Key Points
- ▪
The liver receives a dual afferent blood supply; the portal vein provides 75% and the hepatic artery 25% of hepatic inflow. With differences in oxygen content, each source contributes about 50% of the liver’s oxygen supply.
- ▪
Hepatocytes make up 80% of the liver. Nonparenchymal cells include Kupffer cells, members of the monocyte-phagocyte system that play a key role in immunity. Stellate cells undergo transformation to fibroblasts and produce collagen in response to injury, fundamental to fibrosis and cirrhosis.
- ▪
Hepatic dysfunction affects most organ systems because of the liver’s extensive metabolic, detoxification, and digestive functions; alterations cause encephalopathy, coagulopathy, and muscle wasting.
- ▪
Liver injury leads to fibrosis and portal hypertension, which is responsible for ascites, gastrointestinal bleeding, and renal dysfunction, the characteristic manifestations of cirrhosis.
- ▪
The major causes of liver disease include noncholestatic cirrhosis, cholestatic cirrhosis, acute hepatic necrosis, biliary atresia, metabolic diseases, malignant neoplasms, and drug-induced injury. Noncholestatic cirrhosis includes hepatitis; hepatitis C is the most common diagnosis in U.S. liver transplant recipients.
- ▪
Nonalcoholic steatohepatitis is increasing in incidence in the United States, possibly secondary to the obesity epidemic.
- ▪
Alagille’s syndrome is the most common form of familial intrahepatic cholestasis; 90% of patients have associated congenital heart disease, most often pulmonic stenosis.
- ▪
Alpha-1-antitrypsin deficiency, the most common metabolic disorder of the liver, is associated with decreasing FEV 1 and exacerbated by smoking.
- ▪
Hemochromatosis first affects the liver, then the pancreas with diabetes mellitus, the skin with bronze pigmentation, and iron deposition in the heart with restrictive cardiomyopathy and arrhythmias.
- ▪
Mucopolysaccharidoses affect enzymes in glycosaminoglycan metabolism. Each presents differently, but all may be associated with difficult intubation from immobility and macroglossia.
- ▪
Postoperative jaundice can result from increased production of bilirubin, hepatocellular injury, and cholestasis. Causes include hemolysis of transfused red blood cells, reabsorption of hematomas, liver hypoperfusion, and drug-induced injury.
- ▪
Further key points are noted throughout the chapter.
The liver is a complex organ system with myriad functions that is susceptible to dysfunction as a result of disease processes and physiologic abnormalities. A dysfunctional liver can affect the function of almost every organ system in the body. Anesthesia and surgery can stress the reserve of the liver, resulting in worsening function even without overt evidence of cause. These factors can make the preoperative, intraoperative, and postoperative care of patients with liver disease challenging. An understanding of normal hepatic structure and function, the acute and chronic responses of the liver to various types of injury, and the behavior of the healthy or diseased liver during perioperative events helps structure an approach to anesthetic management issues. Representative disease processes are examined for unique aspects of perioperative evaluation and care for patients with specific liver diseases.
Normal hepatic anatomy
Certain aspects of hepatic anatomy and physiology with implications for perioperative care of the patient with liver disease include (1) the dual blood supply of systemic blood via the hepatic artery and portal venous blood from the splanchnic circulation; (2) histologic arrangement of hepatocytes, including the unique hepatic sinusoids and the resulting blood-hepatocyte interface; and (3) isolation of biliary and blood compartments with regulation of enterohepatic circulation.
The liver is the largest parenchymal organ in the human body, representing approximately 2% of total body weight in the adult. Blood flow to the liver is normally 100 mL/100 g of tissue per minute, or 25% to 30% of the resting cardiac output. The metabolic functions of the liver are facilitated by the interposition of the liver between the splanchnic and systemic venous systems. Approximately 75% of the blood supply to the liver is delivered by the portal vein. This blood is partially deoxygenated as a result of oxygen extraction by the splanchnic organs. After coursing through capillary beds of the stomach, pancreas, spleen, and intestines, portal venous blood contains high concentrations of nutrients, as well as secreted and ingested exogenous substances. Under normal circumstances, this portal blood provides 35% to 50% of the oxygen delivered to the liver. The well-oxygenated blood of the hepatic artery delivers the remaining 50% to 65% of oxygen, despite being only 25% of the liver’s blood supply. Portal venous flow depends on the normal variations in splanchnic blood flow, as regulated by the arterioles and capillary flow of the splanchnic bed. Hepatic artery blood flow demonstrates autoregulatory changes in response to blood pressure, as well as to portal blood flow and sinusoidal oxygen levels.
The liver comprises two lobes of unequal size, the right and left lobes, as divided by the falciform ligament. These lobes can be further subdivided into the eight segments of Couinaud ( Fig. 5-1 ), based on separate vascular and biliary branches. Vascular outflow through the hepatic veins crosses between segments. Segments may be surgically resected to excise pathologic lesions or living-directed donation for transplantation.

Microscopically, the classic concept of the hepatic histologic structure is that of the hepatic lobule ( Figs. 5-2 and 5-3 ). This model is that of a polygon, typically a hexagon, with branches of the portal triad (hepatic artery, portal vein, and bile duct) at the vertices. A central vein, technically a venule, marks the central axis of the lobule. Mixed arterial and portal blood flows from the vessels at each vertex, through the sinusoids, to the common central vein. The sinusoids are formed by one-cell-thick plates of hepatocytes and lined with endothelial cells. These sinusoids differ from normal capillaries because of the mixture of portal venous and arterial blood. They also lack a basement membrane, and their endothelium has fenestrations typically ranging in size from 50 to 200 nm. These fenestrations and the low sinusoidal pressure allow a multitude of solutes, including macromolecules, to enter the perisinusoidal space of Disse. Here, molecules are in direct contact with the microvilli of the hepatocyte’s basolateral membrane. The hepatocyte also has specialized canalicular membrane portions with distinct microvilli. In combination with the adjacent hepatocyte, this specialized area forms the wall of the bile canaliculi, its isolation completed by tight intercellular junctions. Intracellular actin and myosin filaments along the canalicular channel are presumed to promote drainage of bile into the canals of Hering and subsequently into the interlobar bile ducts.


Concentric zones radiating from the portal triad out to the draining venule, numbered 1 to 3, reflect decreasing oxygen content in the sinusoidal blood and decreasing concentrations of nutrients arriving from the gut. These zones correlate with differential enzyme concentrations, metabolic activities, and degree of cellular damage caused by a variety of agents and situations ( Fig. 5-4 ).

Although hepatocytes make up about 80% of the liver, a host of other cells are found in the liver. Two important nonparenchymal cells are Kupffer and stellate cells. Kupffer cells are members of the monocyte-phagocyte system (macrophage derived) and typically reside on the luminal aspect of sinusoidal endothelial cells. Their phagocytic and inflammatory responses are important in sepsis, eliminating bacteria and clearing inflammatory mediators. Thus, patients with liver disease tend to be prone to infectious complications. Stellate cells (Ito cells) are found in the space of Disse and, in health, store lipids and vitamin A. In the fibrogenic response to injury, however, the stellate cells undergo transformation to fibroblasts and produce collagen, which leads to loss of fenestrations and creation of a pseudo–basement membrane. This is believed to be a fundamental step in the development of hepatic fibrosis and cirrhosis.
Key Points
- ▪
The liver is the largest parenchymal organ.
- ▪
Blood flow is normally 25% to 30% of cardiac output.
- ▪
Portal vein is responsible for 75% of blood flow and 35% to 50% of oxygen delivery.
- ▪
Hepatic artery is responsible for 25% of blood flow and 50% to 65% of O 2 delivery.
- ▪
Hepatocytes are arranged in zones leading away from portal triad; each zone shows varying degrees of O 2 content, enzyme concentrations, metabolic activity, and cellular damage.
- ▪
Through the liver, carbohydrate and lipid metabolism provides an energy source for the body.
- ▪
The liver produces proteins in plasma (except for immunoglobulins), detoxifies and transforms endogenous and exogenous substances, metabolizes heme, and provides immune function of Kupffer cells.
Hepatic function in health
Carbohydrate Metabolism
The liver provides for the body’s varying energy requirements under the modulation of neural and endocrine regulators. Complex interacting systems of energy storage and utilization are required to compensate for asynchronous periods of nutritional ingestion and energy demand. Figure 5-5 presents a simplified diagram of carbohydrate and lipid metabolism in the hepatocyte.

Many cells of the body are glucose dependent (e.g., erythrocytes, renal medullary and retinal cells) or glucose preferential (e.g., brain cells). Maintenance of blood glucose levels depends on nutritional circumstances. Glucose is eventually released from stored glycogen through glycogenolysis, as promoted by epinephrine and glucagon. The liver and the skeletal muscle contain the vast majority of the body’s glycogen. Glycogen stores in the adult liver during fasting are capable of providing adequate glucose levels for 24 to 48 hours, representing 250 to 500 mg of glucose. During fasting the brain will transition from glucose dependency to ketone metabolism and thus sustain itself while greatly decreasing the body’s daily glucose utilization. Gluconeogenesis is the creation of glucose from lactate, pyruvate, and amino acids, the products of anaerobic and catabolic metabolism, as stimulated by the depletion of glycogen stores.
The liver can rapidly switch from glycogen breakdown to glycogen formation, depending on the current nutritional state and energy requirements. Disruption of carbohydrate homeostasis can be a manifestation of liver dysfunction. Acute liver injury (e.g., viral hepatitis) is often associated with mild hypoglycemia despite normal or depressed insulin levels. Hypoglycemia can be pronounced in the alcoholic patient despite apparently minimal hepatic decompensation; ethanol cannot be used in gluconeogenesis, and its metabolism can critically reduce the availability of pyruvate. In fulminant hepatic failure of any cause, hypoglycemia can be life threatening.
Glucose intolerance, conversely, is often observed in chronic liver disease. Although insulin levels may actually be elevated because of decreased hepatic clearance, peripheral receptors are decreased in number. Additionally, receptor-binding characteristics and activity may be altered. Hepatocytes may also be isolated from the usual concentrated levels of portal pancreatic insulin release because of portosystemic shunting.
