© Springer International Publishing Switzerland 2016
Marinella Astuto and Pablo M Ingelmo (eds.)Perioperative Medicine in Pediatric AnesthesiaAnesthesia, Intensive Care and Pain in Neonates and Children10.1007/978-3-319-21960-8_2424. Long-Term Consequences of Anesthesia (and Surgery) on the Infant Brain
(1)
Department of Anesthesiology and Intensive Care – Pediatric Section, Odense University Hospital, Odense, DK-5000, Denmark
(2)
Institute of Clinical Research – Anesthesiology, University of Southern Denmark, Odense, DK-5000, Denmark
24.1 Introduction
Very few issues (if any) within pediatric anesthesia have caused so much concern and emotional gravity than the fact that the past roughly 15 years, a plethora of animal studies have uniformly shown that exposure to most of the currently used anesthetics possibly during a vulnerable period of brain development (i.e., brain growth spurt or peak of synaptogenesis) may lead to neurodegeneration (particularly apoptosis) and abnormal synaptic development [1–4]. Importantly, the observed morphological abnormalities are associated with functional deficits in learning and behavior later in life [5]. Initial studies were mainly performed in immature rodent pups, but more recent studies have included nonhuman primates (rhesus monkeys) [6–8]. Given the number of neonates, infants, and young children anesthetized annually worldwide, these findings could have significant public health implications. Both gamma-aminobutyric acid (GABA) agonists (e.g., inhalational anesthetics, propofol, benzodiazepines) and n-methyl-d-aspartate (NMDA) antagonists (e.g., ketamine and nitrous oxide) are implicated [3, 4]. Although results from animal studies using opioids, α-2 receptor agonists, and xenon are less overwhelming – and in some cases/circumstances have indicated that these agents may in fact possess neuroprotective properties – they are generally inconclusive.
Translating these animal studies into a human clinical context is difficult. How do various developmental stages of brain development in animal models translate into humans? The anesthetic techniques and managements used in the majority of animal (rodent) studies are extremely different to normal clinical practice, for example, the use of supra-clinical doses and long duration of exposure to anesthetic drugs sometimes resulting in excessively high mortality (20–80 %). Additionally, the use of multiparameter monitoring and control of airway and respiration are difficult (or even impossible) due to the small size of the neonatal animals, which also precludes repeated blood gas and glucose measurements due to small circulating blood volumes [3]. This may be of utmost importance. A recent animal study by Wu et al. compared the effects of mechanical ventilation and spontaneous breathing on outcome in 14-day-old rats exposed to isoflurane and sevoflurane. Compared with mechanical ventilated rats, spontaneous breathing rats had significantly higher mortality, neuroapoptosis, and impaired neurocognitive outcome [9].
24.2 Limitations of Animal or Preclinical Data
The initial animal studies on anesthesia-related neurotoxicity and the developing brain were never driven by any clear or well-defined associations between general anesthesia and subsequent specific neurocognitive deficits in humans or the applicability to clinical care of human infants. Additionally, many inherent factors limit the applicability of animal-derived data to humans. More specifically, ontogenic differences in neurodevelopment between humans and animals that may impact outcome are largely unknown. Factors regarding the administration of anesthesia and study design, for example, differences in anesthetic drug potency between human and animals, lack of surgical stress, differences in monitoring and correction of physiologic, metabolic, and biochemical aberrations induced by anesthetic drugs and surgery, use of excessively high doses of anesthetics for a long period of time, and use of agents that are rarely used in modern clinical practice (such as ketamine, isoflurane, and nitrous oxide), may complicate matters [1]. Furthermore, there is a lack of systematic evidence for the clinical benefits of animal research in general and animal neuroscience in particular [10].
