Local Anesthetics in Obstetrics: Evidence-based Applications, Controversies, Toxicity, and Current Therapies
Barbara M. Scavone
Introduction
A wide variety of local anesthetic drugs are employed in obstetric anesthesia practice. These agents are administered during intrathecal and epidural anesthesia, during peripheral nerve blockade procedures such as pudendal nerve block, and subcutaneously. Safe use of these medications necessitates knowledge of their chemical properties, pharmacodynamics, and pharmacokinetics. The clinician must also become familiar with the diagnosis and treatment of various adverse reactions, the most serious of which is local anesthetic systemic toxicity. Understanding these pharmacologic principles will allow for a rational approach to the use of these therapeutic agents.
Local Anesthetic Chemical Structure and Mechanism of Action
The commonly used local anesthetics possess a similar chemical structure consisting of a lipid soluble aromatic ring, a hydrocarbon chain, and a terminal amine group, with various R chains throughout (Fig. 8-1). Amino-ester local anesthetics contain an amide bond between the aromatic ring and the hydrocarbon chain. Commonly used amino-ester anesthetics include procaine, chloroprocaine, tetracaine, and cocaine. (Cocaine is not commonly administered to parturients due to teratogenicity.) Amino-amide local anesthetics have an amide group linking the aromatic ring and the hydrocarbon chain. Lidocaine, bupivacaine, levobupivacaine, ropivacaine, and mepivacaine are examples of amino-amides.
Local anesthetics reversibly block neural conduction when administered near central or peripheral nerves; they do so by reversibly binding a specific receptor site on voltage-gated Na+ channels, preventing influx of Na+ into the cell, and thus preventing generation of an action potential (Fig. 8-2) (1,2,3). The clinical properties of the individual local anesthetics are determined by interplay of many factors including the drug’s charge, pKa, lipid solubility, and protein binding (Table 8-1). First, the local anesthetic must gain entry into the cell; only the uncharged form of the molecule can enter the cell in significant amounts (4,5). Clinically used local anesthetics are weak bases; the molecule accepts a proton at its terminal amine group to become charged. The pKa is the pH at which a molecule exists equally in the charged and the uncharged forms. Since local anesthetics have pKa’s greater than physiologic pH, the human body is a relatively acidic environment, favoring formation of the protonated charged moiety. The further away from physiologic pH the pKa of the drug, the greater the proportion that is ionized, unable to cross the cell membrane. Therefore, the pKa of a local anesthetic partially determines its speed of action, such that those with a lower pKa (closer to physiologic pH, less charged) tend to have a faster onset. (An exception to this general rule concerns chloroprocaine, which has a short onset latency despite its high pKa.)
Lipid solubility augments the passing of the molecule into and through the cell membrane, and thus, in part determines the local anesthetic’s potency (6).
Once inside the neuron, the charged form of the local anesthetic binds the voltage-gated Na+ channel at a specific receptor site on its inner surface (4,5). Binding interferes with conformational changes in the Na+ channel necessary for opening, and thus prevents the translocation of Na+ ions into the cell; an action potential is not generated when threshold depolarization is reached. The local anesthetic demonstrates a higher likelihood of binding to the channel when it is in its open/activated or closed/inactivated state (the states associated with depolarization), rather than its resting state (1). This contributes to the phenomenon known as “phasic block” or “use-dependent” or “frequency-dependent” blockade: The tendency for nerves that fire action potentials frequently to be more sensitive to the effects of local anesthetics than those that do not fire as often.
Eventually the local anesthetic dissociates from the binding site, leaves the cell and is metabolized. Smaller molecules leave the site quickly; very large ones, slowly. Intermediate-sized local anesthetics enter the cell relatively rapidly, and leave at rates dependent on the drug’s shape, structural flexibility and lipophilicity. Moderate lipophilicity (e.g., lidocaine) augments drug departure, and extreme lipophilicity (e.g., bupivacaine) tends to favor continued binding (7). In addition, agents that are soluble into perineural lipids and/or tightly bound to perineural proteins demonstrate slower rates of absorption into the intravascular compartment and thus, both lipid solubility and protein binding influence duration (8,9).