Lipid Metabolism and Transport
Fatty acids provide the most efficient energy source for both intrahepatic and extrahepatic storage and utilization. The liver’s central role in lipid metabolism, beyond utilization, involves regulated conversion of excess carbohydrates to fatty acids, esterification of free fatty acids to form triglycerides for transport and storage, and synthesis of transport proteins. In normal circumstances, the liver takes up a relatively fixed amount of free (nonesterified) fatty acids regardless of dietary intake. This provides the major energy source for hepatocytes. The nutritional state determines the subsequent balance between synthesis and esterification of fatty acids in the fed state versus oxidation in the fasting state ( Fig. 5-6 ).

Hepatic steatosis (“fatty liver”) refers to abnormal accumulation of predominantly triglycerides with fatty acids in hepatocytes. Previously defined in terms of weight percentage (> 5%) or number of hepatocytes affected (> 30% in a lobule), the diagnosis is now also grossly correlated to findings of noninvasive imaging. Conditions associated with steatosis include obesity, alcohol ingestion, pregnancy, nonalcoholic steatohepatitis, and certain drug toxicities, as discussed later.
Cholesterol is not a direct energy source but serves as a structural unit of membranes and is a precursor for steroid production. Most cholesterol is synthesized in the liver and, in combination with dietary cholesterol, is either secreted in the bile, incorporated into lipoproteins for plasma transport, or converted to bile acids.
Protein Synthesis
With the exception of immunoglobulins, the liver produces the vast majority of proteins found in plasma, including proteins of coagulation, plasma-binding proteins involved in transport (e.g., albumin, transferrin, lipoprotein, haptoglobin), and acute-phase reactants. These proteins share many common synthetic pathways but have distinguishing characteristics of substrate, modulation, and kinetics that explain the clinically variable response to injury and disease. For example, the clotting factors dependent on vitamin K for posttranslational modification can be affected by K’s nutritional intake or absorption, whereas inflammatory mediators stimulate acute-phase reactants. Serum albumin levels reflect not only production from available amino acids but also volume of distribution, abnormal losses (e.g., ascites, pleural effusion, proteinuria), and regulators responding to parameters such as serum oncotic pressure. Another protein monitored in patients with liver disease is alpha fetoprotein (AFP). Its role is primarily in neonatal life, with most of it being replaced by the first year. Hepatocellular proliferation in adulthood will result in an increase in AFP levels. High levels are seen in acute hepatitis and liver failure and are a marker for hepatocellular carcinoma. Altered protein levels, which actually reflect liver disease, will develop after variable periods, depending on the synthetic rates and plasma half-life of the particular proteins.
Thus, although it is generally true that serum protein levels will be decreased with liver dysfunction, the specific laboratory abnormality and time frame (hours or days for coagulation factors vs. weeks for albumin) are important diagnostic clues in liver disease.
Detoxification and Transformation
The liver is the major site in which both xenobiotics (foreign chemicals) and endogenous substances undergo detoxification and transformation. These changes usually generate less active and more hydrophilic compounds. In notable exceptions, transformation actually renders substances toxic, as discussed later. The pathways involved are categorized into three phases. Phase 1 metabolism alters the molecule by reactions, usually involving the cytochrome P450 enzyme system (CYP450), such as oxidation, reduction, and hydrolysis. Phase 2 metabolism conjugates the parent molecule or its metabolite with a polar molecule (e.g., acetate, amino acid, sulfate, glutathione) and thus further enhances water solubility. Phase 3 elimination is an energy-dependent excretion. A particular molecule may undergo any or all of these processes. Changes in the pathway(s) utilized may occur as dictated by substrate concentrations, enzyme induction, disease, and nutritional status.
Bilirubin Metabolism
Bilirubin is a tetrapyrrole produced from the breakdown of heme at the rate of about 250 mg/day in the normal adult. About two-thirds comes from hemoglobin of senescent erythrocytes processed by the reticuloendothelial system, and the remainder mostly from nonhemoglobin hemoproteins (e.g., CYP450 enzymes). The turnover of myoglobin is slow enough that its substantial hemoprotein content does not contribute significantly to bilirubin production. Heme is first converted to biliverdin by heme oxygenase and then to bilirubin by biliverdin reductase. This unconjugated bilirubin, which is water insoluble and neurotoxic at high levels, is bound to albumin and transported to the hepatocyte, where it is conjugated with glucuronic acid to form bilirubin monoglucuronide and diglucuronide. After secretion into canaliculi, bilirubin is incorporated into bile and remains unchanged through the gallbladder and most of the small intestine. In the terminal ileum and colon, hydrolysis by bacterial enzymes produces urobilinogen, which is reabsorbed, and re-excreted predominantly in bile, with a small fraction filtered by the kidney into the urine.
The injured liver
Cellular Responses
The liver can sustain injury from a variety of processes, both primary to the organ (e.g., viral hepatitis) and secondary (e.g., right-sided heart failure or metastatic cancer). Regardless of the cause, general categories of cellular consequences are typically observed.
Hepatitis is simply liver injury associated with the incursion of inflammatory cells. Depending on the type of hepatitis, hepatocyte injury may stimulate the inflammatory response (e.g., toxic injury) or may be secondary to it. Degeneration is defined in terms of microscopic findings. Foamy degeneration occurs with ineffective biliary excretion, whereas ballooning degeneration is found in toxic and immunologically mediated injury. Steatosis specifically represents accumulation of fat droplets in the cell. Multiple small accumulations are seen in microvesicular steatosis (e.g., acute fatty liver of pregnancy), whereas macrovesicular steatosis is defined as a large nucleus-displacing droplet (e.g., obese and diabetic patients).
Necrosis can occur after a variety of injuries. Necrosis results in poorly stained cells with lysed nuclei, frequently exhibiting zonal distributions. Centrilobular necrosis is a common pattern in which the most severe damage immediately surrounds the central vein. This is characteristic of toxins and ischemic injury, the latter presumably reflecting the decreasing oxygen content of the sinusoidal blood as it flows to the terminal venule, whereas the toxic pattern may reflect not only relative hypoxia but also regions of high metabolic activity and biotransformation. Periportal necrosis, conversely, is exceedingly unusual but may be found in pre-eclamptic patients for unknown reasons. With most injuries, a variety of necrotic and inflammatory patterns are seen. Focal necrosis denotes scattered necrosis within lobules, whereas more severe bridging necrosis spans adjacent lobules. Apoptosis is the energy-dependent deconstruction of cells with an attenuated inflammatory response and salvage of cell components that can be reused. The conditions and regulators that influence apoptosis in the liver are being elucidated. Whether the balance between necrosis and apoptosis can be predicted or even manipulated clinically remains to be seen.
Regeneration and fibrosis represent two different outcomes in the liver’s attempt to replace injured liver units. The liver, since the Greek myth of Prometheus, has a reputation for its unparalleled ability to regenerate. When its connective tissue framework is left intact, the liver can actually re-form itself from less than half of its original size, as demonstrated in living-directed liver donors. Similarly, the liver that has undergone extensive necrosis may subsequently recover essentially normal structure, except for minor abnormalities of bile ductules and parenchymal arrangement. Stimulating factors thus far identified in the human include epidermal, transforming, and hepatocyte growth factors.
Fibrosis is a much different consequence of injury response. It is generally irreversible and will compromise function. Fibrosis results from the deposition of collagen within the space of Disse, around portal tracts, or around the central vein by transformed stellate cells. Previously healthy hepatocytes are eventually replaced with connective tissue. Cirrhosis is the term applied to nodules of regenerating hepatocytes within such scar tissue, reflecting the impact of disruption of the normal connective framework before or during regeneration. This architectural disruption results in increased resistance to hepatic blood flow, with eventual portal hypertension and decreased functional mass with impaired metabolic and excretory function. Box 5-1 outlines causes of hepatic fibrosis and cirrhosis, which represent the consequences of a wide range of diseases. In the Western world, about 90% of cirrhosis of known etiology is related to alcoholic liver disease, viral hepatitis, or biliary disease. Approximately 10% of cases are of unknown etiology and are termed cryptogenic cirrhosis.
Medications and Toxins
Alcohol
α-Methyldopa
Amiodarone
Arsenic
Carbon tetrachloride
Chlordecone
Isoniazid
Methotrexate
Methylene diamine
Nitrofurantoin
Oral contraceptives
Pyrrolizidine alkaloids
Sulfa antibiotics
Vitamin A
Infections
Brucellosis
Capillariasis
Chronic hepatitis (B, C, and D)
Cytomegalovirus
Echinococcosis
Schistosomiasis
Syphilis (tertiary and congenital)
Metabolic and Genetic Disorders
α 1 -Antitrypsin deficiency
Alagille’s syndrome
Biliary atresia
Fanconi’s syndrome
Fructose intolerance
Galactosemia
Gaucher’s disease
Glycogen storage disease
Hemochromatosis
Hereditary tyrosinemia
Ornithine transcarbamylase
Porphyrias
Tyrosinosis
Wilson’s disease
Wolman’s disease
Other Causes
Autoimmune chronic hepatitis
Biliary obstruction (chronic)
Budd-Chiari syndrome
Cystic fibrosis
Idiopathic portal hypertension
Jejunoileal bypass
Nonalcoholic steatohepatitis
Primary biliary cirrhosis
Primary sclerosing cholangitis
Right-sided heart failure and tricuspid regurgitation (chronic)
Sarcoidosis
Laboratory Manifestations of Hepatobiliary Dysfunction
Most common tests have limitations of sensitivity and specificity and assess narrow aspects of hepatic function. The concept of a panel of tests that represent a measure of hepatic reserve or “liver function tests” is flawed. However, different patterns of abnormalities do often correlate with the underlying pathology and allow further targeted investigation. Table 5-1 summarizes common tests used to evaluate liver disease. These tests can be broadly divided into two categories, those that reflect liver injury and those that reflect liver function. The markers of direct injury include released hepatic enzymes. Synthetic function can be reflected in protein levels and clotting times, whereas dye clearance and drug transformation can be used to investigate blood flow and metabolic capacity.
Etiologies and Lab Results | Hepatocellular Necrosis | Biliary Obstruction | Chronic Infiltration | |||
---|---|---|---|---|---|---|
Toxin or Ischemia | Viral | Alcohol | Complete * | Partial | ||
Transaminases (aminotransferases) | 50-100 × | 5-50 × | 2-5 × | NL to 5 × | NL to 5 × | 1-3 × |
Alkaline phosphatase (ALP) | 1-3 × | 1-3 × | 1-10 × | 2-20 × | 2-20 × | NL to 20 × |
Bilirubin | 1-5 × | 1-30 × | 1-30 × | 1-30 × | 1-5 × | NL to 5 × |
Prothrombin time (PT) | Prolonged; minimal or no improvement with vitamin K | Often prolonged; may improve with parenteral vitamin K | Normal | |||
Albumin | Decreased in chronic disease | Often normal; may be decreased | Usually normal | |||
Illustrative disorders | Shock liver, acetaminophen toxicity | Hepatitis A or B | Pancreatic cancer | Hilar tumor, sclerosing cholangitis | Sarcoid, metastatic carcinoma |
* Acute onset of complete biliary obstruction may result in massive elevations in transaminases that are transient and in the range of 20 to 50 times normal.