24.3 Normal Development of Human Brain
Morphology and function of the brain is not a static process but rather a complex, continuous, and lifelong process. Immediately after birth, the brain is about 25 % of its adult size and unevenly matured [3, 4]. The brain stem and spinal cord are relatively well developed, whereas the limbic system and cerebral cortex are still immature. Despite being produced before birth, the cortical neurons are poorly connected, and most synapses are produced after birth during a peak synaptogenesis where the rate of cortical synapse formation is estimated to be two million new synapses per second. At the age of 2 years, the cerebral cortex comprises more than 100 trillion synapses. The newborn brain contains little myelin, and myelination appears to be virtually an “automatic or innate” process in that its sequence succession is very predictable, and it appears that severe malnutrition is the only single individual environmental factor known to impact myelination. Sensory afferent stimuli before and after birth differentiate the function of neurons and neural pathways, ensuring neuroplasticity of the CNS [3, 4]. However, this plasticity may be a two-edged sword in that it implies that on one hand young children’s brains are more open to learning and enriching influences on the other they may also be more vulnerable and susceptible to environmental changes. Selection and formation of active neural circuits (pruning) occurs throughout life but is much more common in early childhood. This initial excess of neurons and synapses is followed by significant neuronal death and decline in synapses. This normal and DNA-programmed cell death or apoptosis is necessary to ensure normal CNS morphology and function. It is an intimate and integral part of normal brain development and maintenance. The net result is that <50 % of neurons survive into adult life. Any interference with this normal apoptotic process may have detrimental effects to the central nervous system. All commonly used anesthetics have been shown in preclinical studies to markedly increase neuroapoptosis. However, several other studies have subsequently also documented acute neuronal cell loss, altered dendritic architecture, reduced synaptic density, destabilization of the cytoskeleton, and cell cycle abnormalities following exposure to all commonly used general anesthetics [3, 4]. For obvious reasons, it remains to be proven whether similar anesthesia-induced neurodegeneration occurs in human clinical settings.
24.4 Human Studies
Relative to the magnitude of animal investigations, the number of human studies is relatively limited. The majority of these are listed in the reference list and have recently been reviewed in details elsewhere [11, 12], where more detailed information about these studies can be obtained. Fortunately, all these studies have been inconclusive, that is, it has been very difficult to show whether this animal phenomenon has a human corollary [13–26]. In the following, the most important issues related to the human studies are presented.
24.5 Relevant Human Outcome Measure
It remains to be determined how anesthesia-related neurotoxicity (if it exists) will reveal itself in humans. Contrary to babies with, for example, fetal alcohol syndrome or babies born to mothers on certain antiepileptic drugs in whom distinctive mental and physical defects are well described, any detrimental effect associated with anesthetic exposure in infancy must be of a minor nature [3]. Otherwise, it would be easy to demonstrate and it would most likely have been suspected many years ago. Therefore, the following questions are of utmost importance: what is a meaningful outcome measure and when and how do we best measure it? Should we search for developmental disorders in preschool, learning disabilities (LD) in elementary school, social disturbances in adolescence, or psychiatric disorders in adulthood, and what relevance has loss of various cognitive functions or early dementia in the elderly? To what extent are sensorimotor deficits or psychiatric disorders relevant? More importantly, how well does a single short-term interim measure performed in early childhood or adolescence adequately predict outcome later in life, and what are the long-term consequences of that? Currently, we do not have the answers.
Learning disabilities is a very crude outcome influenced by many underlying factors, for example, chronic diseases and environmental factors. More importantly, LD is not a specific neuropsychological outcome measure, but rather a categorical determination arising from differences between a child’s educational outlook (e.g., IQ) and his or her actual achievement. Focusing on the cumulative incidence of LD implies that follow-up is ceased when an LD is identified, and a priori it is then thought that the LD will persist and never disappear. This impedes estimation of the true prevalence of this outcome measure. Further, a child labelled with LD may at some point in life have a change in achievement placing him or her back in the normal range. Such an incident will not be detected by the current used study designs. Assessment of academic performances has a pragmatic advantage over, for example, IQ testing or similar in that parents are likely to be more interested in how their child will do in school. A recent study employing extensive and repeated neurodevelopmental testing suggested that group testing such as academic performance may lack sufficient sensitivity to detect smaller, minor, or more subtle neurocognitive impairment between children exposed and children not exposed to anesthesia compared with individually administered cognitive tests [26]. It has been claimed that studies using individually administered tests of cognition may be more likely to be positive into any potential phenotype (e.g., abnormalities in speech and language). However, at this point, we do not know under what circumstances individual cognitive testing are actually also meaningful human outcome measures. Many of the outcomes used in these studies are interrelated. One question quickly arises: exactly how different are individually tests of speech and language and school tests? Certainly, good academic achievements require good speech and language skills [27].