Differential Blockade
Differential blockade refers to the tendency of nerves among different classes to exhibit varying degrees of susceptibility to local anesthetic blockade. It is a result of axon myelination, diameter, and function (Table 8-2). Myelination causes a neuron to be more sensitive to local anesthetics. In unmyelinated fibers, action potential generation causes the area adjacent to reach threshold potential, and thus, the action potential is propagated. A large length of nerve axon must be blocked in order to prevent propagation in this manner. In contrast, in myelinated nerves, one
need block only three successive nodes of Ranvier to block action potential propagation (generally a shorter length of the neuron) (10).
need block only three successive nodes of Ranvier to block action potential propagation (generally a shorter length of the neuron) (10).
Large diameter axons demonstrate resistance to blockade as compared to axons of small diameter. This may be a function of the diameter itself, but may also represent the fact that the nodes of Ranvier are placed at intervals farther apart in large diameter nerves, and therefore a greater axonal length must be exposed to anesthetic before three successive nodes are blocked (10,11,12).
It is possible that rates of differential blockade that seem to correlate with axon diameter and myelination status actually vary according to functional class of the nerve, and are due to anatomic and physiologic differences that exist among nerves of varying functions. This might include variations in the density and gating behavior of particular ion channels, the density of ion pumps, or characteristics of the myelination (13).
Anatomic features such as the location of a nerve within a trunk may determine some blockade features. Also, the principle of phasic block dictates that rapidly firing nerves are more sensitive to conduction blockade than nerves that fire less frequently. Preganglionic sympathetic nerve fibers have a high rate of tonic firing and are easily blocked; sensory neurons fire more frequently than motor neurons, and are more affected by local anesthetics.
Several important implications follow from the concept of differential blockade. During spinal and epidural anesthesia,
a site of maximal concentration of local anesthetic exists, termed the epicenter, which is dependent on injection site, patient position, drug baracity, etc. Anesthetic concentration then decreases as a function of distance from the epicenter. Zones of differential blockade develop, because the sympathetic nerves (most sensitive) may be affected along the entire concentration gradient, but the motor nerves (least sensitive), only at sites of greatest concentration density. Sympathetic blockade usually exceeds sensory blockade by several dermatome levels during central neuraxial anesthesia, even if sympathectomy is not always complete (Fig. 8-3) (14,15,16). Decreased temperature sensation occurs at a higher dermatome level than decreased sensation of sharp pain, which exceeds light touch (Fig. 8-4) (17,18). Therefore it is advisable to assess sensitivity to some painful stimulation such as pinprick rather than simply to cold sensation prior to skin incision. The Aδ fibers, which mediate sharp pain, are blocked at lower concentrations of local anesthetic than are the C fibers, associated with burning pain (13). Lastly, since motor neurons are relatively resistant to blockade, dilute solutions of local anesthetic can be administered to provide labor pain relief without affecting maternal expulsive efforts (19).
a site of maximal concentration of local anesthetic exists, termed the epicenter, which is dependent on injection site, patient position, drug baracity, etc. Anesthetic concentration then decreases as a function of distance from the epicenter. Zones of differential blockade develop, because the sympathetic nerves (most sensitive) may be affected along the entire concentration gradient, but the motor nerves (least sensitive), only at sites of greatest concentration density. Sympathetic blockade usually exceeds sensory blockade by several dermatome levels during central neuraxial anesthesia, even if sympathectomy is not always complete (Fig. 8-3) (14,15,16). Decreased temperature sensation occurs at a higher dermatome level than decreased sensation of sharp pain, which exceeds light touch (Fig. 8-4) (17,18). Therefore it is advisable to assess sensitivity to some painful stimulation such as pinprick rather than simply to cold sensation prior to skin incision. The Aδ fibers, which mediate sharp pain, are blocked at lower concentrations of local anesthetic than are the C fibers, associated with burning pain (13). Lastly, since motor neurons are relatively resistant to blockade, dilute solutions of local anesthetic can be administered to provide labor pain relief without affecting maternal expulsive efforts (19).