Tests that reflect hepatic clearance
Ammonia
The liver normally clears ammonia from the blood and converts it to urea for renal excretion. With severe liver dysfunction or portosystemic shunting, ammonia levels may be elevated. Although typically used in the evaluation of possible hepatic encephalopathy, ammonia levels correlate poorly with the severity of clinical presentation.
Bilirubin
As discussed with normal metabolism, bilirubin is a product of heme breakdown. It exists in conjugated (water soluble) and unconjugated (lipid soluble) forms, which are reported imprecisely as the direct and indirect fractions, respectively. Serum bilirubin is usually less than 1 mg/dL and primarily unconjugated. Elevated serum levels occur in most significant liver diseases; degree of elevation correlates with prognosis in primary biliary cirrhosis, alcoholic hepatitis, and fulminant liver failure. The appearance of conjugated bilirubin in the blood is thought to be caused by reflux from the hepatocyte, but this does not discriminate between obstructive and parenchymal causes. Other causes of elevated bilirubin include Gilbert’s syndrome, increased production (e.g., hemolysis, ineffective erythropoiesis, hematoma resorption), and inherited disorders of bilirubin transport.
Tests that reflect synthetic function
Albumin
Albumin is synthesized only in the liver, typically at a rate of 100 to 200 mg/kg/day in the adult, and under normal circumstances the plasma half-life is 3 weeks. Abnormalities are poorly specific for liver disease, however, because many factors affect its production and turnover. For example, nutritional state, plasma osmotic pressure, and thyroid levels all affect the rate of albumin production. The increased albumin losses seen in nephrotic syndrome, burns, and protein-wasting enteropathies also affect the balance between production and loss of albumin. Hypoalbuminemia can be helpful in assessing chronic liver disease when nonhepatic causes are excluded. Its prolonged half-life means that measured changes are slow to develop and slow to revert to normal in relation to the onset and resolution of the causative process.
Prothrombin Time
Prothrombin time (PT) determinations depend on serum concentrations of fibrinogen, prothrombin, and factors V, VII, and IX, all of which are products of the liver. Furthermore, the half-life of these factors is short enough (< 24 hours) that the PT changes rapidly. Given their short half life, factor V and VII levels can also be followed in evaluating liver function in fulminant liver failure. An abnormal PT can result from reduced factor synthesis (e.g., vitamin K deficiency, liver failure, warfarin therapy) or increased factor loss (e.g., disseminated intravascular coagulation).
Vitamin K deserves special mention in the context of the PT. Prothrombin and factors VII, IX, and X undergo posttranslational carboxylation of glutamic acid residues that is necessary for activity and requires vitamin K as a cofactor. Deficiency of vitamin K or antagonism of this process by warfarin (Coumadin) alters the PT. Additionally, in the jaundiced patient, a favorable response to parenteral vitamin K implies that intake or absorption of vitamin K is abnormal, versus a nonresponse, which implies that parenchymal disease is at least partly the basis for the abnormality (see Table 5-1 ).
Serum enzyme tests
Alkaline Phosphatase
Hepatic alkaline phosphatase (ALP) is concentrated in the canalicular hepatocyte membrane and bile duct epithelial cells, and increased production and release appear to cause the elevated ALP levels seen in cholestasis. However, ALP exists in normal tissues throughout the body as well as in extrahepatic neoplasms. Elevated levels are not specific for biliary disease because states of increased metabolic activity are associated with increased ALP activity in the affected tissue. Therefore, young adults with rapid bone growth and gravid patients with placental production routinely have elevated ALP levels. ALP levels as high as three times normal occur in many liver diseases. More pronounced increases suggest infiltrative processes or biliary obstruction, which can be intrahepatic (e.g., tumor) or extrahepatic. Even if the entire biliary tree is not obstructed, ALP can be greatly elevated.
Gamma-Glutamyl Transpeptidase
γ-Glutamyl transpeptidase (GGTP) has a tissue distribution similar to alkaline phosphatase except that it has low concentrations in bone. Thus, GGTP may be helpful in discriminating the source of ALP elevations. However, GGTP can also be quite sensitive to the ingestion of alcohol and drugs, including several anticonvulsants.
Transaminases (Aminotranferases)
Aspartate transaminase (AST, formerly known as SGOT [serum glutamic-oxaloacetic transaminase]; also called aspartate aminotransferase) and alanine transaminase (ALT, formerly SGPT [serum glutamic-pyruvic transaminase]; also alanine aminotransferase) are participants in gluconeogenesis. Both enzymes are plentiful in the cytosol of the hepatocyte, and an AST isozyme is present in the mitochondria as well. AST is also found in a variety of tissues, including heart, brain, and skeletal muscle; ALT is more specific to the liver. These enzymes are elevated in many forms of liver disease, presumably as a result of leakage from damaged cells. Substantial hepatic necrosis as found in chemical and ischemic injury appears to be particularly associated with elevation of these enzymes. Advanced cirrhosis can exist without significant elevations if active cell injury is absent or minimal at the time of evaluation.
The relative increase in AST compared with ALT can be useful in supporting a diagnosis of alcohol injury (AST/ALT > 2) versus most other acute liver injuries (AST/ALT ≤ 1), although cirrhosis is also associated with AST/ALT ratio greater than 1. Absolute levels can be diagnostic when extreme and helpful when moderately elevated (see Table 5-1 ).
Lactate Dehydrogenase
Because of its presence in tissues throughout the body, lactate dehydrogenase (LDH) usually offers little diagnostic discrimination beyond that of the transaminases. However, LDH does demonstrate a short-lived but exceptionally high elevation in ischemic injury, as well as a moderate but sustained increase in some malignancies.
Key Points
- ▪
No single test is specific for hepatic reserve.
- ▪
Hepatic clearance may be tested with ammonia and bilirubin levels.
- ▪
Bilirubin can be elevated secondary to hemolysis, hematoma resorption, and disorders of biliary transport.
- ▪
Synthetic function can be tested with albumin and prothrombin time.
- ▪
Albumin is made only in the liver, but many factors affect production and turnover.
- ▪
Abnormal PT can result from reduced factor synthesis or increased factor loss.
- ▪
Serum enzymes may reflect hepatocyte or injury, but none is isolated to the liver; GGTP is more specific for the liver than ALP.
Etiology of liver dysfunction
Liver dysfunction has been categorized in a variety of ways. Clinical presentation (e.g., jaundice), etiology (e.g., viral hepatitis), circumstances (e.g., postoperative liver dysfunction), time frame, and severity (e.g., subfulminant liver failure) are common descriptors, but none offers a complete description. For example, acute liver failure may have an infectious or toxic etiology, whereas viral hepatitis may result in abrupt, severe liver dysfunction or may proceed along a chronic, subclinical course.
Viral Hepatitis
Although a vast number of viruses can produce hepatitis ( Table 5-2 ), only five viruses produce liver disease as their primary clinical manifestation. Each of the five hepatitis viruses has been designated with a letter (e.g., hepatitis A, hepatitis B) according to their clinical manifestations ( Table 5-3 ). It is important to remember that although each virus infects the liver, the hepatitis viruses have different biochemical, biologic, and clinical characteristics. Indeed, the viruses do not form a phylogenetic family and are not actually related. Although infection with each virus can be associated with significant morbidity and mortality, infection with any virus may result in an anicteric illness and may not be diagnosed as hepatitis.
Virus | Vaccine Available |
---|---|
Epstein-Barr virus (EBV) | No |
Cytomegalovirus (CMV) | No |
Human herpesvirus type 1 (HHV-1) | In development |
Human herpesvirus type 2 (HHV-2) | In development |
Coxsackievirus type B | No |
Echoviruses | No |
Adenovirus | Yes, for certain subtypes;, limited to military use |
Yellow fever virus | Yes |
Varicella-zoster virus | Yes |
Measles | Yes |
Hepatitis | A | B | C | D | E |
---|---|---|---|---|---|
Virus family | Picornaviridae | Hepadnaviridae | Flaviviridae | Viroid | Caliciviridae |
Genome | ssRNA | Partially dsDNA | SsRNA | ssRNA | ssRNA |
Transmission: Fecal-oral Sexual | Yes No | No Yes | No Rare | No Rare | Yes No |
Blood/percutaneous | Rare | Yes | Yes | Yes | No |
Incubation period | 15-50 days | 4-26 wk | 2-26 wk | 3-7 wk | 15-60 days |
Immunity | IgG anti-HAV | IgG HBsAB | Unknown | IgG HBsAb | IgG anti-HEV |
Chronic hepatitis | No | Yes | Yes | Yes | No |
Fulminant failure | < 1% | < 1% | Rare | 2%-10% | 1% (30% in pregnancy) |
Cirrhosis | No | Yes | Yes | Yes | No |
Hepatitis A
The hepatitis A virus (HAV) is a 27 to 32–nm, nonenveloped virus (Picornaviridae) with a 7.5-kilobase genome of single-stranded (ss) RNA. HAV is almost always transmitted by the fecal-oral route after ingestion of contaminated food or drink. The virus is absorbed through the small bowel and transported by the portal blood flow to the liver. HAV replicates in the liver and is then shed into the blood or, more frequently, through the bile and into the stool. Viral shedding begins as early as the second week of infection and consequently may occur before the patient experiences any clinical signs or symptoms of hepatitis. Viral shedding may continue until 2 weeks after the onset of jaundice. Although the virus is shed into the stool in high titers, viral titers in the blood remain low during the short (1-2 weeks) viremic phase. As such, transmission of HAV by blood transfusion is extremely rare, although transmission from a single donor has been reported. The virus has also been transmitted to hemophiliac patients with contaminated factor VIII concentrates.
After an incubation of 15 to 50 days, patients may experience the acute onset of systemic complaints, including fever, malaise, nausea, vomiting, and abdominal pain. Patients may also note the appearance of dark urine and jaundice. Mild hepatic enlargement and tenderness is noted in approximately 85% of patients, with splenomegaly in up to 15%. Coagulopathy, encephalopathy, and renal failure are rare in patients with acute HAV infection. The infection is normally a self-limited illness, with complete recovery usually in less than 2 months; however, serious complications can occur. Underlying liver disease is associated with increased risk of fulminant hepatic failure with HAV superinfection. Chronic hepatitis does not occur, but an atypical, relapsing course has been described in both children and adults.
