FIGURE 72.1 Graft failure among adult recipients of livers transplanted from deceased donors, 2012. (Adapted from 2012 Annual Report of the U.S. Organ Procurement and Transplantation Network and the Scientific Registry of Transplant Recipients: Transplant Data 1994–2003. Department of Health and Human Services, Health Resources and Services Administration, Healthcare Systems Bureau, Division of Transplantation, Rockville, MD; United Network for Organ Sharing, Richmond, VA; University Renal Research and Education Association, Ann Arbor, MI. http://optn.transplant.hrsa.org.)

FIGURE 72.2 Half-lives for adult recipients of livers transplanted from deceased donors, 2012. (Adapted from 2012 Annual Report of the U.S. Organ Procurement and Transplantation Network and the Scientific Registry of Transplant Recipients: Transplant Data 1994–2003. Department of Health and Human Services, Health Resources and Services Administration, Healthcare Systems Bureau, Division of Transplantation, Rockville, MD; United Network for Organ Sharing, Richmond, VA; University Renal Research and Education Association, Ann Arbor, MI. http://optn.transplant.hrsa.org.)

FIGURE 72.3 Recipients alive and with a functioning liver transplant as of June 30, 2012. (From 2012 Annual Report of the U.S. Organ Procurement and Transplantation Network and the Scientific Registry of Transplant Recipients: Transplant Data 1994–2003. Department of Health and Human Services, Health Resources and Services Administration, Healthcare Systems Bureau, Division of Transplantation, Rockville, MD; United Network for Organ Sharing, Richmond, VA; University Renal Research and Education Association, Ann Arbor, MI. http://optn.transplant.hrsa.org.)
Cardiovascular complications are the leading cause of non–graft-related mortality and morbidity following LT in the acute transplant period and remain the number one cause of 1-year mortality (13). Therefore, an important element of the pretransplant evaluation is the assessment of the cardiovascular system. LT is a hemodynamically challenging procedure and individuals must have adequate cardiovascular reserve to tolerate the surgery.
Essentials of the Cardiac Evaluation
In addition to a thorough history and physical examination, adult patients undergoing evaluation for LT should have an ECG, looking for findings that suggest the presence of underlying ischemic, conductive, or structural cardiac disease; if present, this should prompt further testing. Transthoracic echocardiography (TTE) is an excellent noninvasive test that should be considered in all patients under consideration for LT. Much information can be garnered from a TTE including, but not limited to, diastolic and systolic ventricular function, presence of cardiomyopathies, structural defects, valvular abnormalities, evidence of an intrapulmonary shunt on the bubble contrast study, and the presence of pulmonary artery (PA) hypertension. There are several significant cardiopulmonary conditions associated with ESLD outlined below which can be safely ruled out on a screening TTE.
TABLE 72.1 Model for End-Stage Liver Disease (MELD) Scoring Systema |
![]() |

FIGURE 72.4 Estimated 3-month survival as a function of MELD score. (Adapted from Wiesner R, Edwards E, Freeman R, et al; The United Network for Organ Sharing Liver Disease Severity Score Committee. Model for end-stage liver disease (MELD) and allocation of donor liver. Gastroenterology. 2003;124(1):91–96.)
Coronary Artery Disease. It has been reported that the prevalence of CAD in patients with ESLD approaches 30%, clearly exceeding that of the general population (14,15). This is at least in part due to the increasing age of patients receiving LT combined with comorbidities considered risk factors for CAD. In fact, the number of recipients older than 65 years has more than doubled from 2002 to 2012 (11,16). Chronic HCV infection is also independently associated with presence of metabolic conditions—insulin resistance, type 2 diabetes mellitus, and hypertension—which are established risk factors for CAD (17). Patients with nonalcoholic steatohepatitis (NASH), in particular, are more likely to be older, obese, hypertensive, diabetic, have chronic kidney disease, and have hyperlipidemia or metabolic syndrome, all risk factors for CAD, and to suffer posttransplant cardiovascular events (18,19). In fact, growing evidence suggests that NASH itself is an independent risk factor for cardiovascular disease (20). LT candidates with two or more of the following traditional cardiac risk factors (21) are more likely to have obstructive CAD (11,19,22–25):
- Age: male 45 or older, female 55 or older or premature menopause without estrogen replacement therapy
- Family history of premature coronary disease: definite myocardial infarction or sudden death before age 55 years in male first-degree relative and before age 65 in female first-degree relative
- Current cigarette smoking
- Hypertension: blood pressure over 140/90 mmHg, or an antihypertensive medication
- HDL cholesterol below 40 mg/dL (1.03 mmol/L)
The presence of even nonobstructive CAD has been shown to negatively impact short and long-term mortality outcomes in LT (26–31). Therefore, it is important to identify and treat patients at risk for CAD prior to LT given the risk of perioperative cardiac complications (29,32,33); in some cases the burden of CAD may be severe enough to prohibit LT.
Approximately one-fourth of LT candidates with traditional coronary risk factors may have developed clinically significant CAD even while asymptomatic. As patients with ESLD often are sedentary and encephalopathic, typical signs of myocardial ischemia may be masked. Extrapolating from currently accepted preoperative, cardiac evaluation guidelines created for the general population that place emphasis on symptomatology may not be prudent in the setting of LT (34,35).
