Agent
Spinal
Epidural
Nerve block
Durationa
Lidocaine
Can be used
2 %, 15–30 ml
2 %, 30–50 ml
1–1.5 h
Ropivacaine
Can be used
0.2 %, 10–20 ml
0.5 %, 30–50 ml
3–4 h
Bupivacaine
0.75 %, 1–2 ml
0.25 %, 10–20 ml
0.25 %, 30–50 ml
3–4 h
Mepivacaine
Can be used
Can be used
1.5 %, 30–50 ml
2–3 h
Chloroprocaine
Not used currently
3 %, 15–30 ml
Can be used
0.5–1 h
Anatomy and Physiology of Nerve Conduction
The fundamental unit of excitable tissue is the nerve cell (Fig. 18.1). The major parts of the nerve cell include the cell body, the nucleus, dendrites, and the axon. The lipoprotein nerve cell membranes of the axon and to a lesser degree the dendrites are involved in electrical activity. Most of the axons in the body are covered with a discontinuous insulating substance, called myelin (Fig. 18.2), with gaps determined by the size and function of the nerve (nodes of Ranvier).



Fig. 18.1
Anatomy of a nerve cell

Fig. 18.2
The myelinated axon
The physiological basis for nerve conduction is the movement of sodium and potassium ions across the axonal membrane through ion channels (Fig. 18.3). Sodium channels exist in three states: resting, activated (open), or inactivated. The sodium channels allow movement of sodium only in the open state, while potassium moves freely, achieving electrical neutrality and determining the electrical charge intracellularly, with sodium restricted to the extracellular side when the sodium channels are closed. The result is that sodium is the predominant extracellular cation and potassium the predominant intracellular cation. Following chemical, mechanical, or electrical stimuli, movement of sodium occurs into the cell via open sodium channels causing depolarization. At a given threshold (−55 mV) an action potential occurs and this segment of the axon causes depolarization of adjacent axonal membrane (neural conduction). The electrical neutrality is rapidly restored by egress of potassium outside the cell, inactivation of voltage-gated sodium channels, and the balance restored by energy-dependent sodium/potassium ATPase (transports three sodium ions out of the cell for every two potassium ions it transports inside the cell). In axons covered with myelin (myelinated nerves), the depolarization occurs at the nodes of Ranvier, with sodium movement at one node causing opening of the sodium channels at the adjacent node.


Fig. 18.3
Sodium channel, potassium movement, and the sodium–potassium ATPase pump
Conduction block occurs when this process is interrupted by sodium channel blockade, which can be reversible or nonreversible. Clinical conduction block with local anesthetics occurs exclusively in the reversible group, with irreversible block occurring with pesticides and animal venom. All reversible sodium channel block occurs on the intracellular side of the sodium channel (Fig. 18.4). For physiologic reasons, local anesthetics are delivered on the extracellular side, because intraneural injection into the axon would cause damage to the nerve cell membrane. This means that the molecule chosen must be capable of diffusing across the axonal membrane (lipid solubility), and occupy the open face of the sodium channel on the intracellular side (ionic bonding). The crossing of the neural membrane occurs at the Nodes of Ranvier in myelinated axons, and blockage of consecutive nodes increases the probability of conduction block. This is the reason why conduction block occurs quickly in smaller, unmyelinated (C) nerve fibers and slowest in the larger myelinated (A) nerve fibers (Table 18.2).