Diagnosis of HAV infection is usually confirmed by serologic testing. Anti-HAV immunoglobulin M (IgM) is detectable in the serum approximately 3 weeks after exposure. Early diagnosis is also possible with the detection of HAV in stool using electron microscopy or the detection of viral RNA; however, both methods are impractical. Although 75% of adult patients with HAV have clinical manifestations, up to 70% of infections in children younger than 6 years are asymptomatic. Their bowel hygiene and capacity to act as asymptomatic carriers make children the principal reservoir for the virus.
Hepatitis A infections occur throughout the world but are more common in developing countries with poor sanitation. In the United States, the incidence of HAV infection is 9 to 10 per 100,000 population, with an overall seroprevalence of 30%. Two highly effective vaccines available in the United States since 1996 are recommended for children and adults with chronic liver disease. Anesthesiologists and all health care providers should consider immunization. In the event of possible transmission of HAV to a health care provider, a single dose of 0.02 mL/kg immune globulin is highly effective in preventing infection if given within 14 days of exposure.
Hepatitis B
The hepatitis B virus (HBV) is a 42-nm, enveloped virus (Hepadnaviridae) with 3.2-kb genome of partially double-stranded (ds) DNA. Worldwide, more than 400 million people have chronic HBV infection. Unlike HAV, HBV is primarily transmitted by blood, blood products, and sexual contact. Perinatal infection can occur, with evidence of infection occurring across mucous membranes through semen, saliva, and breast milk. Intravenous (IV) drug use remains a major mode of HBV transmission, and outbreaks among IV drug abusers are frequently reported. Nosocomial transmission has occurred through use of multidose vials of local anesthetics. Acupuncture has been linked to occasional outbreaks of HBV infection. Fortunately, transfusion-related HBV infection is rare because HBV screening of donated blood has been routine for almost two decades. Nevertheless, an estimated 1:50,000 to 1:63,000 transfused units transmits HBV.
In the United States, Canada, Europe, and Australia, sexual transmission is the most important mode of HBV infection. Both heterosexual and homosexual activities can transmit HBV, but heterosexual activity accounts for the majority of HBV infections. Prostitutes, their clients, and individuals with many sexual partners are at an increased risk of HBV infection. The risk of heterosexual transmission is greater when the infected person is female than when male. In endemic regions such as China and sub-Saharan Africa, most HBV infections occur neonatally or in early childhood, and sexual transmission is less important.
After parenteral exposure, an asymptomatic incubation period ranges from 4 to 26 weeks (average, 6-8). During this period, infected hepatocytes synthesize and secrete large quantities of noninfective hepatitis B surface antigen (HBsAg). Consequently, HBsAg is detectable before the onset of signs and symptoms of hepatitis. Hepatitis B DNA (HBV-DNA) is detectable in the serum by polymerase chain reaction (PCR) shortly after HBsAg and indicates active viral replication. HBeAg, another important indicator of active viral replication, is also detectable at this time. Continued expression of HBeAg is an important biochemical predictor of progression to chronic hepatitis. IgM antibodies to hepatitis core antigen (HBc), a viral protein not detected in the serum, can be detected in the serum shortly before the onset of acute illness. IgM anti-HBc is gradually replaced by IgG anti-HBc over several months. IgG anti-HBs does not appear until after the resolution of jaundice and clinical symptoms and after the disappearance of HBsAg. During this “core window” after the disappearance of HBsAg and before the appearance of anti-HBs, IgM anti-HBc (and IgM anti-HBe) are the only laboratory markers of HBV infection.
Of the approximately 325,000 new HBV infections in the United States each year, approximately 60% of patients will develop subclinical disease without jaundice and completely recover. About 25% of infected patients develop acute hepatitis, characterized by fever, nausea, vomiting, anorexia, abdominal pain, and jaundice. Almost all patients who develop acute hepatitis recover completely, although about 1% of patients develop fulminant hepatic failure, which can be fatal without liver transplantation. From 5% to 10% of patients become “healthy carriers” of HBV disease. These individuals do not normally manifest signs or symptoms of hepatitis but are able to transmit the disease to others. Less than 5% of HBV-infected patients will develop a persistent infection, characterized by mild but persistent elevation of serum transaminases for months to years. Most patients with persistent infection ultimately recover; however, 20% to 30% will develop chronic hepatitis and cirrhosis. Patients who develop chronic hepatitis may have a defective immune response. HBV cirrhosis is a significant risk factor for hepatocellular carcinoma, and approximately 10% of patients with HBV cirrhosis will develop hepatocellular carcinoma.
Unlike HAV, HBV infection tends to be more severe in younger patients. In neonates and children younger than 1 year, risk of an infection becoming chronic is 90%. For children age 1 to 5 years, risk of chronic infection is 30%. For children older than 5 years, risk of chronic infection approaches that of adults. Transplacental passage of HBeAg from an infected mother to the fetus is thought to induce immune tolerance in the neonate.
Highly effective HBV vaccines have been available for more than 20 years. In 1991 the U.S. Centers for Disease Control and Prevention (CDC) recommended universal childhood vaccination against HBV in the United States. Broad-based vaccination initiatives have been effective in reducing the incidence of HBV infection in Alaska and reducing the incidence of hepatocellular carcinoma in Taiwan. Before HBV vaccination was widespread, the incidence of anti-HBs among anesthesiologists was greater than fourfold higher that of the general population. As such, the practice of anesthesiology is an independent risk factor for the development of HBV infection. All anesthesiologists should be vaccinated against HBV.
In the event of possible transmission of HBV to a nonimmunized individual (e.g., accidental needlestick), passive immunization with hepatitis B immune globulin (HBIG) is available. Current recommendations are to administer HBIG in a dose of 0.05 to 0.07 mL/kg immediately after exposure. A second dose 30 days after exposure may further reduce the risk of HBV infection. If HBIG is not given within 7 days of infection, antiviral treatment should be considered.
Most antiviral therapy in HBV is directed toward the treatment of chronically infected patients. Therapy with interferon alfa has proved effective in the elimination of HBeAg in patients with chronic infection. Therapy normally consists of a 16-week course of 5 mU daily or 10 mU three times weekly. Lamivudine, a nucleoside analog, is available orally for HBV therapy. Long-term lamivudine therapy reduces fibrosis and necrosis in patients with chronic HBV infection. Despite these impressive results, however, lamivudine-resistant mutants have emerged. Adefovir dipivoxil, another nucleoside analog, is also effective against HBV. Adefovir seems to have efficacy against lamivudine-resistant mutants.
Hepatitis D (Delta agent)
Hepatitis D virus (HDV) is a 35-nm viroid with a 1.7-kb genome of ssRNA. The viroid is enveloped with HBsAg and requires coinfection with hepatitis B virus for HDV infection and replication. Delta agent was first noted in 1977, and its unique structure was described in 1986. As with HBV, HDV is transmitted parenterally. IV drug abuse remains the most common mode of transmission in North America, Europe, and Australia. Sexual transmission of HDV can occur, but may be less efficient than for HBV. Perinatal infection of HDV is rare.
Hepatitis D infection can occur in two settings. In acute coinfection , HDV occurs at the same time as acute HBV infection, usually when a patient has been exposed to blood or serum from a patient harboring both infections. Superinfection can occur when a patient with a persistent HBV infection or chronic hepatitis becomes infected with HDV. Coinfection with HBV and HDV results in a more severe course of acute hepatitis and increased risk (3%-4%) of fulminant hepatic failure. Nevertheless, approximately 90% of coinfected patients have complete recovery and develop immunity. Secondary to defective immunity, patients with chronic HBV infection provide the ideal host for HDV superinfection. About 10% of patients superinfected with HDV develop fulminant hepatic failure that rapidly progresses. Most of the remaining 90% develop an accelerated cirrhotic picture. A small percentage of patients will recover and develop immunity.
The diagnosis of HDV infection is normally made by the detection of IgM anti-HDV. IgM anti-HDV is not normally detectable in patient serum until the onset of acute hepatitis and jaundice. It is possible to detect HDV antigen in patient serum before the onset of hepatitis during the late incubation period; however, HDVAg is present only transiently, and thus testing may be unreliable. HDV-RNA is the earliest marker of infection and can be detected by PCR, but this is rarely used to establish HDV infection.
There is no specific treatment for HDV infection. Because HDV infection is only possible in the case of HBV infection, and vaccination reliably prevents HBV infection, vaccination against HBV remains the best method to prevent HDV infection.
Key Points
- ▪
Hepatitis A virus is transmitted by the fecal-oral route, with rare progression to liver failure. Superinfection with underlying liver disease can result in hepatic failure, and vaccination is recommended.
- ▪
Hepatitis B virus is transmitted by body fluids; 60% of patients have subclinical disease, and 1% with acute HBV infection develop fulminant liver failure.
- ▪
About 1% of HBV patients develop chronic hepatitis and cirrhosis, with 10% risk of hepatocellular carcinoma.
- ▪
Superinfection of HBV-infected patients with hepatitis D increases the risk of fulminant hepatic failure.
- ▪
Hepatitis C is transmitted by body fluids; 80% of patients develop chronic hepatitis, 25% with cirrhosis.
Hepatitis C
The hepatitis C virus (HCV) is a 55-nm enveloped virus (Flaviviridae) with 9.4-kb genome of ssRNA. Worldwide, more than 170 million people have chronic HCV infection. HCV was not identified until 1989. As with HBV, HCV is primarily transmitted by blood, blood products, and sexual contact. The two main risk factors for HCV infection are IV drug use and blood transfusion before 1990. Indeed, HCV has been identified as the etiologic agent in more than 85% of all cases of posttransfusion “non-A, non-B” hepatitis before 1991. Since routine screening for anti-HCV and blood donor risk factor assessment by most blood donor centers in 1991, transfusion-related infection of HCV is a rare event. An estimated 1 in 103,000 transfused units transmits HCV.