The evaluation for the presence of inducible ischemia with noninvasive exercise or pharmacologic stress testing has limited predictive value in LT candidates (36). The inability to achieve target heart rate or adequate peak double product due to impaired adrenergic and chronotropic responses is associated with an increased risk of postoperative cardiovascular events (37). In the ICU setting, hemodynamic instability, use of vasopressors, mechanical ventilation (MV), and kidney injury make noninvasive stress testing impractical and unlikely to yield findings worthy of interpretation. The decision to pursue stress testing should be based on individualized evaluation of the candidate’s pretest probability for having CAD.
Coronary angiography (CA) should be considered for LT candidates with high pretest probability of CAD (two or more traditional coronary risk factors) those who cannot undergo stress testing, have suboptimal response to pharmacologic stress testing, or who have inducible ischemia on stress imaging. CA can be performed safely in LT candidates even with renal dysfunction and elevated bleeding risk (38–40). CA via the transradial approach, if possible, is the preferred method in LT candidates, to improve hemostasis and reduce periprocedural complications (41–43).
Revascularization of obstructive CAD may be pursued in order to improve symptoms and cardiovascular mortality per ACC/AHA guidelines, and in cases where the burden of obstructive CAD would prohibit LT in an otherwise appropriate surgical candidate (36,44–46). There is evidence suggesting revascularization will improve post-LT outcomes (47,48). Increases in the frequencies of CA and percutaneous coronary intervention (PCI) corresponded to significant reductions in postoperative MIs and 1-year all-cause mortality rates in LT through reductions in the incidence of both fatal and nonfatal MIs (36,49).
Cardiomyopathy. Cirrhotic cardiomyopathy is a physiologic syndrome unique to patients with ESLD. Despite a hyperdynamic circulation, there is both clinical and experimental evidence that liver failure itself can lead to impaired cardiac function. Cirrhotic cardiomyopathy is a syndrome of diminished chronotropic and inotropic response to stress and diastolic and/or systolic dysfunction. Diastolic dysfunction, which limits passive and active filling of the LV, is the most common clinical feature present in cirrhotic cardiomyopathy (50,51). LV systolic function is typically normal at rest, but inadequate reserve with a decreased stroke volume in response to stress is the hallmark of this entity (51–57). This impaired inotropic response, combined with the diminished capacity for a chronotropic response in patients with ESLD limits cardiac reserve during times of stress.
A common electrophysiologic abnormality associated with cirrhotic cardiomyopathy is a prolonged QT interval on ECG. A rate-corrected QT interval of greater than 440 ms has been estimated to be present in 30% to 60% of patients with cirrhotic cardiomyopathy (58). However, the incidence of sudden cardiac death in patients with cirrhosis who develop a pronged QT interval does not seem to be elevated (59–61). The clinical significance of a prolonged QT interval associated with cirrhotic cardiomyopathy remains unknown, but drugs that prolong ventricular repolarization should be avoided.
There is no standard treatment for cirrhotic cardiomyopathy. Down regulation of β-adrenergic receptors and attenuation of response to chronotropic and inotropic drugs is a common feature of this entity and, therefore, β-agonists may not elicit the expected response (62–64). β-Blockers may be beneficial as they improve QTc interval prolongation and may oppose the downregulation of adrenoreceptor density. However, it is unknown if they have an effect on long-term contractile function in cirrhotic cardiomyopathy (65–68). Mineralocorticoid antagonists (aldosterone) and angiotensin-converting enzyme (ACE) inhibitors have not shown long-term benefit in cirrhotic cardiomyopathy (65,69). Fortunately, there is strong evidence that LT reverses the majority of cirrhotic cardiomyopathy (70–73). After LT, heart function parameters improve and the QT interval normalizes in the majority of patients (74). Nonetheless, immediate perioperative stresses may precipitate myocardial dysfunction in the perioperative period (75).
Cardiomyopathies are myocardial diseases presenting as structural or functional disorders of the heart. The prevalence of secondary causes of cardiomyopathy is significantly higher in LT candidates compared with the general population. Certain indications for LT, such as alcoholism, hemochromatosis, and amyloidosis, may also have both direct and indirect toxic myocardial effects. In fact, alcohol is the main cause of nonischemic, dilated cardiomyopathy in the western world (76,77). Careful pretransplant cardiac evaluation is essential and diagnosis of cardiac involvement in these disorders requires a high index of clinical suspicion and coordinated multidisciplinary evaluation for LT candidacy. Decompensated congestive heart failure can be difficult to appreciate as peripheral edema, dyspnea, orthopnea, and elevated jugular venous pressure are frequent findings of ESLD.
The presence of preoperative LV dysfunction is not an absolute contraindication to LT per se, but is a risk factor for perioperative cardiovascular complications and mortality. Most centers restrict LT to those with LV ejection fraction (EF) over 40%; there is general consensus that patients with worse function do not have the reserve to withstand the rigorous hemodynamic insults associated with LT (78). As the typical pretransplant hemodynamic profile is one of low SVR, cirrhotics are considered to be “auto-afterload reduced” and LV systolic dysfunction may be masked or under appreciated. With successful transplantation there is an abrupt increase in SVR and afterload, and patients may develop decompensated congestive heart failure. A decrease in cardiac index (CI) or CO is seen in most recipients after LT acutely, but nonsurvivors typically have lower EF prior to transplant than the survivors indicating poorer cardiac reserve (13,79–81).