Attention-deficit hyperactivity disorder (ADHD) has also been tested as a primary outcome measure to assess for anesthesia-related neurotoxicity [13]. However, the diagnosis and treatment of ADHD are highly disputed. The diagnosis of ADHD is afflicted by ascertainment bias as demonstrated by the large variation in its prevalence across countries, states, races, genders, and ethnicities. Further, in 2011, two million more children were diagnosed with ADHD in the USA compared with 2003, and overall one out of five US teenage boys was labelled with an ADHD diagnosis (more than two-third of these were taking long-term medication such as dexamphetamine). Thus and because ADHD is associated with an array of psychiatric disorders and learning disabilities, its value as an outcome measure in studies on anesthesia-related neurotoxicity is questionable [28, 29]. Recently, autism spectrum disorder has also been used as outcome measure in these types of studies [30]. Similar arguments as just mentioned in relation to ADHD can been applied regarding this outcome measure [31].
24.6 Migration
The degree of migration of study subjects is of major concern in many of the human cohort studies published thus far. As high a proportion as one-third of the original cohort has been reported in some series to move such that information from these individuals could not be retrieved [13, 14]. This is an important issue because significant differences between migrants and nonmigrants are well noted in some of these cohort studies increasing the likelihood for selection biases [32]. Families with a significantly ill child living in close proximity to a major pediatric center are less likely to migrate. Furthermore, children with significant comorbidities are more likely to suffer neurobehavioral problems and require multiple anesthetics.
24.7 Sample Size Issue
The problem surrounding the “sample size issue” was addressed in one of the first human studies on this topic [23]. Since then it has become increasingly apparent that sufficiently large sample sizes are needed to detect modest associations among the multitude of perioperative factors that impact neurocognitive outcomes (see later). In fact, very few of the current available human cohort studies have large sample sizes [21, 22]. The introduction of multiple (individual) testings has been employed to compensate for this fact [19]. However, such an approach carries a high-risk Type I statistical error, which (as mentioned earlier) may be a particular risk in the present context in that many of the tests use are interrelated [27]. Similar problems have been encountered in studies on postoperative dysfunction (POCD) in the elderly [33, 34]. The reality is that to date, there are no reliable tools available to test for the presence of POCD, and simply increasing the number of tests to assess and classify POCD also increases the rate of false-positive classifications.
24.8 Duration and Number of Exposures
Preclinical data suggests that larger anesthetic doses and longer duration may be associated with greater effects (i.e., poorer outcome) [1–9]. Similarly, human studies suggest that one single and short-term anesthetic (and surgical) exposure seems harmless, whereas multiple exposures are associated with poorer outcome (i.e., increased hazard ratios) [14–26]. However, such an association is not necessarily causal, because children who require multiple surgeries are far more likely to suffer serious underlying conditions that – as mentioned above – may independently impact neurodevelopment. Additionally, the effects from surgeries (e.g., stress responses, pain, and neuroinflammation) cannot be disentangled from that of the anesthetic itself in these studies [11, 12]. Very few studies have focused on longer exposures/procedures [35, 36]. In these studies, it appears that many other confounding factors are by far more important than merely exposure to anesthetics.
24.9 Age at Exposure
In preclinical studies, timing of exposure to anesthetic drugs seems to play a pivotal role in mediating the degree of injury as the brain may have periods of increased vulnerability during different stages of development [37]. In young rodents, the period of greatest vulnerability appears to be between postnatal 4 and 10 days (~PD7) and corresponds to the period of the peak synaptogenesis. In guinea pigs, this corresponds to mid-gestation, whereas in rhesus monkeys, it corresponds to up to 35 days of age [3, 4]. In humans, the period of greatest synaptogenesis is thought to occur during the last trimester and up to 2 to 3 to 4 years postnatally. However, there is no evidence to support this contention. Additionally, all brain regions may not develop uniformly, and different regions may be differentially susceptible during different stages of development [3, 4, 38, 39]. A free available website was launched almost 10 years ago to improve accuracy in extrapolating vulnerable periods between different animal species. This website used more modern models and the newest brain developmental data (www.translatingtime.net) [38, 39]. Unfortunately, this new bioinformatics method has not yet been implemented in animal studies on anesthesia-related neurotoxicity. By integrating data from core developmental stages and events from many mammalians into a statistical model, these animal models suggest that the developmental stage of, for example, a rodent cerebral cortex at PD-7 may actually correspond to the maturational stage of the human brain at the very beginning of third trimester [39]. This may well mean that animal experiments using 7-day-old rodents may correlate with providing anesthetics to extremely premature babies.