Consequently, IV drug use has emerged as the principal risk factor for HCV infection in North America, Europe, and Australia. Perinatal transmission is rare and occurs exclusively from mothers who are HCV-RNA positive at delivery. Perinatal transmission may be more common if coinfection with human immunodeficiency virus (HIV) exists. It is unclear whether cesarean birth increases or decreases the risk of perinatal transmission. Breastfeeding appears to pose little risk to the infant. As noted earlier, sexual transmission of HCV is possible; however, transmission is significantly less efficient than for HBV. Nevertheless, prostitutes and their clients, men who have sex with men (MSM), and individuals with multiple sexual partners are at increased risk for HCV infection. Some suggest that coinfection with HIV or herpes simplex virus type 2 (HSV-2) may increase the likelihood of HCV infection. Although the virus is present in saliva of chronically infected persons, transmission through casual contact seems an unusual means of transmission. Patient-to-patient transmission has occurred during colonoscopy, and patients have been infected during surgery. In one hospital, an anesthesia assistant became infected from a patient and subsequently spread the infection to five other patients.
In contrast to HBV, HCV has a high rate of progression to chronic disease and eventual cirrhosis. After infection, HCV has a long incubation period that ranges from 2 to 26 weeks (average, 7-8 weeks). Of the approximately 175,000 persons infected in the United States each year, 75% will develop subclinical disease. The remaining 25% develop a symptomatic disease characterized by fever, nausea, vomiting, abdominal pain, anorexia, and jaundice. Approximately 1% of patients with symptomatic disease develop fulminant hepatic failure that rapidly progresses to death without transplantation. Almost 80% of all patients infected with HCV develop chronic hepatitis, characterized by mild, episodic elevations in transaminases and occasional jaundice. More than 25% of patients with chronic hepatitis develop cirrhosis. HCV cirrhosis is a significant risk factor for hepatocellular carcinoma, with an estimated risk of 1% to 4% per year.
The detection of antibodies against HCV is both sensitive and specific for HCV infection. Newer, third-generation enzyme immunoassays (EIAs) can detect antibodies within 4 to 10 weeks of infection. Unlike HBV, PCR to detect HCV-RNA is often used in clinical practice to determine viral load, a significant predictor of antiviral therapy efficacy. Detection of HCV-RNA is the most sensitive and specific test of HCV infection. Significant controversy surrounds the use of liver biopsy in HCV-infected patients.
Various treatment regimens are available for HCV infection. Standard interferon three times weekly for 24 to 48 weeks (approved in 1990) is successful in treating HCV infection. Interferon alfa (2a or 2b), 3 MU three times weekly for 24 to 48 weeks, has shown response rates as high as 40% and seems to be more effective than standard interferon. Pegylated interferons have been used to treat HCV since the late 1990s, with superior results compared with interferon alfa. When pegylated interferons are combined with ribavirin, studies have shown response rates as high as 88% in certain patient groups.
No vaccine is available for HCV infection. Therefore, avoiding exposure is the best prevention. For anesthesiologists and other health care professionals, adherence to universal precautions is critical. Prophylaxis after an accidental exposure is not currently recommended. There are no randomized, controlled trials examining the efficacy of therapy in acute HCV infection; however, one study showed that after treatment with interferon alfa-2b for 24 weeks, 43 of 44 patients did not have detectable HCV-RNA.
Hepatitis E
The hepatitis E virus (HEV) is a 32-nm, nonenveloped virus (Caliciviridae) with 7.5-kb genome of ssRNA. HEV was discovered in 1983 and is part of the alpha supergroup of viruses. HEV is responsible for the majority of cases of what was previously called “enterically transmitted non-A, non-B hepatitis” (ET-NANBH). As with HAV, HEV is almost always transmitted via the fecal-oral route through the ingestion of contaminated food or drink. During epidemics, the most common mode of transmission is the ingestion of water with fecal contamination. Compared with HAV, there is a low rate of person-to-person transmission of household contacts. Nosocomial infection has been reported. After ingestion, HEV is absorbed through the small bowel and transported by the portal blood flow to the liver. After an incubation period of 15 to 60 days (average, 35-42) a preicteric phase characterized by fever and malaise is reported by 95% to 100% of patients. An icteric phase characterized by abdominal pain, nausea, vomiting, anorexia, and jaundice follows shortly thereafter. Symptoms normally resolve in less than 6 weeks, although fulminant hepatic failure is a rare but reported complication. A characteristic feature of HEV is the high incidence of progression to fulminant hepatic failure in pregnant women. If contracted in the third trimester, HEV mortality may exceed 20%.
The diagnosis of HEV is normally made by exclusion after travel to an endemic area (South and Central America; Southeast Asia, including China, India, and Africa). Nevertheless, assays to detect both IgM anti-HEV and IgG anti-HEV are commercially available. PCR can be used to detect HEV-RNA; however, this is usually done for research purposes.
Currently, no vaccine is available for HEV infection. The administration of immune globulin from endemic areas has not decreased infection rates during epidemics. Health care providers should use universal precautions when dealing with patients with suspected HEV infection. Pregnant women should avoid any type of exposure to HEV.
Hepatitis G
Hepatitis G virus (HGV) was first described in the serum of a patient with “non-A, non-B, non-C” hepatitis. HGV and so-called GB viruses have been described. HGV and GB are detectable in a substantial number of blood donors and have a genomic sequence similar to HCV. However, HGV/GB does not appear to cause liver disease. Indeed, the initial patient was later found to have HCV infection. Interestingly, patients coinfected with HIV and HGV/GB seem to have prolonged survival.
Hydatid Cyst Disease
Hydatid cyst disease is caused by an infection of the animal tapeworm Echinococcus. As with all tapeworms, Echinococcus lives in the small bowel of hosts. Definitive hosts include carnivorous animals such as dogs, wolves, and other canines. Tapeworm-infected canines pass eggs in their feces, which contaminate the environment. Sheep, cattle, and humans become intermediate hosts when they ingest the eggs by eating contaminated foodstuffs. Infected domestic dogs remain the most important vector for transmission of hydatid disease. Once the eggs are ingested, gastric acid and digestive pancreatic enzymes dissolve the egg’s external shell. The larvae then penetrate the bowel wall, enter the portal circulation, and are carried to the liver. Approximately 70% of the larvae remain in the liver, with 20% infecting the lungs, although other organs can be infected, including the brain, spinal cord, kidney, and heart.
In the liver, Echinococcus infection results in virtually no symptoms until the cysts become very large. Although pain is the most common complaint, a large cyst may cause obstructive jaundice, cholangitis, pancreatitis, or portal hypertension. Blunt trauma may cause cyst rupture. Diagnosis is normally made by serologic testing after abdominal imaging reveals hepatic cysts. An eosinophilia may also be present.
The treatment of large hydatid cysts is surgical, and the anesthesiologist is likely to encounter patients scheduled for cyst drainage. The surgical approach may be attempted by laparoscopy, laparotomy, or thoracotomy if a subdiaphragmatic cyst is present. There are multiple case reports of an anaphylactic reaction to hydatid fluid during surgical excision. Preoperative steroids and antihistamines should be considered.
Nonalcoholic Steatohepatitis
Although the incidence of chronic viral or alcoholic hepatitis has not increased significantly in the past few years, the overall number of patients with liver dysfunction has increased secondary to the well-recognized obesity epidemic. Nonalcoholic steatohepatitis (NASH) is becomingly increasingly recognized as the most common cause of liver disease in the United States. Few of these patients progress to cirrhosis but often have moderate degrees of dysfunction. The diagnosis of NASH is based on a liver biopsy showing steatosis that is often indistinguishable from alcoholic liver disease ( Fig. 5-7 ), evidence of negligible alcohol consumption, and absence of HBV or HCV infection. The etiology of NASH is unknown, but it is often associated with obesity, type 2 diabetes, and hyperlipidemia.

Patients may present with fatigue, malaise, and right upper quadrant discomfort with elevated enzyme levels. Most patients have only increased enzymes, but a few will progress to cirrhosis. To date, no good predictors of disease progression exist, but presence of inflammation on biopsy, diabetes, and high AST may be associated factors. NASH has a better overall prognosis than alcoholic liver disease, with fewer patients progressing to transplantation and improved 10-year survival. However, NASH is associated with hepatocellular carcinoma. There is no proven therapy, although weight loss may result in improved enzyme levels and histologic findings. Modification of risk factors for hyperlipidemia and diabetes is also recommended.
Genetic Causes of Liver Disease
Alagille’s syndrome (arteriohepatic dysplasia)
Alagille’s syndrome (AGS) is a rare inherited disorder characterized by the progressive loss of the intralobular bile ducts and narrowing of extrahepatic bile ducts. It is the most common form of familial intrahepatic cholestasis, and more than 90% of patients experience chronic cholestasis. AGS has an autosomal dominant pattern of inheritance, and more than 90% of patients have a mutation in the jagged 1 (JAG1) gene on the short arm of chromosome 20; others have a mutation in NOTCH-2. AGS has an incidence of approximately 1:100,000 live births. Most patients present with jaundice, clay-colored stools, and other symptoms of mild cholestasis during the neonatal period. Patients might also present with rapidly progressive, fulminant hepatic failure. AGS is slowly progressive, and treatment is generally supportive. Approximately 15% of patients will require transplantation. A Kasai procedure is generally not considered beneficial and thus is not recommended, especially because the risk during liver transplantation may increase with previous Kasai surgery.
Although the primary manifestation is cholestasis, AGS is of particular interest to anesthesiologists because of the high morbidity of its associated conditions. More than 90% of patients with AGS have congenital heart disease. Approximately 67% of patients have uncomplicated peripheral pulmonic stenosis; however, the remaining 33% have more serious defects, including tetralogy of Fallot (16%), patent ductus arteriosus (5%), ventricular septal defect (4%), and atrial septal defect (4%). The presence of significant cardiovascular disease is associated with increased perioperative mortality during liver transplantation. As many as 85% of patients have “butterfly vertebrae” resulting from clefting abnormalities. Patients are described as having a characteristic facies, and as many as 90% of patients have ophthalmologic abnormalities, usually anterior chamber defects. The facial features are defined by a broad nasal bridge, triangular facies, and deep-set eyes, but are not specific to Alagille’s syndrome. Patients have a characteristically short stature, and resistance to growth hormone has been described.
A meticulous preoperative evaluation of patients with arteriohepatic dysplasia is critical for perioperative planning and optimal care. Severity and type of dysfunction can be highly variable, so associated conditions are evaluated, with particular attention to each patient’s cardiac, hepatic, renal, and orthopedic disease. In some patients, vitamin K deficiency results from malabsorption. If blood loss is possible, preoperative clotting studies may be indicated. Malnutrition can be a major concern, and proactive treatment with high-energy supplements and fat-soluble vitamins is recommended. Severe postoperative cholestasis has been reported in patients with Alagille’s syndrome.
