The improvement in FEV1 is possibly due to an improvement in elastic recoil, a reduction in expiratory flow limitation and a decrease in dynamic hyperinflation shown by many investigators.29,32,33 Resection of the emphysematous, nonfunctional lung tissue should also allow the healthier lung tissue to expand and improve expiratory flow by reducing lung compliance, premature airway closure and end-expiratory volume.34 However, despite improvements in FEV1, postoperative changes in the FEV1/FVC ratio are very small or absent, suggesting that FEV1 increases not only because of an improvement of the expiratory flow but also because of the increase in FVC.34
The reports on the changes in resting arterial blood gases are less consistent, ranging from improvements in arterial partial pressure of oxygen (PaO2) and decreases in arterial partial pressure of carbon dioxide (PaCO2) to little change28 or even worsening of these parameters.35 While some studies link the change in oxygenation to an increase in mean alveolar ventilation,31,36 other studies suggest that the improved oxygenation is due to a reduction in ventilation-perfusion heterogeneity.35 In a follow-up study on a large cohort of subjects enrolled in the NETT, LVRS was found to increase PaO2 and decrease self-reported oxygen use at rest and on exertion.37 Few studies have reported the impact of LVRS on lung diffusing capacity for carbon monoxide (DLCO), however the improvement in DLCO shown appears to be modest.30,38
Emphysema leads to an increase in the lung volume at which the respiratory muscles operate, which reduces their mechanical effectiveness and leads to diminished inspiratory muscle force. Due to hyperinflation, muscle fibers have shortened inspiratory muscle pre-contraction length, which diminishes the pressure generated at a given level of tension.34 Also in patients with chronic inflation, the zone of diaphragmatic apposition, which is the area of the diaphragm immediately apposed to the ribcage, is markedly reduced32 potentially reducing the ability of the diaphragm to generate adequate inspiratory volume change. Numerous studies have shown an improvement in the respiratory muscle strength and interaction after LVRS as shown in an increase of the diaphragm length,39 increase in the maximal transdiaphragmatic pressures,39,40 reduction in maximal inspiratory pressure and reduction in dyspnea.40
The reported changes in pulmonary vascular function after LVRS are inconsistent, with some studies showing no change or improvement in pulmonary artery pressures and right ventricular function, and others showing worsening of pulmonary hypertension.33,41,42 These controversial results are due to possible opposing effects of LVRS on the pulmonary vascular function. Resection of perfused lung can increase the already impaired pulmonary vascular resistance while on the other hand a decrease in vascular resistance might occur through recruitment of vessels in the reexpanding lung tissue or through improvement in elastic recoil, which may increase radial traction on extra-alveolar vessels.43
The multiple effects of lung volume reduction surgery are summarized in Table 15–2.
Table 15–2. Physiological Effects of Lung Volume Reduction Surgery
Outcome and Complications
LVRS is associated with higher but acceptable mortality and morbidity than other general thoracic surgical procedures. Awareness and understanding of the potential complications associated with LVRS are some of the keys to minimizing their occurrence.44 Mortality rates associated with LVRS reported in the literature range from 2.5% to 13%.18,25,31,45 The 90-day mortality rate for LVRS in the NETT for the surgical group overall was 7.9%, much less than the mortality rate reported by Medicare.
Pulmonary complications are the most frequent after LVRS. Pneumonia occurs in about 7% to 14% of the patients. Respiratory failure requiring reintubation occurs in 5% to 10% of the patients, and if postoperative reintubation occurs, more than half of the patients will fail to wean from the ventilator.44 However, the most common complication after LVRS is prolonged air leak, defined here as an air leak that lasts at least 7 days following the procedure. This complication occurs in approximately 40% to 60% of patients and is associated with a more protracted and prolonged hospital stay. There does not seem to be any significant difference in the incidence of air leak after VATS and median sternotomy procedures.44 Within the NETT, an air leak occurred at some point in 90% of bilateral LVRS patients; the median duration of an air leak was 7 days, and 12% had a persistent air leak even 30 days postoperatively.46 Risk factors for post-LVRS air leak include Caucasian race, lower FEV1 or diffusion capacity, use of inhaled steroids, upper-lobe predominant emphysema and presence of moderate to marked pleural adhesions. Factors such as surgical approach or use of buttressing material at the staple line did not affect the presence or duration of air leak46 (Figure 15–5).
