Radiographs of the pelvis are sufficient to demonstrate a hip dislocation. Hips can dislocate posteriorly, anteriorly, or inferiorly. With posterior dislocation, the femoral head migrates posteriorly and superiorly and partially projects over the acetabular roof on an AP view of the pelvis. Anterior dislocations, conversely, result in anteroinferior migration of the femoral head. Radiographs can also show associated injuries of the acetabulum and femoral head. CT scan (preferably after reduction) can better define the injury, evaluate for associated fractures, ensure the congruity of the joint, and assess for retained intra-articular fragments.
Hip dislocations require a significant amount of force to occur. These patients should be treated as trauma patients and quickly evaluated for other injuries. The most common direction of dislocation is posterior (90%), followed by anterior (approximately 10%) and inferior (much less than 1%). Visual inspection of the patient will reveal a leg that is internally rotated, shortened, and adducted with a posterior dislocation. Patients with an anterior dislocation will have more subtle changes of slight external rotation and abduction. Physical exam should also include a thorough neurovascular exam to ensure distal blood flow and sciatic nerve function. Treatment is reduction (preferably within 6 hours) to reduce the likelihood of avascular necrosis of the femoral head.
Hip dislocations are caused by a significant amount of force; evaluate the patient for life-threatening concomitant injuries.
The most common hip dislocation is posterior.
Hips should be reduced within 6 hours to reduce the likelihood of avascular necrosis of the femoral head.
Plain radiographs can usually identify a femoral neck fracture, which is almost always associated with a hip dislocation. CT scan can be used to better define the fracture and evaluate for associated fractures. Subtle fractures may only be visible on CT.
Figure 10.3 ▪ Femoral Head Fracture—Pipkin Type I.


A, B: AP view of the pelvis and a coronally-reformatted CT image of the pelvis of the same patient show a Pipkin type I fracture of the left femoral head (arrowhead). The fracture plane is distal to the fovea. On the radiograph (image A), the left femoral head is posteriorly dislocated while the Pipkin fracture fragment is rotated and resides within the empty acetabular fossa.
Femoral head fractures have an incidence of approximately 10% with hip dislocations. These fractures are classified based on the fracture location in relation to the fovea of the femoral head and associated injuries. The classification system is called the Pipkin system. For the emergency physician, the presence of a femoral head fracture is not a contraindication to hip reduction; however, it may make the reduction more difficult. The treatment of these fractures is predominately operative unless the fragment is nondisplaced and stable.
Femoral head fractures occur with hip dislocations.
The presence of a Pipkin fracture is not a contraindication to hip reduction; however, it may make the reduction more difficult.
Figure 10.4 ▪ Femoral Head Fracture—Pipkin Type II.

An AP view of the pelvis shows a large Pipkin fracture on the left extending proximal to the fovea: this is a Pipkin type II fracture. The left femoral head is posteriorly dislocated while the large Pipkin fracture fragment resides within the empty acetabulum.
A subchondral fracture of the femoral head occurs as an insufficiency or fatigue fracture. Initial radiographs are often normal, but as the disease progresses, flattening of the femoral head or a sclerotic subchondral line can be seen on radiographs. An MRI is the imaging modality of choice for initial evaluation and can demonstrate the fracture, any associated marrow edema, and the degree of subchondral collapse, if any.
A subchondral fracture of the femoral head is an infrequent cause of atraumatic hip pain. These are typically insufficiency fractures (stress fractures that occur in abnormal, demineralized bones due to physiologic, everyday stresses). These patients are generally older and do not have the common risk factors for osteonecrosis, such as smoking, alcohol abuse, trauma, or steroid use. Nondisplaced fractures heal spontaneously. Fractures that result in subchondral collapse may eventually require a total hip arthroplasty.
Include in the differential diagnosis for older patients that present with sudden-onset, progressively worsening atraumatic hip pain.
These patients do not have the common risk factors for osteonecrosis.
Figure 10.5 ▪ Subchondral Fracture.