Pulmonary Issues and Pretransplantation Evaluation
There are several reasons for pulmonary disease to co-exist with liver failure. Some are related to the cause of liver failure itself, such as emphysema in α-1 antitrypsin deficiency and fibrosing alveolitis associated with primary biliary cirrhosis. Additionally, complications of portal hypertension, regardless of etiology of liver failure, can affect lung function. Ascites and hepatic hydrothorax cause physiologic restriction and decreased functional residual capacity. However, the pulmonary issues that receive the most attention include the vascular abnormalities of HPS and PoPH (82).
Hepatopulmonary Syndrome
The term hepatopulmonary syndrome (HPS) was first used in 1977 and was preceded by autopsy descriptions of marked pulmonary vascular vasodilation correlating with clinical findings of hypoxemia. Enhanced NO production has been implicated as the key factor for the development of pulmonary vascular vasodilation leading to a ventilation–perfusion mismatch and hypoxemia. NO-synthase (NOS) activity in the endothelium and intravascular macrophages appears to be responsible for the enhanced NO production in the lungs (83). Data from multiple liver transplant centers suggest that the incidence of HPS, including mild stages, ranges from 5% to 32% (84). Even after the adjustment for severity of liver disease, the presence of HPS infers a worse survival rate with a median survival of 24 months and 5-year mortality rate of 28% without LT; for patients with severe HPS, the mortality is much worse (Table 72.2) (84–86).
Dyspnea, resting or exertional, is the primary but nonspecific symptom in HPS. Platypnea, dyspnea that increases in the upright position and improves when supine, is a somewhat more specific complaint encountered in HPS. Physical findings of clubbing, cyanosis, hypoxemia, and spider nevi are suggestive of HPS. Severe hypoxemia (PaO2 below 60 mmHg) without another cause and orthodeoxia (a decrease in PaO2 by 5% or more with change from supine to upright position) are strongly suggestive of HPS (84).
The gold standard and most practical method in the diagnosis of HPS is contrast echocardiogram demonstrating intrapulmonary shunting. Microbubble opacification of the left atrium following three to six cardiac cycles after its appearance in the right atrium indicates passage through an abnormally dilated pulmonary vascular bed; microbubbles will not pass through normal capillaries (<8–15 μm) (84,87,88). A more invasive and less sensitive approach is a technetium-99m–labeled, microaggregated albumin lung scan with quantitative uptake in the brain (84).
TABLE 72.2 Diagnostic Criteria for Hepatopulmonary Syndrome | |
![]() |
LT is the only therapy for HPS and results in resolution in 85% of patients transplanted, but there remains an inability to predict reversibility (82,84,85,89). The duration of time after LT until improvement can be quite variable, ranging from a few days to 2 years. The overall 5-year survival rate of all stages of HPS following LT is 76%. The duration of time to improvement and postoperative mortality are both increased in those with severe pretransplantation HPS (84). Because of the high mortality without LT in those with HPS and a PaO2 below 60 mmHg, LT should be considered in these patients who are otherwise adequate candidates for transplantation. A room air PaO2 of at least 50 mmHg is the greatest predictor of posttransplant mortality; most centers choose a transplantation cut-off of a PaO2 somewhere between 40 and 50 mmHg (84,85).
Identification of HPS on pretransplant evaluation requires a multidisciplinary approach to evaluation and management. Consultation with a pulmonologist who is familiar with the syndrome is recommended. In patients with severe HPS who are not considered to be candidates for LT, referral to palliative care is a reasonable option.
Portopulmonary Hypertension
PoPH is a condition involving the pulmonary circulation in cirrhotic patients with portal hypertension (90–92). The definition of PoPH consists of three essential elements:
- Mean pulmonary arterial pressure (mPAP) greater than 25 mmHg
- Pulmonary vascular resistance (PVR) greater than 240 dynes·sec·cm–5
- Pulmonary arterial occlusion pressure (PAOP) less than or equal to 15 mmHg (93,94)
About 8% of patients on the wait list for LT have PoPH and its presence profoundly impacts survival. Patients with PoPH are candidates for a MELD exception to expedite LT (Table 72.3). A retrospective study from the Mayo Clinic composed of PoPH patients from 1994 until 2007 revealed a median survival of 15 months and a 5-year survival of 14% in those not treated with pulmonary vasodilators (95). For individuals who received pulmonary vasodilators, the median survival improved to 46 months with a 5-year survival of 45%. The perioperative mortality of LT in patients with untreated PoPH is profound. An early study by Krowka et al. (96) revealed an mPAP greater than 50 mmHg to be associated with 100% mortality, while a mPAP between 35 and 50 mmHg with a PVR above 250 dynes·sec·cm–5 had a 50% mortality rate.
TABLE 72.3 Criteria for MELD Exception |
![]() |
All potential LT recipients should be screened for PoPH, given the associated mortality; PoPH is reported present in up to 16% of patients with cirrhosis and refractory ascites, but 4% or less in those without refractory ascites (97). Mild PoPH may be asymptomatic and the symptoms associated with more severe PoPH, such as peripheral edema, ascites, and dyspnea on exertion, mimic those of ESLD and are easily overlooked or misinterpreted (94). An ECG with findings of right atrial or right ventricular (RV) enlargement or RBBB and chest radiographic findings of enlarged pulmonary arteries and cardiomegaly should raise suspicion for PoPH, but both CXR and ECG have low sensitivity for the diagnosis. TTE is an excellent screening tool, with a sensitivity of 98% and specificity of 96% when using a cutoff value for the RV systolic pressure of 40 mmHg; the diagnosis of pulmonary hypertension is confirmed by right heart catheterization (94).