Alpha 1 -antitrypsin deficiency
Deficiency of α 1 -antitrypsin is the most common metabolic disease affecting the liver. The disease is most common among white Europeans, in whom the incidence may be as high as 1 in 1500 persons. The disease is somewhat less common among North American and Australian whites, about 1 in 2000 persons. The incidence among African, Asian, and Hispanic individuals is low. The precise geographic distribution depends on the specific genotype.
A potent serine protease inhibitor, α 1 -antitrypsin is synthesized in the liver and secreted into the blood. As it circulates, it binds to and promotes the degradation of serine proteases produced throughout the body. One of the most important proteases inhibited by α 1 -antitrypsin is elastase. Indeed, α 1 -antitrypsin is responsible for more than 90% of all the serum antielastase activity and is principally involved in the degradation of alveolar elastase. Once bound to its protease target, the α 1 -antitrypsin/protease complex binds to a receptor on hepatocytes and is removed from the circulation.
The α 1 -antitrypsin gene has been localized to chromosome 14 and is part of the serine protease inhibitor (SERPIN) supergene. This gene cluster also encodes for corticosteroid-binding globulin, C1 inhibitor, and antithrombin III. At least 17 different mutant alleles of α 1 -antitrypsin have been described; however, two mutations account for the majority of disease. Individuals homozygous for the more common S mutation (Glu264Val) have a 40% decrease in serum α 1 -antitrypsin concentration. The S mutation is more common among Southern Europeans, with peak incidences recorded in the Iberian peninsula. Individuals homozygous for the more serious Z mutation (Glu342Lys) have an 85% decrease in serum α 1 -antitrypsin concentration. Unlike the S mutation, the Z mutation is more common among Northern and Western Europeans, with peak incidences in northern France, the United Kingdom, and Scandanavia. In general, the S mutation only produces clinically significant disease when combined with the Z mutation (SZ genotype).
The low serum protein concentrations observed in individuals with α 1 -antitrypsin deficiency do not occur secondary to defective protein synthesis, but rather to ineffective processing and secretion. These ineffective processes leave the hepatocyte with large quantities of defective protein that accumulate in the cell. Defective processing is particularly severe in the Z mutation, where processing errors lead to the formation of long polymers of Z–α 1 -antitrypsin. In both mutations, the excess of defective α 1 -antitrypsin is visible under light microscopy as large cytoplasmic inclusions. Stores of excessive defective protein ultimately can interfere with normal hepatic function.
The abnormal accumulation of defective protein leads to hepatocyte death and eventual cirrhosis. In general, the severity of hepatic disease is closely associated with the amount of accumulated protein. Liver disease does not occur in patients with unusual mutations of α 1 -antitrypsin that do not result in the accumulation of defective protein in the hepatocyte. Clinical presentation and age at onset vary significantly among patients with α 1 -antitrypsin deficiency, even among individuals with the same genotype. The variation in age at onset of liver disease may result from variations in the rate of synthesis between individuals. Indeed, the appearance of jaundice in infants with ZZ α 1 -antitrypsin deficiency may reflect a chronic infection resulting in increased synthesis of defective protein. Regardless, the appearance of jaundice during the neonatal period is a poor prognostic sign. Although α 1 -antitrypsin deficiency has other manifestations, it is well accepted that liver disease most influences survival. The other primary clinical manifestations occur secondary to the absence of normal protease inhibition, which is most apparent in the lung; patients with α 1 -antitrypsin deficiency have early onset of panlobular emphysema. All individuals experience an age-related decline in the forced expiratory volume in 1 second (FEV 1 ) after age 30; however, this decline is accelerated by α 1 -antitrypsin deficiency. This acceleration is further exacerbated by tobacco smoke, which can double the rate of decline.
The diagnosis is made by the measurement of serum α 1 -antitrypsin concentration. The genotype is confirmed by protein electrophoresis. There is no specific therapy for α 1 -antitrypsin deficiency, and liver transplantation may be required.
Cystic fibrosis
Cystic fibrosis (CF) is the most common lethal inherited disease among white populations, with an incidence of approximately 1 in 3300 persons in the United States. CF was one of the first genetic diseases to be characterized. The gene for CF, the cystic fibrosis transmembrane conductance regulator (CFTR), resides on chromosome 7. Presence of the gene results in defective cellular chloride conductance. Although the principal manifestation of CF is pulmonary with associated viscid secretions, atelectasis, emphysema, and chronic infections with Pseudomonas aeruginosa, hepatic abnormalities may complicate 20% of cases. Portal hypertension and eventual hepatic cirrhosis may complicate up to 10% of all CF cases and represent the second most common cause of death after respiratory failure. As the median age of CF patients increases secondary to a reduction in mortality, the concern is increased incidence of liver disease.
Although pathologic elevation of liver enzymes is frequently observed in infants, most patients with CF do not progress to cirrhosis. Nevertheless, certain genotypes are clearly associated with liver dysfunction and an increased incidence of cirrhosis. There is also an increased incidence of liver disease in patients with certain major histocompatibility complex (MHC) genotypes, male gender, coexisting liver disease, and poor nutrition (especially fatty acid deficiency). Major liver disease is rarely noted in the absence of pancreatic insufficiency. When hepatic disease advances to cirrhosis, it normally presents during the first decade of life. Portal hypertension is usually manifested by splenomegaly, hypersplenism with thrombocytopenia, and ascites. Bleeding of esophageal varices is also noted in some patients.
Transjugular intrahepatic portosystemic shunt (TIPS) has been used with success in children and adolescents with refractory esophageal bleeding. In severe cases, liver transplantation has been performed. The anesthesiologist should be aware that the metabolism of certain drugs may be increased in CF secondary to increased hepatic drug clearance.
Galactosemia
Galactosemia is an inherited deficiency of the enzyme galactose-1-phosphate uridyltransferase, which catalyzes the conversion of galactose-1-phosphate to UDP-galactose; deficiency leads to the abnormal accumulation of galactose-1-phosphate in cells. The enzyme is normally present in liver and erythrocytes. Galactosemia is a rare disorder, approximately 1 in 60,000 live births. Galactose-1-phosphate is directly toxic to cells, and accumulation is most notable in the kidney, liver, and brain. Breast milk contains lactose, a disaccharide consisting of glucose and galactose. As newborn infants receive up to 20% of their caloric intake in the form of lactose, infants with galactosemia rapidly accumulate galactose-1-phosphate. Routine newborn screening normally makes the diagnosis of galactosemia. If the diagnosis is not made, the accumulation of galactose-1-phosphate can ultimately lead to cataracts, severe mental retardation, and cirrhosis.
Treatment involves the avoidance of lactose in the diet; however, patients treated appropriately still develop long-term complications, including cognitive impairment, cataracts, speech abnormalities, and primary ovarian failure. Infants born with galactosemia have an increased incidence of Escherichia coli neonatal sepsis that normally precedes the diagnosis of galactosemia. Without treatment, the disease is generally fatal, although case reports of adult patients presenting with decompensated cirrhosis exist. Galactokinase deficiency, another inherited disorder of galactose metabolism, is less common than galactosemia and generally has a milder course.
Galactosemia may present the anesthesiologist with several unique challenges. Newly diagnosed newborns who have been treated for a short time may have elevated clotting times and may be prone to bleeding. Some patients may have hemolysis, and preoperative evaluation of hemoglobin may be valuable in any jaundiced patient. Also, albuminuria may cause an osmotic diuresis, and thus urine volume may be a poor indicator of intravascular volume.
Key Points
- ▪
Infants with galactosemia may have elevated clotting times, hemolysis, and albuminuria.
- ▪
Glycogen storage disorders types I, II, and IV are associated with severe hepatic disease; type III with muscular disease; and type IV with cardiomyopathy.
- ▪
Careful glucose monitoring is mandatory in patients with type I or III glycogen storage disease.
Glycogen storage diseases
Glycogen is the principal storage form for glucose in the human body. It is composed of long chains of glucose joined together by α-1,4 linkages. The chains intermittently branch to form long, treelike strands of stored glucose. Glycogen stands as a ready reserve for glucose in times of metabolic need. Glycogen metabolism principally occurs in skeletal muscle and liver. Skeletal muscle glycogen provides exercising muscles with a ready source of fuel while hepatic glycogen serves to maintain plasma glucose during fasting. The glycogen storage disorders comprise a family of 10 different diseases. Each disease is characterized by a glycogen metabolism enzyme deficiency. Only glycogen storage disorder types I, III, and IV are associated with severe hepatic disease ( Table 5-4 ).
Disease | Enzyme Deficiency | Main Clinical Features | Liver Disease | Treatment | Notes |
---|---|---|---|---|---|
Type Ia (von Gierke’s disease) | Glucose-6-phosphatase | Profound hypoglycemia Growth failure Metabolic acidosis Hyperlipidemia Renal failure (by second decade of life) Diagnosis in infancy | Hepatomegaly (normal spleen) Hepatic adenomas (by second decade of life) Occasional hepatocellular carcinoma | Portal diversion shunting Glucose supplements (cornstarch, nocturnal glucose infusion) Liver transplantation | Type Ib (10% of type I disease) also associated with neutropenia and neutrophil dysfunction |
Type IIIa (Cori-Forbe’s disease) | Liver and muscle debranching enzyme | Profound hypoglycemia Growth failure Progressive muscle weakness with activity Muscle atrophy More tolerant to fasting than type I Diagnosis in infancy | Hepatomegaly (normal spleen) Hepatic adenomas (less common than type I) Rare hepatocellular carcinoma | High-protein, low-carbohydrate diet Glucose supplementation (cornstarch, nocturnal glucose infusion) rarely necessary | Type IIb (15% of type III) has normal muscle debranching enzyme and no muscular symptoms Generally improves with age |
Type IV (Andersen’s disease) | Branching enzyme | Failure to thrive Abdominal distention Miscellaneous gastrointestinal complaints Cardiomyopathy Hypoglycemia rare Diagnosis in infancy | Hepatosplenomegaly Progressive macronodular cirrhosis Hepatic failure | Death without liver transplantation | Rare |
The perioperative management of any patient with a glycogen storage disorder requires meticulous care and planning. Obviously, the blood glucose level should be carefully monitored in any patient with a type I or III glycogen storage disorder. Nothing by mouth (NPO) guidelines should be followed, and patients may require preadmission for IV administration of glucose-containing fluids. Case reports of successful anesthetic management of patients with a type I glycogen storage disease have been reported. Patient-controlled sedation with propofol during spinal anesthesia has also been successfully employed. Patients with a type III glycogen storage disease may pose a special challenge to anesthesiologists secondary to muscle disease. Liver transplantation has been used to treat type I, III, and IV glycogen storage diseases, but cardiomyopathy may persist in type IV secondary to cardiac amylopectin deposition.