A-D: Coronal T1 and FS T2-weighted images of the pelvis and sagital T1 and FS T2-weighted images of the right hip show marrow edema in the superior aspect of the right femoral head. A faint T1 and T2 hypointense line parallels the superoposterior articular surface. The findings are consistent with a subchondral fracture. There is no subchondral collapse.
Radiographs are sufficient to demonstrate even minimally displaced fractures through the femoral neck. Radiographs obtained with gentle axial traction may be required to differentiate between a femoral neck fracture and a basicervical fracture.
These patients present with groin pain and an inability to ambulate. The leg may be foreshortened depending on the amount of displacement. Treatment involves pain control and subsequent surgical treatment with screws, hemiarthroplasty, or total hip arthroplasty. Traction has been shown to be ineffective with these fractures. Several classification schemes are used to describe these fractures, but no single one has been shown to be clinically relevant.
If the patient is unable to perform a straight leg raise, defer range of motion testing until the radiographs are obtained (the goal is to avoid displacing a nondisplaced fracture).
Traction radiographs can help differentiate between a femoral neck fracture and a basicervical femoral neck fracture (the two require different treatments).
Initial radiographs may be normal. Disease progression creates subchondral sclerosis and cysts. With further progression, radiographs may show the crescent sign, which is due to compaction of the trabecular bone deep to the subchondral plate with rebound of the subchondral plate itself; this is a brief and transient phase. Eventually, the subchondral plate loses its ability to rebound into its normal spherical shape and permanent subchondral collapse occurs. Radiographs of chronic osteonecrosis also reveal serpiginous sclerotic lines forming geographic outlines within the superior aspect of the femoral head: these are the classic radiographic features of osteonecrosis. Radiographs during the end-stage of this disease reveal joint space narrowing and a deformed femoral head. An MRI is the most accurate imaging modality for femoral head osteonecrosis, especially in the acute phase of the disease when radiographs are still normal.
Femoral head osteonecrosis presents with insidious pain, predominately in the groin, in a younger subset of patients. This process occurs most commonly in patients aged 20 to 50 years. Patients may have an antalgic gait and will have pain with hip range of motion, especially internal rotation. Risk factors for osteonecrosis include trauma, corticosteroid use, alcohol abuse, smoking, HIV, and sickle cell disease among others. Treatment is based on the stage of disease, and ranges from activity/risk factor modification to total hip arthroplasty.
Consider this diagnosis in a younger patient with hip pain and normal radiographs.
Risk factors include trauma, HIV, sickle cell disease, smoking, alcohol abuse, and chronic steroid use.
Figure 10.7 ▪ Femoral Head Osteonecrosis.


A, B: AP and frog leg lateral view of the left hip demonstrate a geographic area of sclerosis in the superior aspect of the left femoral head consistent with osteonecrosis. Both the AP and frog leg lateral view demonstrate some subchondral collapse of the superior aspect of the left femoral head.
Figure 10.8 ▪ Femoral Head Osteonecrosis.


A, B: Coronal T1 and STIR images of the pelvis (same patient as in figure 10.7) show a serpigenous hypointense line forming a geographic area within the superior aspect of the left femoral head consistent with osteonecrosis. Minor subchondral collapse is evident on this study as well.
Acute stress fractures are invisible on radiographs and CT; they can be detected with an MRI. In the days before MRI, a bone scan was used to detect stress fractures. Stress fractures can be detected on radiographs and CT 7-10 days after the injury as jagged, fuzzy sclerotic lines within the trabecular bone; in long tubular bones, solid periosteal new bone formation will be seen over the healing stress fracture at this later stage. The sclerosis corresponds to the fracture line demonstrable on MRI and represents healing. MRI is the study of choice when a femoral neck stress fracture is suspected.
Patients with femoral neck stress fractures present with groin pain, which can be either acute or insidious. On physical exam, they will have difficulty performing a straight leg raise. If they are able to perform a straight leg raise, testing hip range of motion will cause pain. Risk factors include osteoporosis, older/postmenopausal age, female, and corticosteroid use. Younger athletes can present with these stress fractures, especially when associated with the female athlete triad. Treatment is dependent on patient factors and includes fixation with femoral neck screws, hemiarthroplasty, or total hip arthroplasty.
Making the diagnosis of a femoral neck stress fracture requires a high index of suspicion.
Acute stress fractures are invisible on radiographs and CT. For a fracture to be visible on these modalities, there must be cortical disruption. Therefore, CT does not add anything in the evaluation for an acute stress fracture and should not be obtained.
An MRI is the only imaging modality that can demonstrate an acute stress fracture and should be obtained if the index of suspicion remains high after normal radiographs.
An MRI can demonstrate the whole spectrum of injuries from normal to displaced fracture (normal-stress reaction-stress fracture-nondisplaced fracture, displaced fracture).
Figure 10.10 ▪ Femoral Neck Stress Fracture.