Fig. 18.4
Mechanism of action of local anesthetics
Table 18.2
Peripheral nerve fiber types
Fiber type | A-alpha | A-beta | A-gamma | A-delta | B | C |
---|---|---|---|---|---|---|
Mode of conduction | Motor, proprioception | Light touch, pressure, proprioception | Motor | Pain, cold temperature, touch | Autonomic | Pain, temperature, touch |
Size | 4+ | 3+ | 2+ | 2+ | 1+ | 1+ |
Myelination | Yes | Yes | Yes | Yes | Yes | No |
Organic Chemistry of Local Anesthetic Molecules
The prototype local anesthetic agent has four structural elements that contribute to function (Fig. 18.5). They are all amphipathic molecules, which means that within the molecule there are elements with different purposes. All local anesthetics are weak bases, manufactured and stored at acid pH as sodium (or carbonate) salts. The largest element is the hydrophobic side of the molecule which creates lipid solubility. On the opposite side of the molecule is the hydrophilic element, allowing for ionic activity. The hydrophilic side is a tertiary amine for all commercially available local anesthetics except benzocaine (a secondary amine). The hydrophobic and the hydrophilic elements are linked via an intermediate chain of between 3 and 7 carbon equivalents in size. Within the intermediate chain is a bond (either amide or ester) that determines the type of molecule and its metabolism in the body. The amino amides originate from and are metabolized to anilines whereas the amino esters are related to para-aminobenzoic acid (PABA).


Fig. 18.5
Chemical structure of local anesthetics
Lipid solubility is determined by the size of the hydrophobic element of the molecule, but also by aliphatic substitutions on the intermediate chain and the hydrophilic element. Lipid solubility determines the potential for the molecule to cross the axonal membrane, and hence determines the potency and toxicity. More lipid solubility means more potency and potential for central nervous system (CNS) toxicity.
Protein binding is an indirect measure of the potential for the molecule to remain embedded in the substance of the axonal membrane. The greater the protein binding affinity of a given local anesthetic, the longer the duration of conduction block with that agent. Because lipid solubility is an important determinant of protein binding, potency and duration of local anesthetics are usually similar, that is, highly potent agents also have a long duration of conduction block.
The pKa of the molecule is determined by the tertiary amine, the ionic signature. The pKa is the pH for a given molecule at which the cationic (ionic) and base (unionized) forms are in equal concentrations. Commercial local anesthetics have pKa between 7.6 and 9.3 and are manufactured as sodium salts at an acid pH (5.0–5.5, unless manufactured with epinephrine which requires storage at a pH less than 3.0 to prevent spontaneous hydrolysis of the epinephrine). In the bottle/vial, the majority of the molecules are cationic (>1,000:1).
Properties of Local Anesthetics
The properties of local anesthetics that describe their unique clinical characteristics include speed of onset of action, potency, duration of action, and toxicity.
Onset of action of a local anesthetic can be enhanced by using a higher dose, higher concentration, and a pKa which is close to the physiologic pH (more availability of the unionized form). Speed of onset of conduction block is the time from injection of the solution to interruption of neural activity. This is determined by the need for the solution to be injected extracellularly, the time for a substantial number of molecules to cross the axonal membrane and occupy the sodium channels. The solution injected is predominately cationic, and the cation has very limited potential to cross the lipid membrane. Extracellular buffering (mostly bicarbonate) raises the pH from 5.0 to physiologic, where the cation/base ratio increases to about 70:30. Some of the base then begins to cross the cell membrane to the intracellular side. Once on the intracellular side, the base rapidly converts to the cationic state because of the more acidic intracellular pH, which rapidly occupies the sodium channels. The latency to onset is determined first by the distance of injection to the nerve cell membrane (accuracy of the person performing the block, and the hydrophilic properties of the agent), and secondly by the cation/base ratio. The higher the concentration of the base, the faster the onset of conduction block. Since the base concentration at acid pH is less as the pKa increases, the speed of onset of action is inversely proportionate to pKa. For example, the pKa of lidocaine is 7.6 and bupivacaine is 8.3, with lidocaine having a substantially increased speed of onset of action. This is also the physiologic basis for pre-block alkalinization of a local anesthetic solution with bicarbonate to increase the speed of onset of action.
Potency of a local anesthetic is directly related to the amount of the base that ultimately crosses the axonal membrane, and to the lipid solubility of the base form of the agent. The potency increases in direct proportion to lipid solubility. The local anesthetic with the least lipid solubility is procaine and it has the lowest potency. Conversely, bupivacaine has the highest lipid solubility and is the most potent local anesthetic available commercially.