In the past the presence of PoPH was considered a contraindication to LT given the profound perioperative mortality rates. Now, most centers treat these patients with pulmonary vasodilators. LT may commence if there is adequate response to treatment based on improved mPAP and RV function. This is a laborious and costly undertaking; it requires close follow-up with frequent echocardiography and/or right heart catheterization to monitor the response to treatment. The medical regimens are intense and require close attention to detail and unfailing compliance by the patient. The treatment may require an infusion pump and continuous supply of a refrigerated drug such as epoprostenol. Ideally, a pulmonologist or other expert in pulmonary vascular disorders should manage these patients. Many centers will proceed with LT if mPAP is reduced below 35 mmHg and RV function improves. Adequate RV function is likely the best predictor of operative survival, although no studies are available to support assertion (97–100). In some patients LT reverses PoPH and they are eventually weaned from the pulmonary vasodilators over a period of time. Currently, there are no means to predict who will respond and who will need continued treatment for PoPH for life (94,97).
Compliance with the pulmonary vasodilator therapy before, during, and after surgery is essential. These drugs have varying half-lives and abrupt cessation or changes in dosing can cause a precipitous decline in RV systolic function, serious systemic hypotension, and death. Close consultation with the prescribing physician may be necessary during the acute perioperative period or during any ICU stay for patients with PoPH who are on these therapies.
Hepatorenal Syndrome
HRS is a functional renal impairment that occurs in 11.4% of patients with liver failure within 5 years of the first episode of significant ascites (101). There are two types, both potentially reversible with LT. HRS 1 is rapidly progressive, with doubling of initial creatinine to above 2.5 mg/dL, oliguria, or 50% reduction of creatinine clearance to less than 20 mL/min occurring in less than 2 weeks. HRS 2 is associated with a more moderate, steady decline in renal function and ascites refractory to diuretics (102). Criteria for the diagnosis of HRS include the following (103):
- Cirrhosis with ascites
- Serum creatinine over 1.5 mg/dL
- No improvement in serum creatinine after 2 days of treatment with diuretics and volume expansion with albumin (1 g/kg to a maximum of 100 g/day)
- Absence of shock
- No current or recent exposure to nephrotoxic agents
- Absence of signs of parenchymal renal disease as suggested by proteinuria or hematuria or abnormal renal ultrasound results
Arterial vasodilation in the splanchnic circulation caused by portal hypertension plays a primary role in the pathogenesis of HRS. As liver failure progresses, despite the increases in CO and decreased SVR, there are local increases in renal vascular resistance due to the activation of the renin–angiotensin system, followed by a further decline in renal perfusion and glomerular filtration rate (GFR) and impaired sodium and water excretion. It is important to bear in mind that the true reduction in GFR may be disguised by a relatively normal serum creatinine as muscle mass in patients with long-standing liver disease and cirrhosis is usually significantly reduced (102,104).
Without the recovery of hepatic function, the prognosis for patients with HRS is very poor overall, with an approximate survival rate of 50% in 1 month even with dialysis (102,105,106). Kidney function may recover if liver failure resolves or LT is performed. The potential for renal recovery, however, is difficult to predict and is negatively impacted the longer dialysis is needed. Deciding which patients will require a combined liver/kidney transplant remains in evolution and requires a multidisciplinary approach (107).
Patients with HRS 1 are often managed in an ICU setting as they are likely to deteriorate. In most cases diuretics should be stopped, particularly potassium-sparing diuretics. Early management may include large volume paracentesis if the abdomen is tense, followed by albumin infusion of 8 g for each liter of ascitic fluid removed. Early paracentesis may improve intra-abdominal pressure and renal perfusion and allow assessment for peritonitis (79). Maintenance of euvolemia, preferably with albumin, and vasoconstrictor therapy to improve mean arterial pressure (MAP) is the mainstay of treatment (102,108). Terlipressin, a vasopressin analogue, is the treatment of choice in some countries, but is not approved in the United States or Canada (102,109–111). The efficacy of treating HRS with terlipressin versus norepinephrine appears similar, but adverse events (particularly abdominal pain, chest pain, ischemic events, and arrhythmias) are more frequently encountered with terlipressin. The cost of terlipressin is more than threefold that of norepinephrine (112,113). Norepinephrine is the vasopressor of choice in the United States for the treatment of HRS in the ICU. Oral midodrine, a selective α-1 adrenergic agonist, may be used in patients not considered critically ill. Octreotide, a somatostatin analogue, inhibits endogenous vasodilator release and increases splanchnic vasoconstriction. Octreotide, combined with a vasoconstrictor, theoretically improves renal and splanchnic hemodynamics. There is limited evidence that supports the efficacy and safety of octreotide use in combination with a vasoconstrictor for patients with HRS (114,115).
In select patients with HRS who fail to respond to medical therapy, placement of a transjugular intrahepatic portosystemic shunt (TIPS) is a possible therapeutic option (103,116). Some patients appear to benefit exhibiting improvement in creatinine clearance, reduction in serum creatinine, and increased urinary sodium excretion. Patients in the ICU may be too ill to tolerate placement of TIPS and there are complications that occur following TIPS placement, including hepatic encephalopathy, worsened hepatic function, further renal injury from the use of IV contrast agents during TIPS placement, and death. Mortality rates from HRS are still substantial following TIPS without LT or return of hepatic function (116).