A, B: Coronal T1 and STIR images of the right hip (same patient as in figure 10.9) show an area of marrow edema within the medial aspect of the right femoral neck. In the middle of this area of edema and adjacent to the medial cortex, there is a thin T2 hypointense line, oriented perpendicular to the cortex of the femoral neck (arrowhead). The findings are consistent with an acute stress fracture.
Acute stress fractures of the pubic rami are radiographically occult. After 7-10 days, they become visible as sclerotic lines indicating healing. If these fractures become displaced, they become visible radiographically. Radiographs miss approximately 20% of subtle pubic rami fractures. An MRI is the only modality that can demonstrate acute stress fractures. Subtle, minimally displaced/nondisplaced fractures can be seen on CT.
Pubic rami stress fractures generally occur in elderly, osteoporotic patients. These patients present with pain localized to the rami. Treatment involves pain control and immobilization, but ramoplasty has been described.
Though not nearly as common as femoral neck stress fractures, pubic rami stress fractures do occur.
An MRI should be obtained if the index of suspicion remains high after normal radiographs.
Figure 10.11 ▪ Pubic Ramus Stress Fracture.



A: AP view of the pelvis shows an old healed left intertrochanteric femoral fracture repaired with a dynamic hip screw. Mild osteoarthritic changes are present in the hip joints bilaterally and degenerative disk disease is evident in the lower lumbar spine. The bones are diffusely demineralized. There is no evidence of an acute fracture. There is no dislocation. B, C: Coronal T1 and STIR images of the pelvis through the superior pubic rami show a jagged T1 and T2 hypointense line through the left superior pubic ramus (arrowhead) surrounded by a large amount of marrow edema that fills most of the left superior pubic ramus. The findings represent a nondisplaced fracture of the left superior pubic ramus. Regional periosteal edema is present as well. Notice that while the metal of the left dynamic hip screw produces some regional susceptibility artifact, it does not preclude evaluation of the pubic rami.
The anteroposterior pelvis radiograph is usually sufficient to demonstrate the injury. In elderly patients and those with osteoporosis, an MRI can be helpful to determine the presence of any intertrochanteric fracture extension.
These fractures predominately result from the forceful contraction of the hip abductors or a direct fall. Pain is generally localized to the greater trochanter area. Treatment of isolated greater trochanter fractures is conservative with operative intervention reserved for those fractures that are greatly displaced or nonhealing. In elderly osteoporotic patients, the possibility of intertrochanteric extension should be strongly considered because this changes the fracture category and markedly alters patient management. In these patients, the intertrochanteric extension is sometimes occult on radiographs but can be demonstrated on MRI or, sometimes, CT. In such cases, the visible, greater trochanter portion of the fracture is called “the tip of the iceberg” sign.
With elderly or osteoporotic patients, consider an MRI to evaluate for intertrochanteric extension, as the treatments are vastly different.
It is important to differentiate this fracture from calcific tendinopathy of the gluteal muscles.
The lesser trochanter can be evaluated with an anterior–posterior pelvis radiograph. Externally rotating the leg helps to evaluate the lesser trochanter. This is one of the classic pathologic avulsion fractures in adults (typically due to a metastasis at the base of the lesser trochanter). An MRI can be obtained to evaluate the extent of the fracture and to assess for an underlying lesion.
Lesser trochanter fractures are uncommon in adults and should be considered a pathological fracture until proven otherwise. These fractures cause pain either in the groin or in the posterior buttock worsened by resisted hip flexion. Treatment is usually conservative.
In adults, these fractures are pathological until proven otherwise.
Lesser trochanter avulsion fracture is the least common avulsion fracture involving the pelvis (anterior superior iliac spine, anterior inferior iliac spine, and ischial tuberosity are all more common).
Figure 10.13 ▪ Lesser Trochanter Avulsion Fracture.