The duration of action of a local anesthetic is determined by the affinity of the agent to remain embedded in the axonal membrane. The axonal membrane is a hydrophobic, lipoprotein environment. The duration of a given agent is best estimated by the protein binding of the agent, although it is also influenced by lipid solubility. As protein binding increases, so does the duration of action. In general, as the lipid solubility increases, so does protein binding and hence duration of action of the local anesthetic. For example, the least protein bound agent is 2-chloroprocaine, which also has the shortest duration of action. Conversely, etidocaine and bupivacaine have the highest protein binding potential and also the longest duration of action.
System Effects and Toxicity of Local Anesthetics
Local anesthetics besides being available as injectable solutions are also available for topical application of eyes (absorbed via the mucous membranes), and for dermal anesthesia (EMLA cream). Systemic absorption of local anesthetics is determined by site of injection, dose, addition of vasoconstrictor, and pharmacologic profile of the local anesthetic. The uptake of local anesthetic into the blood from greatest to least is IV > tracheal > intercostal > caudal > paracervical > epidural > brachial > sciatic > subcutaneous. Toxicity of local anesthetics is directly related to the potency and lipid solubility of the drug. More highly toxic drugs have a smaller gap between the therapeutic and the toxic dose (therapeutic index).
Cellular Effects
Cytotoxicity of local anesthetic solutions is related to the pH injected and the direct cellular impact after injection. At some concentration, all local anesthetics are cytotoxic, and this limits the upper concentration available for clinic use. 2-chloroprocaine has the highest potential for cytotoxicity, because of low pH, use of preservatives, and other unknown mechanisms.
Neurological Effects
Neurotoxicity of local anesthetics occurs when local anesthetic accumulates in the central nervous system (CNS), particularly the limbic brain, where inhibitory neurons are blocked at a lower concentration than excitatory, with the result being excitation, agitation, uncontrolled motor activity, and at some concentration, seizure activity. The most potent agents, bupivacaine and etidocaine, are agents with the greatest potential for neurotoxicity. CNS impact occurs from the free form of the local anesthetic (non-protein bound), which does not accumulate until protein binding capacity is exceeded, and then does so rapidly. This means that the same agent will be more neurotoxic with faster accumulation in the blood, as in intravascular injection or when the agent is injected into a highly vascular area, such as the intercostal or the caudal epidural space. It also means that there will be less toxicity when vasoconstrictors (epinephrine) are mixed with the solution, reducing vascular uptake. In general, the toxicity of the esters is less than the amides because of the rapid metabolism of the ester bond by cholinesterase. The amides are metabolized in the liver prior to elimination and have much longer half-lives.
With the unbound form of local anesthetic increasing in the CNS, the first manifestation is excitation, originating within the limbic system, including agitation, tremor, and uncontrolled motor activity. Because of the proximity of the cell bodies of the cranial nerves in the brainstem, paresthesia (tingling of the face, numbness on the tongue), spots before the eyes, or ringing in the ears is also reported. Tremor and involuntary motor activity (muscle twitching) can follow, and if progression continues, seizure activity can occur. Toxicity is potentiated by hypoxia, hypercarbia, and acidosis, and as a result, early effective resuscitation is important. Raising the seizure threshold with barbiturates or benzodiazepines is also an option to prevent or treat seizure activity. It is important to limit the motor activity associated with seizures as it greatly increases oxygen demand while reducing the potential for oxygen delivery.