Early consultation with nephrologists knowledgeable about HRS is recommended. Renal replacement therapy (RRT) is an option for appropriate patients who fail medical therapy. In the ICU continuous renal replacement therapy (CRRT) is often required due to concomitant hemodynamic instability. Patients who have the potential to recover from acute hepatic failure or those on the LT waiting list are more likely to benefit. Benefit is less clear in patients with HRS who do not have recoverable liver failure and who are not transplant candidates (102). Since recovery of liver function is the greatest hope for survival in HRS, urgent assessment for possible LT should transpire in appropriate patients. In those patients with nonrecoverable liver failure who are deemed not to be candidates for LT, realistic expectations and outcomes should be communicated to the patient and family; palliative care is a reasonable approach to treatment in this situation.
OBESITY IN LIVER TRANSPLANTATION
Obesity is a global pandemic; patients presenting for consideration of LT are no exception. This has made NASH one of the fastest growing indications for LT. Currently, it is the third most common etiology of liver disease in patients who have had LT in the United States (2,117) and the second most common etiology of liver failure on the transplant waiting list (118). It has been predicted that NASH may exceed hepatitis C and alcohol as the most common reason for LT within the next decade (117–119). NASH is the hepatic manifestation of metabolic syndrome, which is a combination of increased abdominal girth, hypertension, hyperglycemia, and hyperlipidemia. Patients with NASH have increased risk for early postoperative and delayed morbidity and mortality, particularly cardiovascular events (18,19,117). The impact of obesity itself on mortality following LT remains uncertain; early analyses and reviews suggest that obese patients have worse survival outcomes. However, the findings of more recent single-center and multicenter reviews contradict the earlier findings (119–121). Given that obese patients are more likely to have comorbid conditions such as metabolic syndrome, obstructive sleep apnea, diabetes mellitus, and coronary artery disease, and are often less functional than nonobese candidates, it is not surprising that they appear to be at increased risk for postoperative morbidity and increased resource utilization. These are evidenced by longer ICU and hospital stays, operative times, transfusion needs, wound complications, infectious complications, cardiovascular events, and biliary complications requiring interventions (19,120,121). Hence, LT in the morbidly obese is not a trivial undertaking. But, given that patient and graft survival appear to be similar to that in nonobese patients, obesity itself should not be considered an absolute contraindication (117,121). Preoperative LT assessment of obese patients should include a thorough evaluation for cardiac, pulmonary, endocrine, and nutritional disturbances commonly associated with obesity. Sustained weight loss through diet and exercise is the most effective strategy for NASH and a multidisciplinary approach to the pretransplantation and posttransplantation weight management should be utilized (117).
COAGULOPATHY OF LIVER FAILURE AND TRANSFUSION
Patients with cirrhosis have a true bleeding–clotting diathesis. Several pathophysiologic abnormalities, other than low levels of procoagulants, may promote bleeding complications in ESLD. The vascular phase of hemostasis is impaired by vasodilation and a reduced vascular constrictive response to injury. Hemodynamic alterations of portal hypertension cause vascular congestion, especially of the mesenteric vessels. This, combined with the fragility of esophageal varices, explains the proclivity of these patients to present with gastrointestinal bleeding rather than a coagulopathy. Other factors may promote bleeding, including thrombocytopenia, which is secondary to hypersplenism, bone marrow suppression, and decreased thrombopoietin production in ESLD. Fibrinolysis may be poorly regulated due to elevated levels of tissue plasminogen activator (tPA), as well as poor hepatic clearance and increased extravascular production of tPA (122–124).
Historically, it was suggested that patients with liver failure are “auto-anticoagulated.” This old dogma was based on abnormal findings of traditional tests of coagulation, such as PT, international normalized ratio (INR), platelet count, and partial thromboplastin time (PTT) suggesting risk for bleeding and poor coagulation. The emerging model of coagulation in patients with ESLD is one of “rebalanced hemostasis” (Fig. 72.5). This theory takes into account that procoagulant and anticoagulant factors are decreased in parallel, since most are manufactured by the liver (122–124). In ESLD, the INR does not accurately predict the need for transfusion or the risk of bleeding during invasive procedures (125–130). Furthermore, there is a lack of supportive evidence that transfusion with FFP for the purpose of normalizing the INR prior to an invasive procedure reduces transfusion needs and bleeding risk. In fact, it may actually increase the risk of bleeding by increasing central venous pressure (CVP) and vascular congestion (127–129,131,132,133). Blood from patients with ESLD generates as much thrombin as normal controls when methods of dynamic testing, such as thromboelastography (TEG), are used; this represents the activity of both procoagulants and anticoagulants (122,125,134–138). TEG is an accepted alternative to traditional tests of coagulation to assess coagulation and guide transfusion in ESLD, as most serum component markers of coagulation are reflected in the intricate dynamics of whole blood clotting.