A-D: AP and frog leg lateral views of the right hip show an avulsion fracture of the lesser femoral trochanter. This is a classic pathologic avulsion fracture. Indeed, this patient had metastatic lung cancer. Selected axial and coronally-reformatted CT images of the chest show a very large mass in the superior segment of the right lower lobe.
Intertrochanteric fractures by definition involve both trochanters. If there is a cortical break or any degree of displacement, the diagnosis can be established on conventional radiographs. In subtle cases, however, an MRI may be required to differentiate between an isolated trochanteric fracture and an intertrochanteric femoral fracture. Treatment options vary markedly between the two.
In younger patients, these fractures are the result of high energy impact and the patient should be evaluated for concomitant injuries. Conversely, the elderly can sustain this fracture from minor trauma. Nonetheless, elderly patients require careful evaluation for associated injuries as well. Intertrochanteric fractures require operative intervention, which should be performed within 24-48 hours of the injury.
Figure 10.14 ▪ Intertrochanteric Fracture.



A: AP view of the left hip shows a nondisplaced fracture of the greater femoral trochanter (arrowhead). The fracture is seen extending through the lateral cortex of the femur. It is not clear, based on this conventional radiograph, how far medially the fracture extends. This could be an isolated fracture of the greater trochanter or a nondisplaced intertrochanteric fracture. B: A CT scan of the pelvis was obtained to evaluate the fracture further. This coronally reformatted image shows a fracture of the greater trochanter. The fracture does not appear to extend into the medial aspect of the intertrochanteric region. C: Because the orthopoedic surgeon was concerned that this may be an occult intertrochanteric fracture, an MRI was subsequently obtained for further evaluation. This coronal FS T2-weighted image demonstrates a jagged T2 hyperintense line extending all the way through the intertrochanteric portion of the left femur (arrowheads). The findings are consistent with a nondisplaced intertrochanteric fracture. An MRI is the best modality to demonstrate such a fracture. Conventional radiographs and CT can underestimate the extent of the fracture.
Look for additional injuries when you see this fracture.
An MRI can be used to determine intertrochanteric extension if the radiographs are equivocal.
Radiographs are usually sufficient to demonstrate this injury. This fracture is defined by being within 5 cm of the lesser trochanter, but not involving the lesser trochanter.
Similar to the intertrochanteric fracture, a subtrochanteric fracture can be the result of high-energy or, in the elderly, seemingly low-energy mechanisms. Concomitant injuries are common and should be sought. Treatment is surgical fixation.
Always look for additional injuries when you see this fracture.
Radiographs are usually sufficient to demonstrate this injury.
Conventional radiographs are sufficient to demonstrate the fracture and can usually demonstrate the periprosthetic osteolysis or loosening. CT or MRI can demonstrate periprosthetic osteolysis better than conventional radiographs in complex, overlapping anatomical sites (e.g., in the pelvis or foot). At the hip, the most common site of loosening and fractures is on the femoral side.
Fortunately, periprosthetic fractures are a rare complication of total hip arthroplasty. The fractures range from nondisplaced greater trochanter fractures, which are generally stable to the fracture illustrated that will require operative fixation. Treatment is based on the stability of the implants and the type of the fracture.
Femoral-sided fractures are more common. The entire implant should be included within the field of view of the radiographs and carefully evaluated for periprosthetic osteolysis, loosening, or fracture.
Full-length femur radiographs should be obtained to assist operative planning.
Radiographs reveal a zone of lucency along the implants. Perfectly straight zones of lucency (“windshield wiper effect”) are seen with implant motion and loosening. Lumpy and undulating zones of lucency are caused by particle disease and infection. Any type of orthopedic implant can be affected.
Figure 10.19 ▪ Periprosthetic Loosening.

An AP view of the left hip shows prior open reduction and internal fixation of the proximal femoral shaft fracture with a retrograde intramedullary nail and interlocking screws. The fracture is ununited: 10 months after the injury the fracture plane is clearly visible and the fracture edges are sclerotic and flared. There is a linear zone of lucency medial and lateral to the IM rod consistent with loosening due to motion. One could easily envision how a “windshield wiper” motion of the IM rod would create this zone of lucency.
The three most common causes of periprosthetic osteolysis or loosening are particle disease, implant motion, and infection. Patients present with pain and limited function. The degree of limited function is dependent on the implant location and the degree of loosening. The presence of infection should always be considered and must be differentiated from particle disease. Orthopedic referral is required.
Figure 10.20 ▪ Particle Disease versus Infection.