Table 8-1 Chemical Properties of Local Anesthetics | |||||||||||||||||||||||||||||||||||
---|---|---|---|---|---|---|---|---|---|---|---|---|---|---|---|---|---|---|---|---|---|---|---|---|---|---|---|---|---|---|---|---|---|---|---|
|
Table 8-2 Neuronal Properties that Determine Local Anesthetic Sensitivity | ||||||||||||||||||||||||||||||
---|---|---|---|---|---|---|---|---|---|---|---|---|---|---|---|---|---|---|---|---|---|---|---|---|---|---|---|---|---|---|
|
Additives
Labor analgesia is often provided with intrathecal and/or epidural opioids added to the local anesthetic. The inclusion
of opioids in labor analgesia solutions allows for the use of lower local anesthetic concentrations, thus decreasing motor weakness and the incidence of instrumental vaginal delivery (20,21). Fentanyl added to spinal anesthesia for cesarean delivery increases intraoperative comfort, decreases need for intraoperative analgesic supplementation, and helps prevent intraoperative nausea (22,23,24).
of opioids in labor analgesia solutions allows for the use of lower local anesthetic concentrations, thus decreasing motor weakness and the incidence of instrumental vaginal delivery (20,21). Fentanyl added to spinal anesthesia for cesarean delivery increases intraoperative comfort, decreases need for intraoperative analgesic supplementation, and helps prevent intraoperative nausea (22,23,24).
As explained above, local anesthetics are weak bases with pKa’s higher than 7.4, and so tend towards ionization at physiologic pH. Many commercial local anesthetic preparations are formulated as hydrochloride salts for stability, and are therefore acidic, favoring ionization. The addition of bicarbonate to the local anesthetic solution adjusts pH of the local environment closer to pKa, thus favoring non-ionization and hastening speed of onset of sensory blockade which can be advantageous in emergent situations.
Addition of epinephrine to spinal anesthetics increases density and duration of block, and slows block regression time (25,26,27). When epinephrine is added to epidurally administered drugs, onset is faster, analgesia is more dense, and duration is longer (28,29). Explanations for this are twofold: Epinephrine’s α-2-agonism directly enhances neuraxial anesthesia (30). In addition, epinephrine is vasoconstrictive and decreases clearance from nonneural tissues, thus amplifying blockade and prolonging duration of blockade (30). Inclusion of epinephrine in epidural lidocaine or bupivacaine solutions reduces peak plasma concentrations of the agents (31). Addition of epinephrine to labor analgesia solutions may intensify motor block (32). Phenylephrine is no longer used as a vasoconstrictor additive due to its association with transient neurologic symptoms (TNS) (33).
Use of the α2-agonist clonidine with local anesthetics enhances intrathecal and epidural labor analgesia; however, it increases maternal hypotension and sedation (34,35,36). Addition of neostigmine to labor analgesia regimens is associated with high rates of nausea and vomiting and its routine use is not recommended (37,38). The benefits of additives must be weighed against increased costs and a theoretical potential for error when multiple medications are administered.
Effect of Pregnancy
It has been observed that women in the second and third trimesters of pregnancy experience higher cephalad blockade in response to intrathecal hyperbaric local anesthetics than nonpregnant women and it has been suggested that the same effect is also seen after epidural administration of local anesthetics (39,40). The effects of pregnancy on local anesthetic action are twofold: (1) Anatomical changes in the central neuraxiom favor cephalad spread of injected local anesthetics. (2) In addition, neuronal susceptibility to local anesthetic blockade increases.
Magnetic resonance imaging reveals that epidural vein engorgement which occurs during pregnancy due to vena cava compression by the gravid uterus causes posterior displacement of the vertebral canal, resulting in a decrease in cerebrospinal fluid volume (41,42). Administration of standard local anesthetic doses into this smaller intrathecal volume results in a greater dermatomal spread and a higher block. Vein engorgement also impedes leakage of injected epidural solutions through the foramina, thus promoting longitudinal spread of epidural administered medications (43).
In addition to these anatomical factors, the nerves per se appear to have heightened sensitivity to local anesthetic action during pregnancy. Parturients demonstrate intensified blockade of the median nerve at the wrist compared to nonpregnant women who receive the same dose of lidocaine (44). In vitro studies reveal that nerves from pregnant animals are more quickly and easily blocked by local anesthetics than those from nonpregnant animals (45,46). This effect is not due to differences in local anesthetic neuronal uptake and is presumed to be a pharmacodynamic effect, possibly mediated by progesterone (47,48). The acid–base changes that occur in cerebrospinal fluid during the pregnancy probably exert little effect (49). Protein binding of local anesthetics in CSF is not altered in pregnancy.