Even more interesting, patients with cirrhosis have a nearly twofold increased risk for spontaneous venous thromboembolism (VTE) compared to age-matched population controls (139). There are several mechanisms that tip the hemostatic balance in favor of coagulation and thrombin generation (122,123,134–139). Important contributors include elevated levels of factor VIII and Von Willebrand factor in ESLD. Activated protein C downregulates thrombin formation, and thrombomodulin is the primary activator of protein C; levels of both are low in ESLD. ADAMTS 13, which normally limits the function of VWF on platelets, is present at lower levels than normal in ESLD. This elevated level of VWF and the greater affinity of VWF for platelets may explain why platelet adhesion to injured vascular endothelium is maintained, despite lower platelet counts. Factor VIII plays a key role in thrombin generation, and circulating levels actually increase as the severity of liver disease progresses (122–124,135). Given the tendency toward increased VTE risk, consideration should be given to the use of antithrombotic prophylaxis for patients with ESLD in the ICU.
FULMINANT HEPATIC FAILURE
ALF may be defined as the abrupt loss of liver function, characterized by hepatic encephalopathy and coagulopathy within 26 weeks of the onset of symptoms—classically jaundice—in a patient without previous liver disease. Although ALF frequently results in death, many will recover with supportive medical therapy. In the past 20 years, improved critical care management has substantially improved survival in ALF without LT (140). When LT for ALF transpires, the burden of neurologic and infectious complications of ALF extend into the posttransplant period, resulting in inferior survival rates compared to those of elective LT. This combination of improved survival without LT and suboptimal survival after LT makes accurate identification of those patients who will actually benefit from LT complex (141–143).
The prognosis of fulminant liver failure is determined by four key elements (144):
- Etiology
- Rate of progression
- Age of the patient
- Laboratory markers of disease severity
Since the pace of the evolution of ALF has important implications on mortality, it may be subdivided based on the jaundice-to-encephalopathy interval. With hyperacute liver failure the interval is 7 days or less; ALF has an interval of 8 to 28 days; and subacute liver failure more than 28 days. In general, patients with hyperacute liver failure have a more favorable rate of spontaneous survival without LT. This group is more likely to have acetaminophen (APAP) overdose or acute hepatitis A as the etiology of ALF, but are also more likely to have cerebral edema; in contrast, subacute liver failure patients have a worse rate of spontaneous survival. APAP overdose constitutes nearly 50% of the cases of ALF in the United States (Fig. 72.6) (140).
Infection occurs in 80% of patients with fulminant hepatic failure. The use of prophylactic antibiotics may increase the risk of fungal infections, often fatal, which occur in roughly one-third of patients with ALF. Encephalopathy inversely correlates with prognosis; Table 72.4 summarizes the four stages of encephalopathy that are seen in FHF. Cerebral edema occurs in most cases that progress to stage 4. Typical symptoms of severe cerebral edema are the Cushing reflex, decerebrate rigidity, disconjugate eye movements, and a loss of pupillary reflexes (145).
The King’s College Hospital criteria, described in 1989, were the first to differentiate between APAP-induced ALF and other causes; Table 72.5 summarizes the King’s College criteria for LT (146). The sensitivity and specificity of the King’s College criteria for LT in ALF have been evaluated in several meta-analysis and systematic reviews, with reported overall specificity of 82% for non-APAP etiologies and 92% to 95% for APAP-induced ALF (144,146–149). When considering whether or not to proceed with LT, reduced sensitivity leads to failure to transplant a patient with ALF who will die without LT, but reduced specificity carries the risk of unnecessary transplantation in a patient likely to recover spontaneously. In this setting, the MELD score has a high sensitivity and negative predictive value, but a low specificity. Use of the MELD score in conjunction with King’s College criteria may be beneficial to the decision-making process (144,150). One of the main components of both scores is the INR, hence unnecessary correction of the INR may affect prognostication. The use of liver biopsy for prognostication in patients with ALF is controversial. Assessing the volume of viable hepatocytes may have prognostic value, but sampling error is a point of contention. A critical mass of at least 25% to 40% viable hepatocytes is considered to have a good prognosis. Hepatomegaly may warrant biopsy to rule out a malignant infiltrative process or consideration of alcoholic hepatitis as the etiology of ALF (151).

FIGURE 72.5 Schematic presentation of the balance between procoagulant and anticoagulant factors in patients with liver disease. Both sides of the balance are functionally reduced, resulting in a more or less rebalancing of the hemostatic system in these patients, although at a lower level with smaller margins. FVIII, factor VIII; YTAFI, thrombin activatable fibrinolysis inhibitor; tPA, tissue plasminogen activator; VWF, von Willebrand factor. (Adapted from Warnaar N, Lisman T, Porte R. The two tales of coagulation in liver transplantation. Curr Opin Organ Transplant. 2008;13(3):298–303.)

FIGURE 72.6 Causes of acute liver failure in the United States: data from the Acute Liver Failure Study Group Registry, from 1998 to 2007. Percentages of the total number of patients enrolled are shown above each etiology. (Data courtesy of W.M. Lee, Principal Investigator, the Acute Liver Failure Study Group.)
Intracranial hypertension (ICH) and cerebral edema are a frequent cause of death, second only to infection, both before and after LT in ALF. In patients with severe or progressive encephalopathy, empiric strategies to prevent or reduce ICH include mild respiratory alkalosis (PaCO2 of 30–35 mmHg), elevating head of bed to at least 30 degrees, mild hypernatremia (Na+ 145–155 mEq/L), and avoiding noxious stimulation. Vasopressors will often be required to treat hypotension and maintain cerebral perfusion pressure. Osmotically active agents (e.g., mannitol) have been successfully used to decrease cerebral edema. Other therapeutic maneuvers employed for severe encephalopathy and ICH have included barbiturate coma and induced hypothermia (140). The utilization of intracranial pressure (ICP) monitors to manage ICH in ALF is controversial. Institutional practices vary widely from standard practice in some to never in others. Major points of contention include significant risk of associated intracranial bleeding and lack of evidence supporting improved outcomes. The rate of fatal intracranial bleeding associated with ICP placement in this situation is approximately 3.5% (140). Despite a lack of evidence, many experts believe ICP monitors improve management and outcomes, but may also provide information as to when the process may not be recoverable. The US Acute Liver Failure Study Group has supported the use of ICP for patients with ALF, particularly in those with severe encephalopathy who will go on to LT or who have a high likelihood of spontaneous recovery (140,152).