Hereditary fructose intolerance
Hereditary fructose intolerance (HFI) is an inherited deficiency of the enzyme fructose-1,6-bisphosphate aldolase (aldolase B). Aldolase B catalyzes the conversion of fructose-1,6-bisphosphate to two triose phosphates, dihydroxyacetone phosphate and glyceraldehyde-3-phosphate. Deficiency leads to the abnormal accumulation of fructose-1-phosphate and initiates severe symptoms when patients are exposed to fructose. The enzyme is normally present in liver, kidney, and small bowel. HFI is a rare disorder, with an incidence of approximately 1 in 23,000 live births.
When patients consume fructose or sucrose (a disaccharide consisting of glucose and fructose), the acute presentation of abdominal pain, malaise, hypoglycemia, nausea, and vomiting is often noted. Continued ingestion of fructose yields jaundice, hepatomegaly, and renal dysfunction. Persistent fructose consumption results in fulminant hepatic failure. Treatment consists of the avoidance of fructose and sucrose in the diet. Unlike galactosemia, patients are normally without symptoms if fructose is avoided, and intellectual development is unimpaired. Some investigators believe that HFI is underdiagnosed, and formal testing yields the diagnosis among patients with unexplained, chronic abdominal pain. Secondary to an almost-complete absence of dietary sucrose, patients with HFI have excellent dentition. Oral medications containing sucrose or fructose are avoided in patients with HFI.
Hereditary hemochromatosis
Hereditary hemochromatosis is an autosomal recessive disease characterized by an inappropriately high degree of iron absorption. In the past, it had been theorized that the disorder occurred due to alcohol abuse and was merely a secondary nutritional disorder; however, the gene was later found to reside on the short arm of chromosome 6, closely linked to the genes encoding for human leukocyte antigen (HLA). It was not until 1996 that the gene responsible for hemochromatosis (HFE) was discovered, allowing for formal genetic testing and diagnosis. A variety of conditions, both acquired and idiopathic, can be characterized by excessive total body iron ( Box 5-2 ). In many cases, these diseases mimic hereditary hemochromatosis and may be superficially indistinguishable in their clinical manifestations. Nevertheless, it is universally accepted that hereditary hemochromatosis refers specifically to increased iron absorption secondary to HFE -related genetic mutations.
Primary Iron Overload
Hereditary hemochromatosis (HFE)
Non- HFE related
Juvenile hemochromatosis
Transferrin receptor-2 mutations
Ferroportin-1 mutation
African iron overload
Secondary Iron Overload
Red blood cell transfusions
Iron-loading anemias
Thalassemia major
Sideroblastic anemia
Chronic hemolytic anemia
Aplastic anemia
Pyruvate kinase deficiency
Long-term dialysis
Chronic liver disease
Hepatitis B
Hepatitis C
Alcoholic liver disease
Nonalcoholic steatohepatitis
Portocaval shunting
As specific HFE mutations are identified and investigated, it has become increasingly clear that hereditary hemochromatosis represents a spectrum of clinical disease. Indeed, some homozygotes may manifest disease without a substantial increase in iron stores, whereas others do not manifest clinical symptoms in any appreciable way. In addition, although HFE is equally distributed between the genders, clinical disease is two to eight times more common in men than women. Patients with hereditary hemochromatosis can be classified into four groups: (1) genetic predisposition without abnormalities, (2) iron overload without symptoms, (3) iron overload with early symptoms, and (4) iron overload with end organ damage. Other factors, genetic and environmental, certainly influence the development of clinical disease. The early observation of the link among hereditary hemochromatosis, cirrhosis, and alcohol abuse may be explained by alcohol further increasing iron absorption.
The normal adult has a total body iron content of 3 to 5 g. Most iron is recycled through the phagocytosis of senescent erythrocytes, and only 1 to 2 mg of iron is normally lost each day. Losses may be greater among menstruating women and in the case of acute or chronic blood loss. Consequently, dietary iron absorption is tightly regulated, with the amount absorbed paralleling the body’s needs. In hereditary hemochromatosis, regulatory processes fail. This results in an abnormal increase in dietary iron absorption with iron deposition in the skin, heart, pancreas, joints, and liver. Hereditary hemochromatosis is surprisingly common. In some white European populations, 10% to 12% of people are heterozygous carriers of the disease. The incidence of homozygous hereditary hemochromatosis ranges between 1:100 and 1:400 in whites of European descent.
The primary presentation of symptomatic hereditary hemochromatosis is becoming rare. Most patients are asymptomatic and report for evaluation and genetic testing after a family member develops the disease. Nevertheless, most symptomatic patients present in the fifth or sixth decade of life. The liver is the first organ to be affected in hemochromatosis, and hepatomegaly is noted in almost 100% patients. The most common presenting symptoms include generalized weakness, malaise, arthralgias, abdominal pain, and impotence (in men). Physical examination may reveal hepatomegaly and, in advanced cases, signs and symptoms of cirrhosis, including ascites and jaundice. Diabetes mellitus, secondary to pancreatic iron deposition, may also occur, although it is rare in the absence of cirrhosis. Iron deposition in skin may give patients a bronze coloration; hemochromatosis has been referred to as “bronze diabetes.” Iron deposition in the heart can lead to fibrotic changes and most often to a restrictive cardiomyopathy. An increase in fatal and nonfatal arrhythmias is also noted. An arthropathy, especially of the hands, is noted in about 50% of patients but does not usually present before age 50.
As iron accumulates in the liver, significant hepatocyte damage occurs. The fundamental disease mechanism results from direct iron toxicity and the consequent increase in iron-generated free-radical production. The increased oxidative stress results in lipid peroxidation, mitochondrial injury, and impaired calcium homeostasis. This results in an inflammatory response, fibrin deposition, and ultimately cirrhosis. Further oxidative stress may result in DNA damage and an increased risk of hepatocellular carcinoma. Hepatocellular carcinoma is the most common cause of death in patients with hereditary hemochromatosis, and the risk is 200 times greater than in the general population. Complications arising from cirrhosis and congestive heart failure are other common causes of death.
Once the diagnosis of hereditary hemochromatosis is made, treatment with phlebotomy and reduction in alcohol and dietary iron intake should be initiated. The goal of therapy is to make the patient non–iron deficient or nonanemic. Thus, careful monitoring of hemoglobin, iron levels, ferritin, and transferrin saturation should guide therapy. Although phlebotomy and careful monitoring of dietary intake effectively reduce iron stores, therapy does not reverse cirrhosis or totally eliminate the risk of hepatocellular carcinoma. This is especially true among patients diagnosed at an advanced age. As such, early diagnosis and treatment, ideally before the onset of symptoms, is critical. Liver transplantation may represent the only treatment in patients with advanced disease or with hepatocellular carcinoma; however, many studies reveal decreased survival in transplant patients with hereditary hemochromatosis compared with other indications.
Hereditary tyrosinemia type 1
There are four known deficiencies in the catabolism of tyrosine: alkaptonuria and tyrosinemia types 1, 2, and 3. Only hereditary tyrosinemia type 1 (HT-1) is associated with liver disease. HT-1 is an inherited deficiency of the enzyme fumarylacetoacetate hydrolase (FAH). The enzyme catalyzes the final step in phenylalanine and tyrosine catabolism, the conversion of fumarylacetoacetate to acetoacetate and fumarate. FAH deficiency leads to the abnormal accumulation of “upstream” tyrosine metabolites fumarylacetoacetate (FAA) and maleylacetoacetate (MAA). Both FAA and MAA are converted to two toxic products, succinyl acetoacetate (SAA) and succinylacetone (SA). SAA and SA interfere with DNA ligase activity, reduce blood and liver stores of glutathione, and interfere with heme metabolism. These effects combine to decrease the body’s ability to deal with oxidative stress and directly result in mutagenic damage and chromosomal breakage. Initially, liver biopsy reveals steatohepatitis; however, this advances to fibrosis and cirrhosis.
Although a rare disorder affecting approximately 1 in 100,000 births, the incidence of HT-1 may be higher in northern Europe (1:8000) and in Quebec, Canada (1:1846). Essentially, two forms exist, acute and chronic. In acute HT-1, patients present with symptoms of severe hepatic dysfunction during the first 6 months of life. Liver biopsy reveals steatohepatitis that advances to fibrosis and micronodular cirrhosis with bile duct proliferation. In general, the acute form is rapidly fatal within the first year of life without hepatic transplantation. The chronic form of HT-1 presents more slowly, with patients rarely seeking medical care before age 1 year. The progress of hepatic dysfunction tends to occur more slowly, and patients develop other symptoms, including nephropathy, rickets, and serious neurologic problems. Secondary to continued DNA damage, a substantial risk of hepatocellular carcinoma exists. Liver biopsy reveals less cholestasis than the acute form; however, macronodular and micronodular cirrhosis are eventually noted.
Liver transplantation is normally indicated within the first decade of life in patients with chronic HT-1; however, advances in treatment are promising. Patients may develop hypertrophic cardiomyopathy. Anemia, thrombocytosis, and prolonged clotting times may be observed. No specific information addresses anesthetic care in patients with HT-1, although preoperative assessment of cardiac, hepatic, metabolic, and hematologic function should be considered.
Lysosomal storage diseases
Lysosomal storage diseases are a heterogenous group of diseases resulting from different defects in lysosomal function. Each disease normally reflects a lysosomal enzyme deficiency and a consequent inability to metabolize various biomolecules. Most diseases follow an autosomal recessive pattern of inheritance. Of the more than 30 well-classified diseases, only a small number result in hepatic impairment.
Mucopolysaccharidoses
Each mucopolysaccharidosis (MPS) results from the deficiency of an enzyme responsible for glycosaminoglycan (GAG) metabolism. GAGs are complex, long-chain carbohydrates that are normally linked to proteins to form proteoglycans. Proteoglycans are common constituents of connective tissue.