Transient neurological symptoms (TNS) are defined as symmetrical bilateral pain in the back or buttocks or pain radiating to the lower extremities after recovery from spinal anesthesia. TNS is thought to occur with using highly concentrated solutions of the local anesthesia for spinal anesthesia. The concentrated solution causes localized inflammation and irritation of the nerve roots. Pain can be treated with opioids and or NSAIDS, and muscle spasms treated with a muscle relaxant, with resolution of symptoms usually in 1–2 weeks. Incidence of TNS is greatest with lidocaine > mepivacaine > ropivacaine > bupivacaine. Other risk factors for TNS include multiple attempts for spinal anesthesia, use of a cutting-edge needle (Quinke), obesity, and lithotomy position for surgery.
Continuous spinal anesthesia, especially using small bore micro-catheters, may cause cauda-equina syndrome (irritation and damage of spinal nerve roots by localized highly concentrated local anesthetic solution). These micro-catheters are no longer used; however, caution still should be used while administering local anesthetics via continuous spinal anesthesia. Bupivacaine appears to be more safer, in this respect, than lidocaine for continuous spinal anesthesia.
Cardiac Effects
All local anesthetics have effects on the heart at some concentration. These effects include a decrease in myocardial contractility, conduction velocity, myocardial automacity, and the duration of the refractory period. Blockage of cardiac sodium channels by the local anesthetic produces these effects. This leads to bradycardia, hypotension, heart block, and even cardiac arrest. About two or three times the blood levels of local anesthetic that produce seizures are required to produce major cardiac toxicity.
While lidocaine in low doses is used to treat ventricular arrhythmias, highly lipid-soluble agents, such as bupivacaine and etidocaine, more commonly cause cardiac toxicity. Unlike lidocaine, which enters and exits the cardiac sodium channels rapidly, bupivacaine and etidocaine enter rapidly and exit more slowly, predisposing to accumulation and selective cardiac toxicity. This occurs when the primary conduction system is blocked and reentrant pathways activated, with the potential for non-perfusing reentrant arrhythmia, such as ventricular tachycardia and ventricular fibrillation. In addition, the more rapid the heart rate, the greater is the accumulation of bupivacaine. Cardiac toxicity is undoubtedly a lipid solubility property, because mepivacaine, which has significant lesser toxicity, only differs in the substitution of a butyl (4-carbon) for a methyl (1-carbon) on the tertiary amine. This may also explain the mechanism of intralipid rescue from bupivacaine cardiac toxicity.
Treatment of local anesthetic toxicity includes stopping the injection of the local anesthetic, airway management, treatment of seizures (diazepam 5–10 mg, midazolam 2–4 mg, propofol 50 mg)), cardiopulmonary resuscitation (treatment of hypotension or arrhythmias—do not use lidocaine), and administration of 20 % intralipid for severe or refractory toxicity (1.5 ml/kg bolus and then 0.25–0.5 ml/kg/min).
Methemoglobinemia
Some local anesthetics, such as prilocaine and benzocaine, can cause the oxidation of the iron in hemoglobin from ferrous (FE2+) to ferric (FE3+) causing methemoglobinemia. When the amount exceeds a threshold (about 4 g/dl), cyanosis becomes visible. This is clinically innocuous in healthy patients, but the cyanosis and lower saturation readings are not easily distinguished from hypoxemia. In patients with diminished pulmonary reserves, the reduced oxygen carrying capacity can be symptomatic, and can be treated with methylene blue (1 mg/kg), with rapid reversal.