TABLE 72.4 Hepatic Encephalopathy in Fulminant Hepatic Failure | ||
![]() |
TABLE 72.5 King’s College Criteria for Liver Transplantation in Fulminant Hepatic Failure |
![]() |
Due to improved ICU management, the past two decades have witnessed improved outcomes of ALF with and without transplantation. There is now some doubt about the benefit of LT in patients with APAP-induced ALF, the prompt recognition of which is critical, since a specific antidote, N-acetylcysteine (NAC), effectively limits hepatocellular injury by replenishing glutathione, the putative scavenger of the reactive APAP metabolite (N-acetyl-p-benzoquinoneimine), and preventing its binding to hepatocellular proteins. King’s College Hospital treated 3,300 patients with ALF between 1973 and 2008, with overall survival rate increasing from 16.7% to 62.2%. This improvement followed the introduction of NAC and LT as treatment for APAP-induced ALF; more notably, survival without transplantation improved to 48% (144). A prospective study of 275 APAP-related ALF carried out by the US Acute Liver Failure Group included 72 patients who were placed on the waiting list for transplantation. The overall survival rate was 73%, increasing to only 78% after LT; more remarkable, over half of the patients placed on the wait list recovered without being transplanted. There was no statistically significant difference in survival after listing for transplantation based on whether or not a transplant took place (149). In both European (ELTR) and US (UNOS) transplant databases, neurologic complications account for 13% of posttransplant mortality in ALF. Both databases attribute death to infection in 18% to 24% of cases of which approximately 25% are fungal infections (141–143).
There are few more difficult decisions in critical care than the determination as to whether a patient with ALF should be listed for LT. In ALF the clinical condition can change significantly within short time intervals. The implication is that their clinical status may markedly improve or deteriorate from the time of their placement on the wait list to organ allocation, making LT irrelevant, either because they are now recovering with supportive care or too ill to proceed with LT. Defining the point when the anticipated outcome no longer justifies the utilization of the organ is difficult from an evidence-based standpoint. An outline of some indicators of when to proceed to transplantation, pause to consider, or abandon a plan to transplant is presented in Table 72.6 (144). A multidisciplinary decision-making process should include a thorough psychosocial evaluation, and consultations by hepatology, transplant surgery, the intensivist and the patient or their proxy.
SURGICAL AND ANESTHETIC MANAGEMENT
LT is one of the most complex surgical procedures, requiring intensive resources and multidisciplinary care. However, in the past two decades improved outcomes have reached levels that would have been inconceivable previously. This success has been achieved through enhancements of care by all involved in LT including:
- Enhanced donor management
- Improved surgical techniques
- Advances in anesthetic management
- Superior immunosuppressive medications
TABLE 72.6 Kings College Criteria Guidelines for Urgent Liver Transplantation in Acute Liver Failure |
![]() |
Surgical Management
As described by Starzl, the original surgical procedures were prolonged and required complete cross-clamping of the portal vein (PV) and the inferior vena cava (IVC) above and below the liver. Venovenous bypass (VVB) was introduced to combat the hemodynamic challenges and mesenteric congestion created by the interruption of the IVC and PV. However, VVB carries complications of its own including vascular and nerve injury, fatal air- or thromboembolism, hemothorax, lymphedema, prolongation of ischemic times of the graft, hypothermia, and infections. In the late 1980s, the piggyback technique, a caval-sparing technique, with end-to-side or side-to-side caval anastomosis was introduced and is generally the procedure of choice for most primary liver transplants today (Fig. 72.7). It spares the IVC and results in improved outflow of the allograft. This technique has resulted in shortened operative and ischemic times and diminished transfusion needs (153,154). A few centers still use VVB routinely, but many feel the shortened anhepatic time of the piggyback technique and partial, rather than complete, clamping of the IVC minimizes mesenteric congestion. VVB may be used occasionally for select, high-risk cases, such as redotransplantations or transplantation for ALF.
Anesthetic Management
Dramatic improvements in anesthetic management have positively impacted outcomes in LT at least equal to that of surgical advancements. These changes include improvements in clinical care as well as evidenced-based administrative practices.
Massive intraoperative blood loss and transfusion requirements were nearly insurmountable prior to the last two decades. Now, bloodless LT is a reality and many transplants transpire with few or no blood products transfused. This is of paramount significance in improving LT outcomes as transfusion is linked increased complication rates, morbidity, and mortality (155,156). Several anesthetic practices have been credited in the reduction of transfusion needs. Lower CVP and fluid restriction during hepatic dissection reduces hepatic and vascular congestion and, consequently, bleeding (157). In addition, avoiding excess intravenous fluid also prevents dilutional coagulopathy and hypothermia, and results in a less edematous allograft with improved function. Using vasopressors to maintain adequate MAP rather than fluids is now standard management in LT. Normovolemic hemodilution, another method utilized to decrease both bleeding and allogeneic transfusion needs in patients with adequate hematocrit, is used successfully in donor hepatectomies for live donor LT to prevent unnecessary transfusions in this otherwise healthy population (158). Anesthesiologists are vigilant in preventing and treating hypothermia and correcting hypocalcemia to further minimize intraoperative bleeding (159,160).