Figure 15–5. A patient with pneumonia and subcutaneous emphysema due to a postsurgical air leak after unilateral lung reduction.
Cardiac complications are the second most common cause of perioperative morbidity and mortality after lung resection surgery. Major cardiac morbidity, defined as intraoperative or postoperative arrhythmia requiring treatment, myocardial infarction or pulmonary embolus in the 30 days after lung volume reduction surgery, had an incidence of 20% in the NETT.47 The most common cardiac complication is arrhythmia-requiring treatment. Atrial arrhythmias can occur in up to 20% of the patients and are associated with fluid overload, atelectasis and hypoxia.44
Bronchoscopic Lung Volume Reduction
Given the potential benefit of LVRS on quality of life, survival and exercise capacity in selected patients with heterogeneous emphysema, several minimally invasive techniques have emerged in order to achieve lung volume reduction without open thoracotomy. Bronchoscopic lung volume reduction (BLVR) allows clinicians to collapse areas of severe emphysema. Several BLVR systems have emerged and are currently under clinical trials. Endobronchial one-way valve systems are deployed into segmental or subsegmental bronchi of emphysematous, hyperinflated lung. They are designed to prevent inspiratory airflow but to allow air and secretions to move from the alveoli to the central airways, therefore resulting in progressive deflation and collapse of the lung distal to the valve.48,49 Complications include migration of the device, hemoptysis, pneumothorax, postobstructive pneumonia, and failure of the lung to collapse due to collateral ventilation.
The Endobronchial Valve for Emphysema Palliation Trial (VENT) is the first prospective randomized multicenter trial to evaluate endobronchial valves. The preliminary results showed that the procedure has an acceptable safety profile.34 The study showed that endobronchial-valve treatment induced modest improvements in lung function, exercise tolerance, and symptoms at the cost of more frequent exacerbations of COPD, pneumonia, and hemoptysis after implantation.50
The Exhale Airway Stents for Emphysema (EASE) trial, a double-blinded, randomized, sham-controlled device study for patients with emphysema/COPD was designed to evaluate the safety and effectiveness of the Airway Bypass procedure with Exhale Drug-Eluting Stents. The prospectively defined clinical endpoints of the trial were FVC and the modified Medical Research Council (mMRC) dyspnea score, a measure of the impact of breathlessness on quality of life. Early results showed statistically significant improvement only in mMRC dyspnea score.51
Fibrin-based glue, with subsequent collapse and remodeling of emphysematous lung, can also achieve occlusion of airways. In an open-label, phase II study of 50 patients with advanced upper lobe emphysema, a fibrinogen-thrombin hydrogel was administered to eight subsegmental sites (four in each upper lobe). Significant improvement was noted in the primary outcome, residual volume to total lung capacity ratio (RV/TLC), and also in secondary outcomes such as improvement in FEV1, symptom scores, and health-related quality of life.52
PREOPERATIVE ASSESSMENT AND PATIENT SELECTION
Potential candidates for LVRS undergo extensive evaluation in order to mitigate perioperative risks and contain perioperative complications. The patient selection criteria are rigorous, involving both functional and radiological assessment with the goals of identifying the patients that are most likely to benefit from the procedure with an acceptable risk, optimizing medical status and customizing perioperative risk reduction strategies. The criteria for lung resection in patients with emphysema have developed over time. Before the NETT there was a high variability in the reported results due to differences in patient population, study design, surgical technique and perioperative management. The NETT, a multi-institutional randomized study sponsored jointly by the National heart, Lung and Blood Institute and the Center for Medicare and Medicaid Services, provided reliable estimates of risk and benefit from LVRS and established criteria selection for patients who will benefit most from lung volume reduction.
Clinical Evaluation
The clinical evaluation of the patients should be focused on identifying the clinical manifestations of the lung disease, presence of comorbid conditions, active cigarette smoking, corticosteroid use, and nutritional status. Medical contraindications include any conditions that increase the perioperative risk or predict a short-life expectancy due to non-emphysema illnesses. Advanced age has also been suggested as a risk factor for an unacceptable outcome.30,53 Previous studies of the LVRS population showed that patients aged 70 years or older are at risk for an increased perioperative morbidity and mortality53 or for less postoperative improvement in lung function.54 Although the NETT did not identify age as a prognostic factor for operative mortality, patients with advanced age were at higher risk for major pulmonary morbidity and cardiovascular complications.47
Based on mechanisms of lung function improvement after LVRS, it is expected that the patients that benefit most have clinical manifestations of lung disease consistent with parenchymal destruction typical for emphysema with mild or minimal airway disease. Therefore, a history of recurrent bronchial infection with clinically significant daily sputum production might identify patients with primarily intrinsic disease who would not benefit from lung volume reduction. Prior thoracic surgery, which might lead to the formation of pleural adhesions and pleural or interstitial disease, are viewed as exclusion criteria for LVRS.