An AP view of the left femur shows a located total left-hip prosthesis. There is extensive “lumpy” and irregular osteolysis along the femoral endostem, in the greater trochanter, and medial to the acetabular cup. This pattern of osteolysis is very different from the linear pattern seen in loosening due to motion. There is no way in which motion could result in this pattern of osteolysis. The differential diagnosis in this case includes particle disease and infection.
The three most common causes of periprosthetic osteolysis or loosening are particle disease, implant motion, and infection.
If left unattended, loose prostheses either dislodge or cause periprosthetic fractures.
Loosening is a common cause of pain.
Figure 10.21 ▪ Particle Disease versus Infection (Companion Case).

AP view of the right hip shows osteolysis within the greater trochanter and right acetabulum. The differential diagnosis again includes particle disease and infection. The acetabular cup has migrated superolaterally. There is a pathologic fracture through the significantly lysed and weakened medial right acetabular wall.
Figure 10.22 ▪ Infection.

An AP view of the right hip shows prior repair of an intertrochanteric fracture with a gamma nail device. There is extensive osteolysis within the greater femoral trochanter and superior aspect of the femoral neck. The differential diagnosis includes infection and neoplastic disease. A biopsy demonstrated osteomyelitis.
Radiographs may reveal evidence of a healing stress fracture (periosteal new bone formation), typically in the lateral cortex of the proximal to mid femoral diaphysis; however, an MRI may be warranted if the radiographs are negative or equivocal.
Bisphosphonate fractures result from a decreased bone turnover, leading to bone fragility. Multiple case reports of patients treated with bisphosphonates have described stress fractures in the subtrochanteric region (typically laterally)—an “atypical” stress fracture. Patients will present with mid-thigh pain. Treatment includes activity modification and bisphosphonate discontinuation.
Figure 10.23 ▪ Bisphosphonate Fracture.

An AP view of the proximal right femur shows a healing stress fracture in the lateral cortex of the proximal right femoral diaphysis (arrowhead). This location is typical for a stress fracture occurring in a patient who has been or is currently on bisphosphonate therapy for osteopenia/osteoporosis.
Consider these atypical stress fractures in patients on bisphosphonate therapy.
An MRI should be considered if the clinical suspicion is high and the initial radiographs are normal or equivocal.
Radiographs are sufficient to demonstrate the injury.
Femoral fractures in young patients are the result of high-energy impact. Elderly patients may suffer this injury with lower-energy mechanisms, such as a same-level fall. Femoral fractures can be associated with significant blood loss, and therefore patients should be evaluated for concomitant injuries. Profuse bleeding caused by these fractures can lead to hypotension. Treatment is surgical.
Most femoral fractures are due to high-energy impact.
Assess all patients with femoral fractures for associated injuries.
Radiographs are the imaging study of choice and are sufficient to demonstrate the injury.
These injuries are usually obvious with a ballistic wound and deformity. Focus should be on resuscitation and ensuring adequate distal blood flow. Treatment is surgical.
Resuscitate the patient and check for distal pulses.
Vascular imaging may be required based on the patient’s exam. CT arteriogram and venogram are the studies of choice.
Figure 10.27 ▪ Ballistic Femur Fracture.

An AP view of the proximal left femur shows a comminuted ballistic fracture of the low intertrochanteric and subtrochanteric left femur. Bullet fragments are present along the path of the bullet. A paper clip paced on the skin laterally denotes an entry or exit wound.
Radiographs are sufficient to demonstrate the injury.
Supracondylar fractures can result from high-energy mechanisms in younger patients, but may occur with low-energy mechanisms in the elderly. Patients present with an obvious deformity and pain proximal to the knee. Exam reveals tenderness in this area. The clinician should differentiate this injury from a knee dislocation by palpating the joint line. A thorough neurovascular exam should be performed as well. Treatment for these fractures is operative fixation.
Differentiate this fracture from a knee dislocation by palpating the joint line.
Vascular injuries do occur due to the proximity of the femoral artery to the fracture site.
Radiographs can demonstrate the fracture and are very helpful in characterizing the underlying osseous lesion. It is the radiographs that reveal the matrix calcification pattern, if present, as well as define the type of underlying lesion (type of osteolysis, pattern of sclerosis, type of periosteal new bone formation). Most tumors are quite nonspecific on MRI (isointense to muscle on T1, hyperintense on T2, enhance after IV Gadolinium injection). The role of an MRI is to define the precise extent of the underlying neoplasm, the degree of osseous involvement, the soft tissue extension of the mass, and the relationship of the mass with other soft tissue structures (particularly the regional neurovascular structures).
Pathological femoral fractures are uncommon and generally result from low-energy mechanisms. The pathology can be either from metastasis (most common), primary bone tumors, or abnormal bone, such as Paget’s disease. Treatment is surgical.
Breast, lung, prostate, kidney, and thyroid are the common cancers that metastasize to bone.
Consider pathological fractures with low-energy mechanisms.
Figure 10.29 ▪ Pathologic Femur Fracture.