FIGURE 72.7 Piggyback technique and creation of a common confluence for hepatic venous outflow. (From Eghtesad B, Kadry Z, Fung J. Technical considerations in liver transplantation: what a hepatologist needs to know [and every surgeon should practice]. Liver Transpl. 2005;11(8):861–871.)
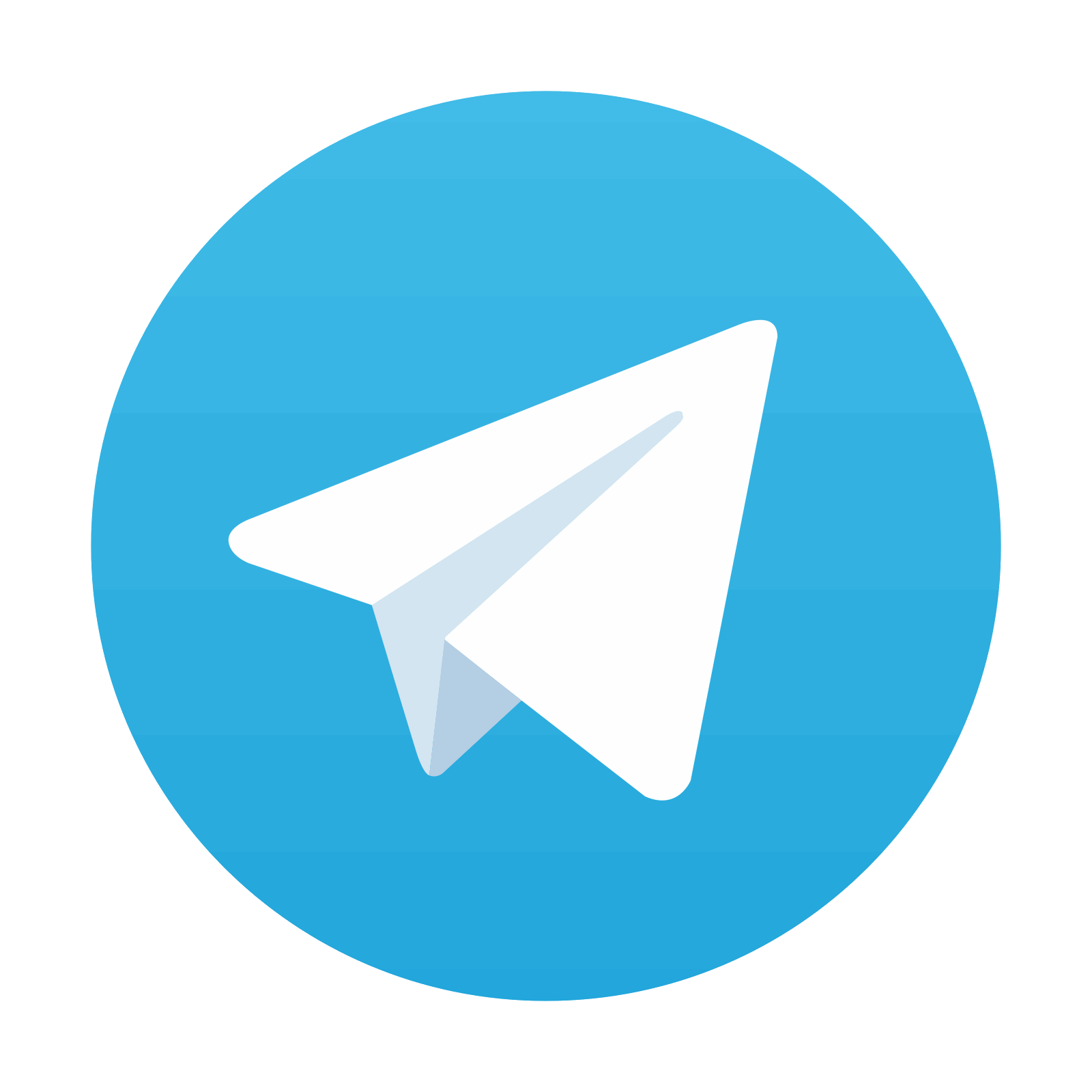
Stay updated, free articles. Join our Telegram channel

Full access? Get Clinical Tree
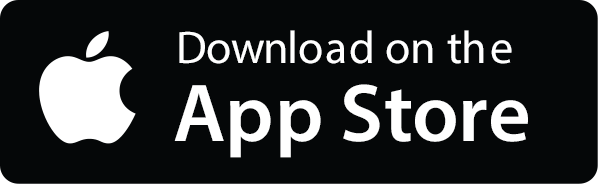
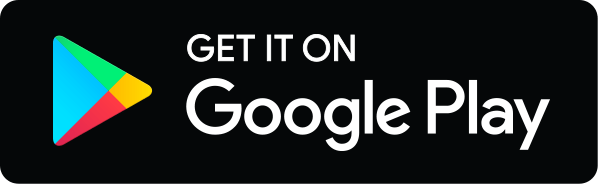