Careful assessment for cardiac disease before selection for surgery is also necessary as LVRS carries a significant risk of cardiac stress and intraoperative myocar-dial infarctions have been reported.55,56 Coronary artery disease (CAD) is frequent among patients with COPD, as the two diseases share cigarette smoking as a common risk factor and a high prevalence of CAD has been shown angiographically in the LVRS patient population.57 The evaluation of patients with advanced emphysema for the presence and extent of coronary artery disease presents a clinical challenge, as these patients may have poor functional capacity due to the underlying lung disease. However, keeping in mind that elective pulmonary resection surgery is deemed an “intermediate risk” procedure by the American College of Cardiology (ACC)/American Heart Association (AHA) guidelines on perioperative cardiovascular examination for noncardiac surgery,58 the medical consultant needs to assess the functional status, severity, and stability of cardiac symptoms in a patient who has CAD or heart failure and proceed with noninvasive testing whenever indicated. Patients who have evidence of significant ischemia on noninvasive testing usually undergo coronary angiography; those who have left main or triple-vessel disease are potential candidates for surgical revascularization, whereas those who have single-or double-vessel disease may undergo PCI with or without stenting or be managed medically. Successful LVRS and coronary artery bypass grafting or LVRS and valve replacement have been performed with improvements in pulmonary function similar to those reported after isolated LVRS procedures.59–62 A recent myocardial infarction (within the past 30 days) represents a major risk factor for perioperative risk complications. Although in the past it was believed that elective surgery should be delayed for 6 months after a myocardial infarction based on Goldman’s original risk index, the ACC/AHA guidelines have decreased this interval to 4 to 6 weeks for a medically stable, fully investigated and optimized patient.58,63