Pharmacokinetics
Understanding the pharmacokinetics of local anesthetics requires knowledge of the principle of absorption as well as the commonly discussed pharmacokinetic variables governing elimination (8,50). Since local anesthetics are placed regionally, absorption from the site of injection must be taken into account when discussing drug elimination. The maximal serum concentration after administration (Cmax) and the time at which Cmax occurs (tmax) characterize absorption. Absorption is dependent on local blood flow which varies by site of injection in the following order (greatest blood flow/highest absorption to least blood flow/lowest absorption): Intercostal > caudal > epidural > peripheral nerve block > subcutaneous injection. The addition of vasoconstrictors decreases Cmax and may prolong tmax and one may expect that this effect is most pronounced in highly vascular tissues such as the epidural space (31). Absorption is also dependent upon local tissue binding: As stated above, drugs that are soluble into perineural lipids and/or tightly bound to perineural proteins demonstrate slower rates of absorption (lower Cmax and longer tmax) (8,9). Systemic uptake from the epidural space is biphasic: A rapid uptake phase is followed by a longer, slower phase, the latter phase being relatively more prolonged for highly lipid soluble drugs such as bupivacaine than the less lipid soluble lidocaine (51). Continued absorption into the intravascular compartment offsets elimination as compared to that which occurs during intravenous administration of the drug (51). If rate of absorption from site of injection is slower than t1/2 (as is for clinically relevant local anesthetics and injection sites) then it is more useful to
discuss mean body residence time (how long the medication stays in the body) than serum t1/2.
discuss mean body residence time (how long the medication stays in the body) than serum t1/2.
Serum elimination t1/2 varies directly with volume of distribution (Vd) and indirectly with clearance. Vd is related to the relative amounts of plasma and blood cell binding versus tissue binding, and is relatively low for the highly protein bound bupivacaine. Clearance differs for amino-ester versus amino-amide local anesthetics. The esters are quickly hydrolyzed by pseudocholinesterase and other plasma esterases. Despite the decrease in pseudocholinesterase during pregnancy the serum half-life of 2-chloroprocaine in vitro is 11 seconds. The half-life of 2-chloroprocaine injection after epidural administration is longer and ranges from 1.5 to 6.4 minutes because of continued absorption from the site of administration (52,53).
In contrast, the amide local anesthetics are metabolized in the liver over several hours. Lidocaine, with a particularly high hepatic extraction ratio, demonstrates a clearance predominantly determined by hepatic blood flow and the elimination half-life of lidocaine following epidural administration during pregnancy is 114 minutes (54). However, the majority of amino-amides possess intermediate hepatic extraction ratios, and thus clearance depends on both hepatic blood flow and enzymatic activity (50).
Serum levels of bupivacaine and ropivacaine increase over time during prolonged epidural infusion (55,56). This may be partially offset by increases in protein binding that occur in the postoperative period, so that free drug concentrations remain stable (57,58).
Pregnancy decreases both Vd and clearance, such that t1/2 remains unchanged (59). Thus, inadvertent intravascular administration of a large dose of local anesthetic to a parturient might result in a higher initial serum concentration but unchanged t1/2.
Placental Transfer and Effects on Blood Flow and the Fetus
Local anesthetics rapidly cross the placenta and can be found in fetal serum (60). In the case of fetal acidemia, maternally administered and absorbed local anesthetic could become ionized and “trapped” in the fetal circulation. It seems prudent to avoid lidocaine in the setting of fetal academia, as it has more potential for placental passage. Bupivacaine is found in only very low concentrations in the umbilical vein, even after several hours of epidural administration (61). Since chloroprocaine is cleared from the circulation so rapidly, its potential for accumulation in the fetus is virtually nonexistent (52,53).
Animal studies demonstrate no effect of local anesthetics on uterine artery blood flow at clinically relevant doses (60). Clinical studies confirm that epidural administration of either bupivacaine or ropivacaine does not cause changes in either uterine artery or umbilical artery Doppler blood flow (62). In contrast, toxic doses of local anesthetics, as might occur during inadvertent intravascular injection may increase uterine tone, thereby decreasing uterine blood flow.
With the exception of cocaine, local anesthetics are not considered teratogenic in man at clinically relevant doses.