A, B: An AP view of the left hip shows a varus-angulated and mildly displaced pathologic fracture through an aggressive, moth-eaten, lytic lesion in the proximal left femoral diaphysis. This unfortunate patient had just had a PET CT examination 7 days earlier showing a large FDG-avid lytic lesion in the proximal left femur (arrow).
Figure 10.30 ▪ Pathologic Intertrochanteric Fracture.

An AP view of the left hip shows a pathologic low intertrochanteric fracture in a patient with Paget’s disease. Notice the classic features of Paget’s affecting the proximal left femur: the bone is mildly expanded and diffusely sclerotic, the cortex is thickened, and the trabeculae are coarse and disorganized.
Radiographs are the imaging study of choice and usually sufficient in demonstrating the injury. A CT may aid in the diagnosis if the fracture is markedly comminuted.
Periprosthetic fractures associated with total knee arthroplasty are more common in patients with osteoporosis and after revision surgery. The supracondylar femoral fractures are related to notching the femur during surgery. Treatment can be nonoperative as long as the fracture is not significantly displaced and the components are stable.
Periprosthetic fractures after total knee arthroplasty can occur with any of the components: femoral, tibial, or patellar.
Full-length tibia or femur radiographs will be required to assist in operative planning.
Radiographs are sufficient to demonstrate the injury, if the joint is still dislocated. Reduced and splinted joints may appear radiographically normal, as the alignment would be restored. Look carefully for abnormal widening of joint spaces or subtle malalignment. The ligamentous injuries associated with a knee dislocation can be evaluated with an MRI.
These patients often present dramatically. The most common dislocation is anterior, but the dislocation can also be posterior, medial, lateral, or rotatory. Approximately 50% of knee dislocations spontaneously reduce in the field. These patients will not have the same dramatic physical deformity but will complain of severe pain. Spontaneously reduced dislocations still carry the same likelihood of neurovascular injury. A careful neurovascular exam should be performed to assure some degree of distal blood flow; if absent, emergent reduction should be performed. After reduction, further testing, including the ankle-brachial index (ABI), should be performed. If the ABI is less than 0.9, vascular imaging should be performed. Definitive treatment is dependent on the ligaments that are injured.
Approximately 50% of knee dislocations spontaneously reduce in the field; however, they carry the same likelihood of neurovascular injuries.
Look carefully for abnormal widening of joint spaces or subtle malalignment.
The ligamentous injuries associated with a knee dislocation can be evaluated with an MRI.
Anterior Cruciate Ligament Tear Indicators—Segond Fracture, Deep Sulcus, and Anterior Tibial Spine Avulsion
Anterior cruciate ligament (ACL) injuries are best imaged with an MRI; however, there are certain radiographic abnormalities (the Segond fracture, deep sulcus sign, and anterior tibial spine avulsion fracture) that have an extremely high association with ACL tears. These findings are not sensitive but they are very specific for concomitant ACL tears.
Figure 10.33 ▪ Segond Fracture.

An AP view of the knee shows a tiny avulsion fracture from the lateral aspect of the lateral tibial plateau (arrow). This is an avulsion of the lateral capsule and is called a Segond fracture. It has a very high (>90%) association with a tear of the anterior cruciate ligament.
Patients will prevent after an instability event with knee pain. Initial physical exam may be limited due to pain. The vast majority of patients will have a joint effusion. Specific knee-ligament injuries are difficult to discern based on a clinical exam. Initial treatment in the ER is conservative with compression (not a knee immobilizer), ice, and pain control.
ACL tears are diagnosed with an MRI, but the presence of a Segond fracture, deep sulcus, or anterior tibial spine avulsion on the initial radiographs can point to the diagnosis.
These indicators are specific but not sensitive for ACL tear.
Figure 10.37 ▪ Subchondral Fracture.