Another issue with potential perioperative implications is pulmonary hypertension. The degree of pulmonary hypertension in COPD is usually of low to moderate magnitude, with mean pulmonary artery pressures rarely exceeding 35 to 40 mm Hg,9 and although right ventricular function is generally preserved, multiple perioperative clinical scenarios (hypoxia, hypercapnia, dynamic hyperinflation) can lead to right ventricular failure. It is accepted that a peak systolic pulmonary artery pressure more than 45 mm Hg or mean pulmonary artery pressure more than 35 mm Hg is a relative contraindication to LVRS.64 As echocardiography is frequently inaccurate in patients with advanced lung disease and tends to considerably overestimate the degree of pulmonary hypertension65 a right heart catheterization is sometimes required in order to rule out significant pulmonary hypertension.
As smoking cessation has a significant positive impact on the rate of decline of FEV1, wound healing and postoperative recovery, candidates for LVRS should be nonsmokers for at least 6 months prior to surgery.66,67 Patients dependent on high-dose corticosteroids therapy may be at risk for delayed wound healing, prolonged postoperative leaks and infectious complications. Also, these patients may have an important coexisting intrinsic inflammatory airway disease component contributing to their airflow obstruction and therefore may not benefit significantly from LVRS. A daily prednisone dose of more than 20 mg (or equivalent) was one of the exclusion criteria in the NETT.34 Nutritional status in patients with advanced emphysema is of great concern. Approximately 50% of patients undergoing LVRS for emphysema have a deficient nutritional status identifiable by a low BMI, which is associated with increased postoperative morbidity.68,69 Although patients with advanced emphysema are rarely overweight, obesity also confers a higher postoperative risk of mortality and morbidity; a BMI greater than 31.1 kg/m2 in men and 32.3 kg/m2 in women was one of the exclusion criteria in the NETT.34
Physiologic Variables
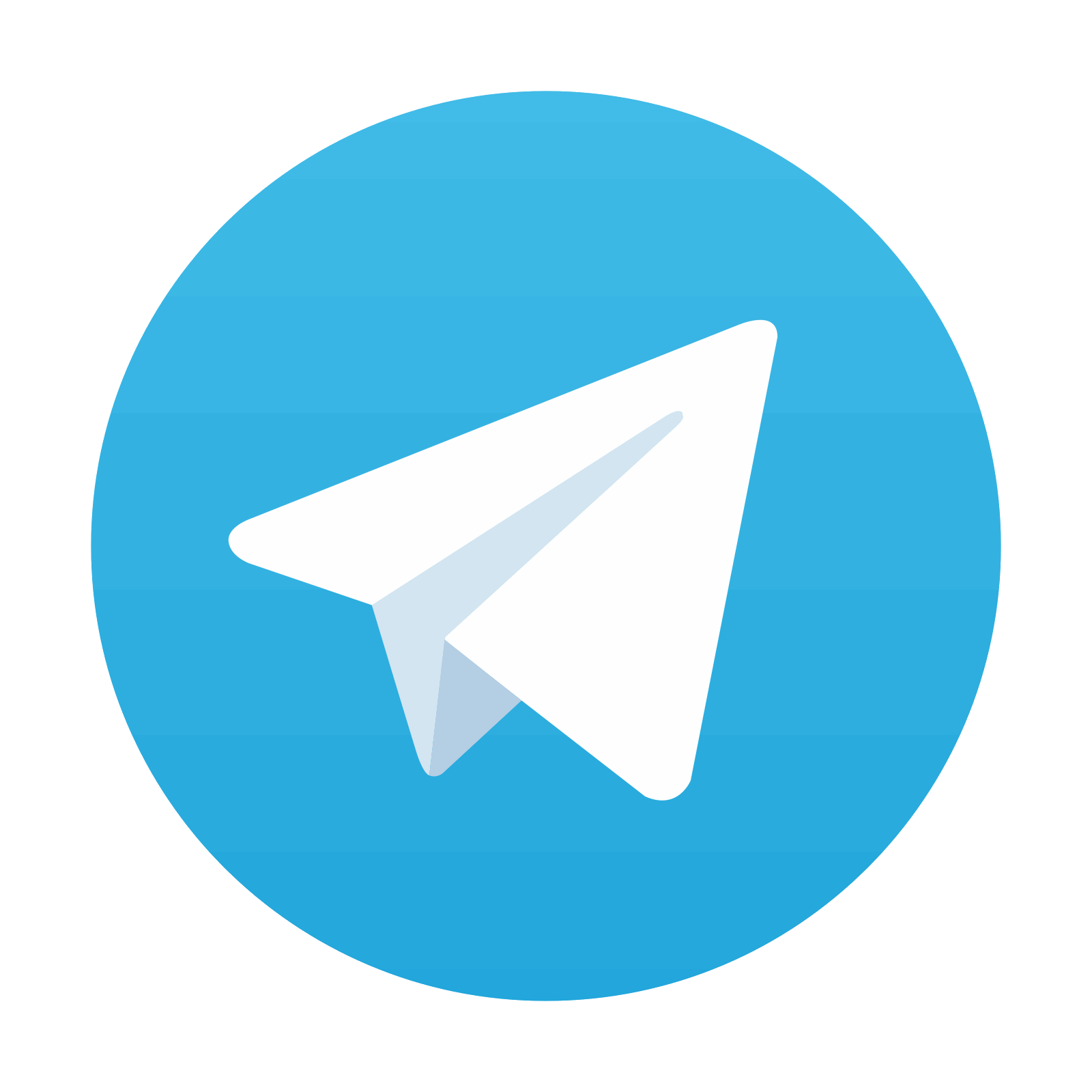
Stay updated, free articles. Join our Telegram channel

Full access? Get Clinical Tree
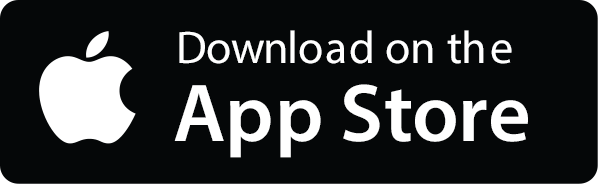
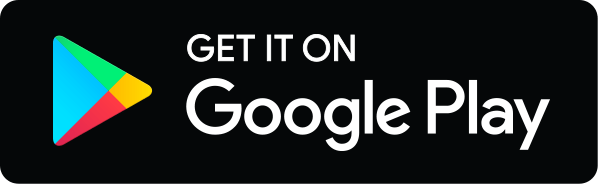