Local Anesthetic Systemic Toxicity
Local anesthetic systemic toxicity (LAST) remains a feared complication of regional anesthesia. When local anesthetic drugs are accidentally injected or otherwise absorbed into the intravascular space in toxic amounts, the result can be devastating due to effects on the central nervous and cardiovascular systems. Unintentional venous injection may be associated with a rapid onset of seizure activity and may be more likely during administration of epidural anesthesia in pregnancy secondary to epidural vein engorgement. In contrast, when toxicity results from slow absorption of local anesthetic after regional administration, one might expect a 20 to 30 minute delay in symptoms, and for serum concentrations of the anesthetic to remain elevated for a longer period of time.
Incidence
A large surveillance study done in France investigating serious adverse reactions related to regional anesthesia detailed 98 complications, 24 of which involved seizure activity and most likely represented LAST (63). None of these 24 likely cases of LAST were accompanied by cardiac arrest or death. The authors estimated the risk of LAST at 1.3 per 10,000 epidural anesthetics. A more recent survey by the same group confirmed these numbers (1.8 per 10,000 epidural anesthetics and 0.7 per 10,000 obstetric epidural anesthetics) and once again, toxicity was manifested by seizures without cardiac arrest (64). A recent examination of anesthesia-related maternal mortality in the United States revealed a risk of 3.8 deaths per million regional anesthetics administered for cesarean delivery, including 0.7 per million which were due to a “drug reaction”—presumably LAST (65). The incidence of LAST is declining in both the general and obstetric populations (65,66,67). The most recent obstetric anesthesia closed claims analysis included no cases of malpractice payment on behalf of an anesthesiologist due to LAST (68). Authors attribute this decline to the withdrawal of 0.75% bupivacaine from the market for obstetric use, an increased awareness of LAST, increased use of safety measures such as test dosing and slow incremental injection, and new treatment options with lipid emulsion solutions (65).
Clinical Presentation
Symptoms of LAST are due to blockade of voltage-gated Na+ channels in the central nervous system (CNS) and cardiovascular system. Symptoms are dose dependent (Table 8-3). CNS effects reflect local anesthetic action on the brain: At low serum concentrations, local anesthetics inhibit the inhibitory neurons in the CNS, and thus a period of excitation ensues. The patient may experience tongue numbness and a vague lightheadedness or dizziness, followed by agitation and muscular twitching, and eventual generalized tonic–clonic seizure activity. At higher serum levels, generalized CNS inhibition occurs, which is manifested by coma and depression of brainstem cardiorespiratory centers, and may result in death.
Local anesthetics bear both direct and indirect effects on the cardiovascular system: Direct effects include myocardial depression; indirect effects are mediated through the CNS and are biphasic such that during the CNS excitatory phase sympathetic activation occurs and during the CNS depressant phase, vasomotor depression appears (69,70,71,72). The end result is that at subconvulsant doses of local anesthetics, one sees a small decrease in cardiac contractility, cardiac output, and systemic blood pressure. At convulsant levels, increases in heart rate, contractility, cardiac output, systemic vascular resistance, and blood pressure occur. Supraconvulsant concentrations are followed by decreases in contractility, cardiac output, and systemic blood pressure. In addition, bradycardias and wide complex arrhythmias develop.
Local anesthetics bear both direct and indirect effects on the cardiovascular system: Direct effects include myocardial depression; indirect effects are mediated through the CNS and are biphasic such that during the CNS excitatory phase sympathetic activation occurs and during the CNS depressant phase, vasomotor depression appears (69,70,71,72). The end result is that at subconvulsant doses of local anesthetics, one sees a small decrease in cardiac contractility, cardiac output, and systemic blood pressure. At convulsant levels, increases in heart rate, contractility, cardiac output, systemic vascular resistance, and blood pressure occur. Supraconvulsant concentrations are followed by decreases in contractility, cardiac output, and systemic blood pressure. In addition, bradycardias and wide complex arrhythmias develop.