A lateral view of the left knee shows a subtle subchondral fracture in the region of the sulcus terminalis of the lateral femoral condyle (arrowhead). In the setting of ACL injury, the femur rotates in such a fashion that the lateral femoral condyle often impacts against the posterior aspect of the lateral tibial plateau. This frequently results in “kissing” contusions or areas of marrow edema in the lateral femoral condyle and posterior lateral tibial plateau. If the impaction forces are greater still, then a subchondral fracture of the lateral femoral condyle can occur, as seen in this patient.
Figure 10.38 ▪ Subchondral Fracture.



A-C: Sagital PD and FS T2-weighted images through the lateral knee compartment of the same patient as in figure 10.37 show a subtle subchondral fracture in the region of the sulcus terminalis of the lateral femoral condyle (arrowhead). There is some associated marrow edema. A sagital PD-weighted image through the intercondylar notch shows a proximal ACL tear (arrow).
Figure 10.40 ▪ Tibial Spine Avulsion Fracture.



A-C: Sagital PD and FS T2-weighted and coronal FS PD-weighted images of the knee of the same patient as in figure 10.39 show an intact ACL attaching into an avulsed anterior tibial spine. Joint effusion, tibial marrow edema, and periarticular soft tissue edema and fluid are also present.
Figure 10.41 ▪ Multiple Knee Fractures (Companion Case).

Segond fracture, anterior tibial spine avulsion, and arcuate fracture. These AP and lateral views of the right knee show evidence of extensive ligamentous injury, including injury to the ACL and postero-lateral corner. The avulsed fragment of the fibular head is the site of attachment of the fibular collateral ligament and biceps femoris tendon: two of the major stabilizers of the postero-lateral corner of the knee.
Radiographs typically reveal a joint effusion. The specific ligamentous injuries are demonstrable on MRI.
Posterolateral corner injuries may involve the biceps femoris tendon, fibular collateral ligament, popliteus tendon or muscle, lateral capsule, and a number of other less important ligaments that are not usually visible on conventional MRI sequences (arcuate ligament, popliteofibular ligament, etc). These injuries are rarely seen in isolation; ACL and/or PCL tears are usually present. Patients will present with pain, instability, and a knee effusion. Treatment depends on co-existing ligamentous injuries. It is critical that posterolateral corner injuries are identified and repaired with the cruciate ligament (usually ACL) repair; otherwise, the ACL repair will fail.
Posterolateral corner injuries require a great deal of force; always keep the possibility of a knee dislocation on your mind when evaluating these injuries.
An MRI is the study of choice to evaluate for ligamentous injuries.
Figure 10.42 ▪ Posteriolateral Corner Injury.