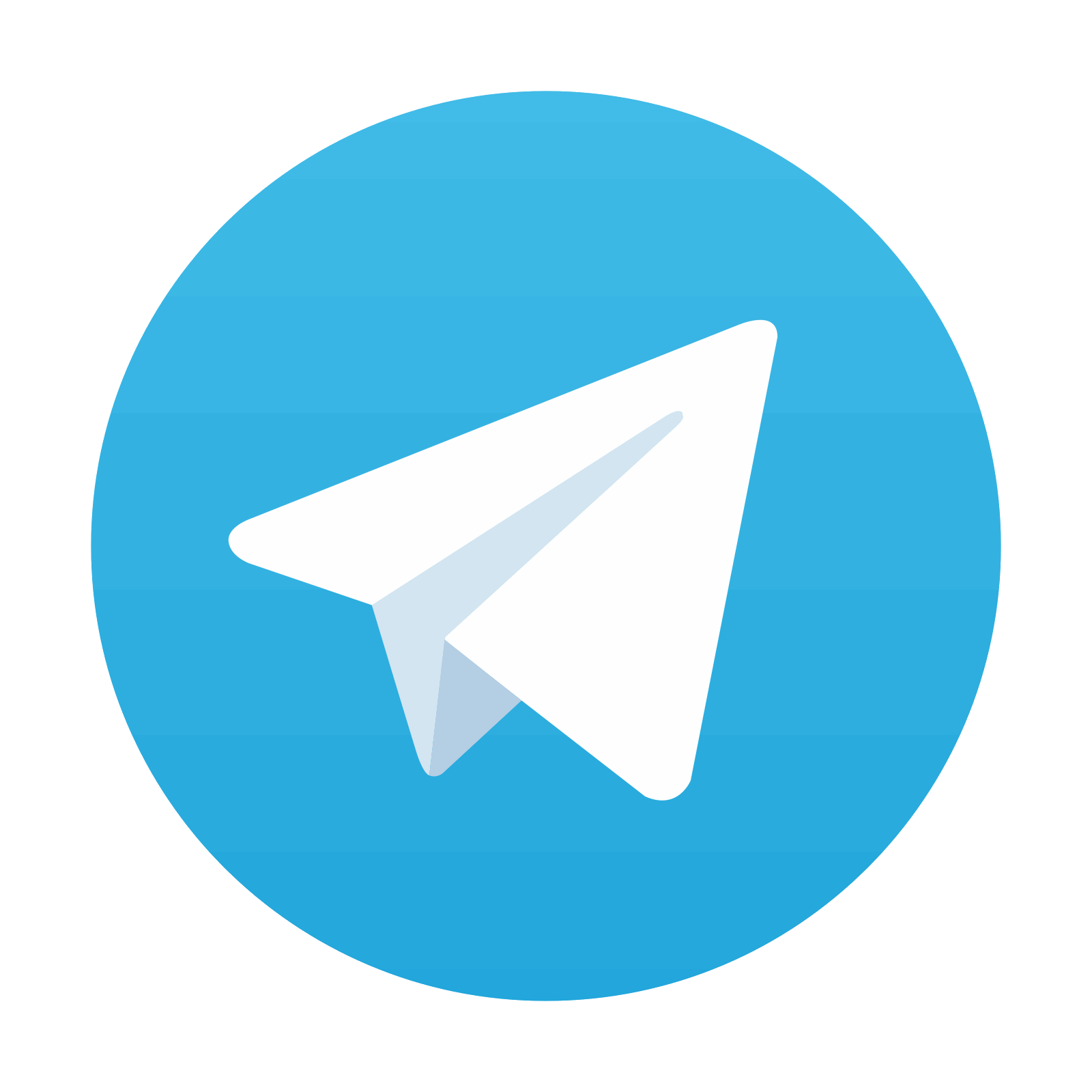
Stay updated, free articles. Join our Telegram channel

Full access? Get Clinical Tree
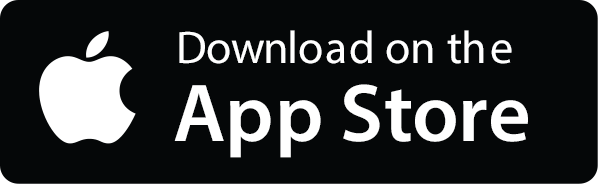
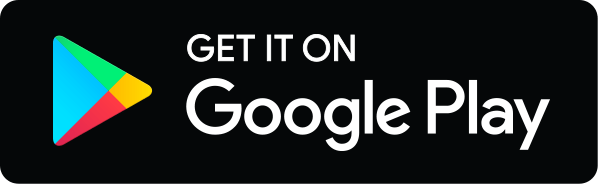