Table 8-3 Dose-dependent Symptoms of Lidocaine Toxicity | ||||||||||||||
---|---|---|---|---|---|---|---|---|---|---|---|---|---|---|
|
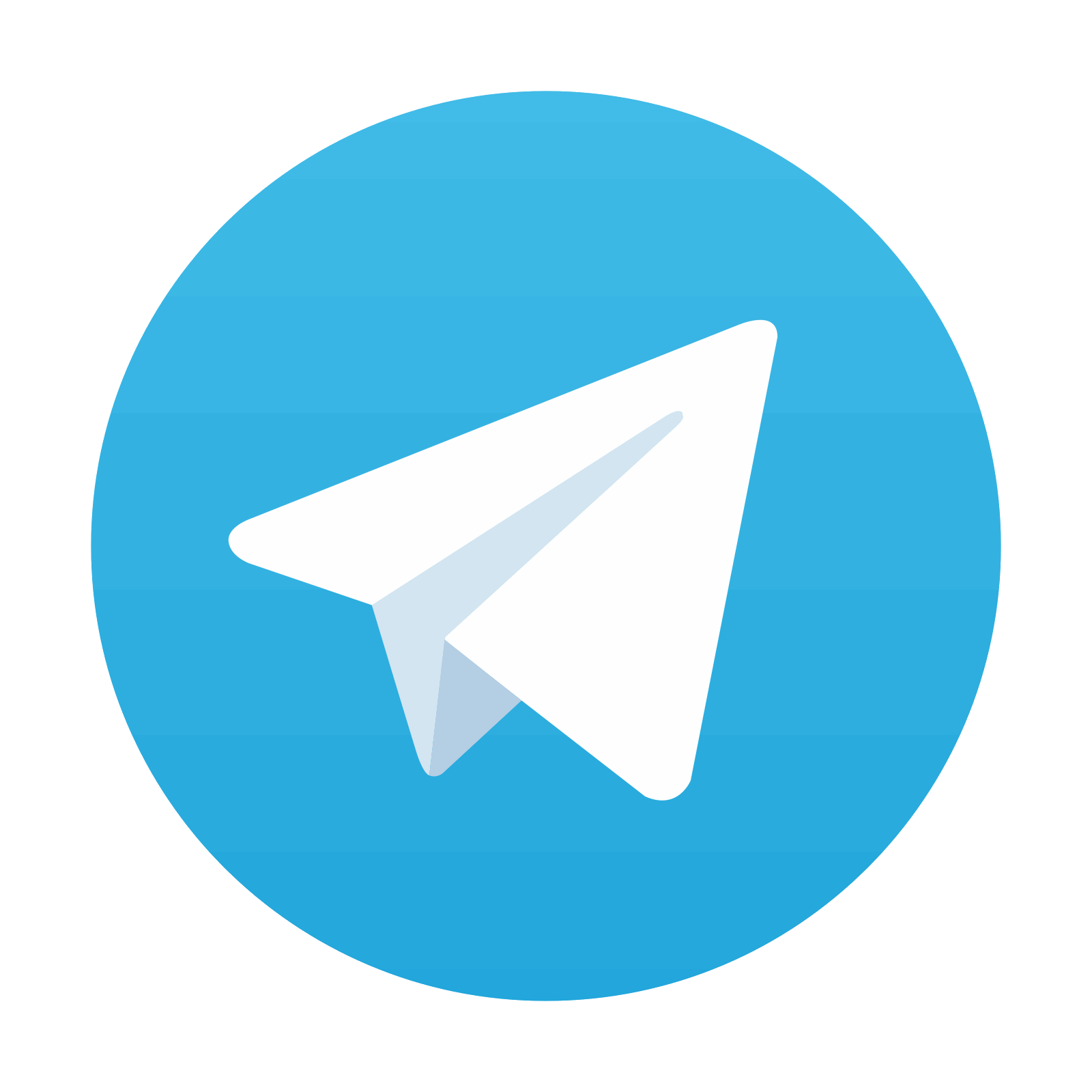
Stay updated, free articles. Join our Telegram channel

Full access? Get Clinical Tree
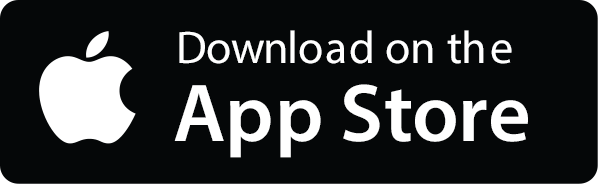
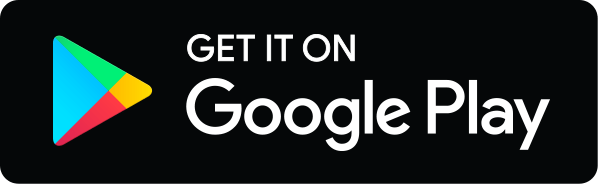