A-C: Selected coronal FS PD and axial FS PD-weighted images of a knee show tears of the distal biceps femoris tendon (arrow), proximal lateral collateral ligament (arrowhead), and hemorrhage within the popliteus muscle (open arrow). Extensive periarticular soft tissue edema and hemorrhage is present as well. The patient had an ACL tear as well (not shown).
Radiographs are normal, except for a possible effusion. Occasionally, a radiograph may demonstrate an extruded meniscus containing chonrocalcinosis (tiny deposits of calcium pyrophosphate dihydrate crystals). An MRI is the study of choice. Meniscal tears are hyperintense signal abnormalities within a meniscus that reach a meniscal surface (the superior surface, inferior surface, or free edge of the miniscus). Globular signal abnormalities within the substance of a meniscus that do not reach a meniscal surface are either areas of myxoid degeneration (usually in older patients) or meniscal contusion (in acute injuries, younger patients). An MRI can demonstrate the exact type and extent of the meniscal tear, as well as associated abnormalities (flipped or displaced meniscal fragments, perimeniscal cyst, intrameniscal cyst, bone contusion patterns, cartilage loss).
Meniscal tears can be either acute or chronic. Patients will present with knee pain and mechanical symptoms such as catching or locking. Physical exam can reveal joint line tenderness and possibly an effusion. It is extremely important to assess the injured joint’s range of motion as a bucket-handle meniscal tear can limit extension and necessitate expedited surgical intervention. Treatment for acute meniscal tears is surgical; treatment for chronic tears varies.
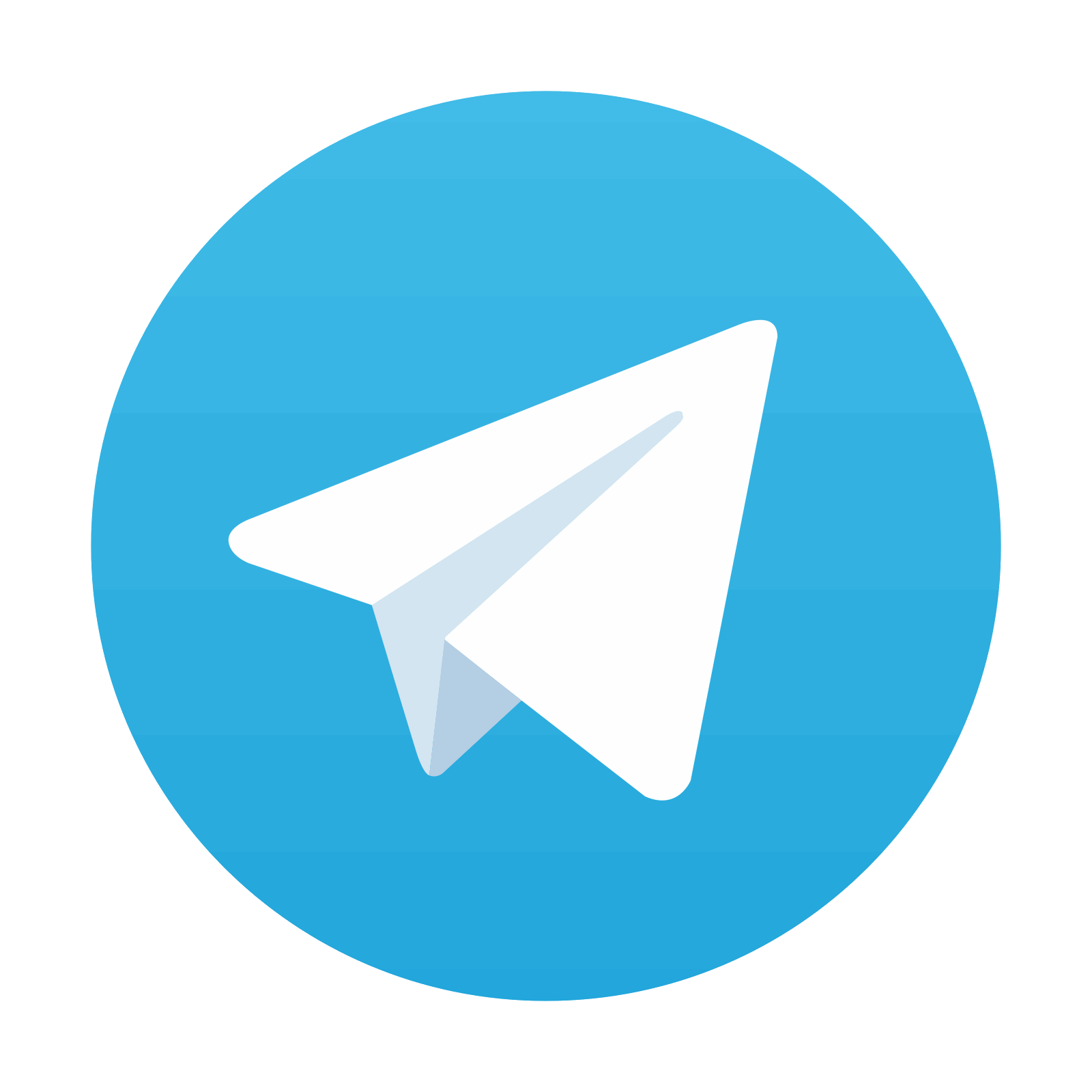
Stay updated, free articles. Join our Telegram channel

Full access? Get Clinical Tree
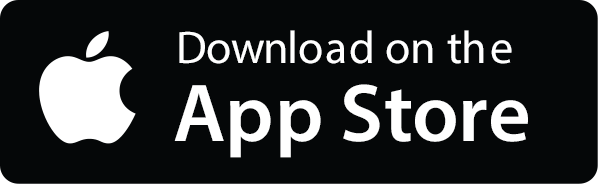
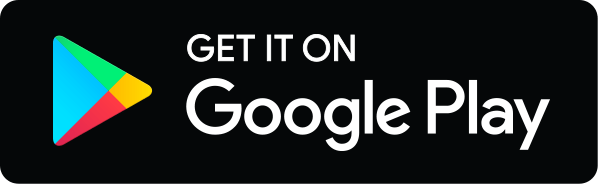