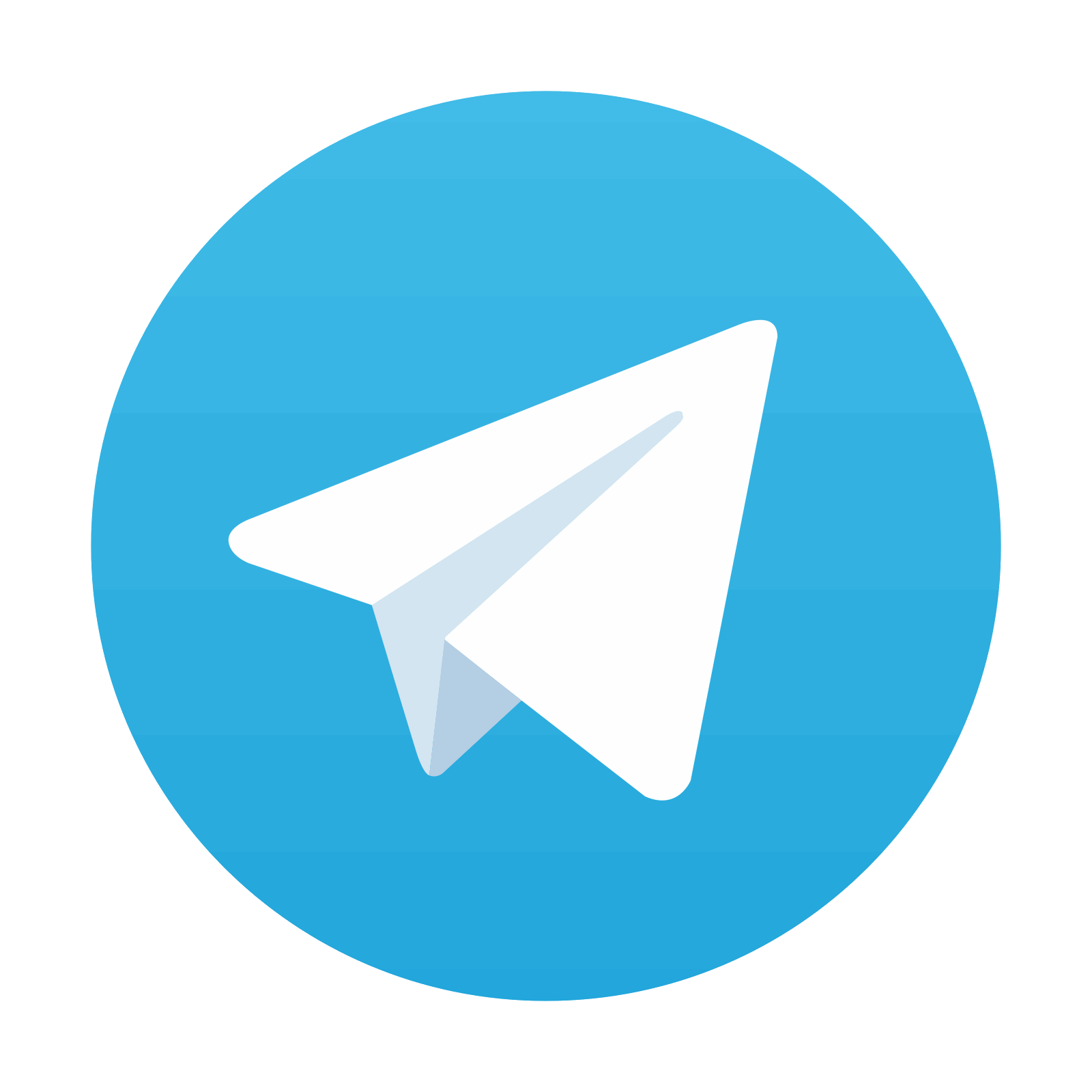
Stay updated, free articles. Join our Telegram channel

Full access? Get Clinical Tree
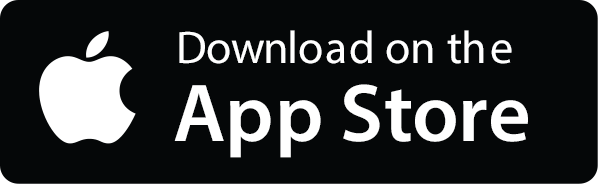
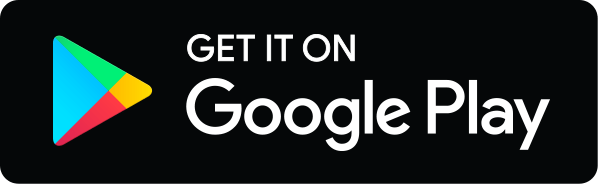