Mucopolysaccharidosis type I (MPS-I) results from the deficiency of α- l -iduronidase. At least three phenotypes exist. MPS-IH (Hurler’s disease) has an acute course characterized by hepatosplenomegaly, mental retardation, and death normally occurring in the first decade. MPS-IS (Scheie’s disease) has a less severe course characterized by hepatosplenomegaly after age 5 years and normal life span without mental retardation. MPS-IH/S (combined Hurler/Scheie) follows an intermediate course. In all these MPS-I diseases, hepatosplenomegaly can be massive, with profound skeletal dysplasia. Myocardial, coronary, and valvular heart disease are common. Corneal “clouding” is an expected complication, and patients may present for corneal transplant. Patients frequently require surgical intervention for orthopedic abnormalities. A stiff neck, large tongue, and tonsillar hypertrophy may make intubation difficult. Copious airway secretions may be treated with anticholinergics. Fiberoptic intubation through a laryngeal mask airway (LMA) may represent a useful technique, especially in children. Patients should be considered at risk for airway obstruction and postobstructive pulmonary edema. Failure of epidural anesthesia has been reported and may be related to the accumulation of glycosaminoglycans (GAGs) in the epidural space. Perioperative antibiotics may be indicated in patients with valvular disease.
Mucopolysaccharidosis type II (MPS-II, Hunter’s disease ) results from the deficiency of iduronate sulfatase and has an X-linked recessive pattern of inheritance. Both a severe infantile and a mild juvenile form of the disease exist. In addition to massive hepatosplenomegaly, GAGs accumulate in the head and neck, and patients have a short neck and large tongue. Unlike MPS-I, corneal disease is rare. Nevertheless, endotracheal intubation can be difficult, and acute airway obstruction has been reported. Failure of the LMA to secure the airway in a patient with MPS II has been reported. Again, however, fiberoptic LMA intubation may be useful in children, and sleep apnea and pulmonary edema have been reported.
Mucopolysaccharidosis type VII (MPS-VII, Sly’s syndrome ) results from the deficiency of β-glucuronidase and has an autosomal recessive pattern of inheritance. At least four phenotypes exist. The neonatal form of MPS-VII presents as hydrops fetalis and is uniformly fatal. An infantile form presents as hepatosplenomegaly, jaundice, and inguinal and umbilical hernias. It is rapidly progressive and has a poor prognosis. A second infantile form also presents as hepatosplenomegaly, but seems to have a milder course. The adult form of MPS-VII presents in adolescence and is not normally complicated by hepatic involvement. Patients may have cardiac involvement with mitral and aortic insufficiency. Acute aortic dissection has been reported. Because of the accumulation of GAGs in the head and neck, patients with Sly’s syndrome may also be difficult to intubate, although this has not been specifically reported with MPS-VII. Intraoperative complete heart block has been observed. Patients with aortic or mitral insufficiency may require perioperative antibiotic prophylaxis.
Lipid storage disorders
Each of the lipid storage disorders results from the deficiency of an enzyme responsible for lipid metabolism. The lipid storage disorders include Fabry’s disease, Gaucher’s disease, and Niemann-Pick disease; only the latter two have hepatic manifestations and are discussed here.
Gaucher’s disease (GD) results from the deficiency of acid β-glucosidase and has an autosomal recessive pattern of inheritance. GD is the most common lysosomal storage disease. Three phenotypes have been described: adult, infantile, and juvenile. Adult Gaucher’s disease (GD type 1) represents 99% of cases and has a variable onset. It is characterized by thrombocytopenia, anemia, and hepatosplenomegaly. Bone pain is a common complaint, and pathologic fractures can occur. Although hepatosplenomegaly may be the most prominent feature, most morbidity results from bone pain. Intelligence is normal, and neurologic symptoms are rare. The availability of placental and now recombinant glucocerebrosidase has improved morbidity in many patients and can result in decreased liver volume. Blood coagulation abnormalities have also improved. Adult GD has a carrier rate of approximately 1:18 among Ashkenazi Jews and an annual incidence of approximately 1:1000 live births in the United States.
The accumulation of glycosphingolipids in the head and neck may make endotracheal intubation difficult, and patients may require a smaller-than-predicted endotracheal tube. Patients should be considered at risk for upper airway obstruction. A small mouth may make LMA insertion difficult. Preoperative evaluation should include a baseline hemoglobin and platelet count, because patients are at risk for anemia and thrombocytopenia. Spinal anesthesia has been used with success.
Infantile Gaucher’s disease (GD type 2) is characterized by hepatosplenomegaly and severe developmental delay. Stridor and laryngospasm are frequent complications. The disease progresses rapidly, and death occurs before age 2. Juvenile Gaucher’s disease (GD type 3) is characterized by ataxia, hepatosplenomegaly, and mental retardation. The typical onset occurs during childhood, and patients normally die before age 15. Juvenile GD has a peak incidence in the Swedish Norrbotten population, with an incidence of 1 in 50,000 persons. Gastroesophageal reflux and chronic aspiration can complicate both type 2 and type 3 GD. As in type 1 GD, the airway management of patients with types 2 and 3 may be difficult, and patients are at risk for postoperative respiratory compromise. Regional anesthesia has been used with success and should be considered.
Niemann-Pick disease (NPD) results from the deficiency of sphingomyelinase and has an autosomal recessive pattern of inheritance. At least six phenotypes of NPD have been described, but three types (A, B, and C) make up the majority of cases. Infantile neuropathic NPD (NPD type A) normally presents before 6 months of age and is characterized by hepatosplenomegaly, lymphadenopathy, seizures, and mental retardation. A progressive loss of intellectual capacity and motor function results from increased deposition of sphingomyelin in the central nervous system (CNS). Non-neuronopathic NPD (NPD type B) has a variable age of presentation and a more heterogenous expression. Nevertheless, most patients are diagnosed in childhood with hepatosplenomegaly. Unlike type A, patients with type B NPD are neurologically intact, and systemic deposition of sphingomyelin is more prominent. Hepatic cirrhosis may develop, and portal hypertension and ascites can complicate the disease. Many type B patients develop pulmonary disease characterized by severe diffusion limitations. Such patients may have low Pa o 2 and develop cor pulmonale and right ventricular failure in the second decade of life. Patients with type C NPD have a deficiency in cholesterol transport that leads to a disease that is phenotypically similar to types A and B NPD. Patients with type C disease present with prolonged neonatal jaundice. Hepatosplenomegaly is less severe than in types A and B, and patients normally undergo slowly progressive neurodegeneration.
Airway management may be more difficult in patients with NPD. Pulmonary disease may complicate perioperative care, especially in individuals with type B disease. Liver transplantation has successfully reduced some of the clinical manifestations in patients with type A and B NPD. However, morbidity and mortality of liver transplantation may be extremely high secondary to pulmonary and neurologic disease.
Other lysosomal storage diseases
Mannosidosis results from the deficiency of α-mannosidase. An infantile form of the disease is characterized by progressive mental retardation and hepatosplenomegaly. Cataracts and corneal clouding may also be observed. An adult form has a delayed onset and allows for longer survival. A small mouth and a large tongue may make intubation difficult. Death normally occurs before age 5. An autosomal recessive pattern of inheritance is noted in this rare disease.
Wolman’s disease results from the deficiency of acid lipase and is characterized by the deposition of cholesterol esters throughout the body. Hepatosplenomegaly and eventual cirrhosis are among the more prominent manifestations; however, pulmonary disease with a high alveolar diffusion gradient may be severe. Adrenal calcification is a unique feature. Neonatal survival is impossible without total parenteral nutrition, and death occurs within the first year of life. Bone marrow transplantation has been used successfully to treat Wolman’s disease. No specific information is available on anesthesia in patients with Wolman’s disease.
Porphyria
The porphyrias make up a family of inherited diseases resulting from deficiencies in one or more of the enzymes required for heme synthesis. The enzymatic deficit results in the accumulation of “upstream” metabolites and consequent symptoms ( Fig. 5-8 ). More than 75% of heme synthesis occurs in the bone marrow, and thus porphyrias are associated with variable hepatic disease. Traditionally, porphyrias are generally divided into erythropoietic or hepatic types, depending on whether the excess production of metabolic intermediates takes place in the liver or in the bone marrow. Porphyrias can be further divided into those with neurovisceral symptoms ( acute porphyrias) and those characterized by photosensitivity and cutaneous symptoms ( cutaneous porphyrias). Table 5-5 summarizes the characteristics of the various porphyrias.

Disease | Site/Clinical Features | Enzyme Affected | Inheritance | Major biochemical findings * | |||
---|---|---|---|---|---|---|---|
Urine | Plasma | RBCs | Feces | ||||
ADP | Hepatic Δ /Acute | ALAD | Autosomal recessive | ALA, copro III | ALA, copro III | Zinc proto and low ALAD activity | — |
AIP | Hepatic/Acute | PBGD | Autosomal dominant | ALA, PBG, copro | — | Low PBGD activity | — |
HCP | Hepatic/Acute and cutaneous | CPO | Autosomal dominant | ALA, PBG, copro III | — | — | Copro III |
VP | Hepatic/Acute and cutaneous | PPO | Autosomal dominant | ALA, PBG, copro III | Fluorescence peak at 626 nm | — | Copro III and proto |
PCT | Hepatic/Cutaneous | UROD | Autosomal dominant ♦ | Uro and hepta-CP | Uro and hepta-CP | — | Isocopro |
HEP | Hepatic Δ /Cutaneous | UROD | Autosomal recessive | Uro and hepta-CP | Uro and hepta-CP | Zinc proto and low UROD activity | Isocopro |
CEP | Erythropoietic/Cutaneous | UROS | Autosomal recessive | Uro I and copro I | Uro I and copro I | Uro I and copro I | Copro I |
EPP classic | Erythro/Cutaneous | FECH | Autosomal dominant | — | Proto | Free proto | Proto |
EPP variant | Erythro/Cutaneous | ALAS-2 | X-linked recessive | — | Proto | Free and zinc proto | Proto |
* Increases of importance for diagnosis in most cases.
Δ These hepatic porphyrias also have erythropoietic features, including increases in erythrocyte zinc protoporphyrin.
♦ UROD inhibition in PCT is mostly acquired, but an inherited deficiency of the enzyme predisposes in familial (type 2) disease.
Acute intermittent porphyria (AIP) is the most common type of porphyria, with a prevalence of about 1:10,000 to 1:20,000 people. Secondary to the disease’s ability to cause neuronal damage, the incidence of AIP among patients with psychiatric disorders may be as high as 1:500. AIP may be considered the prototype for all acute porphyrias, because the presentation of all acute porphyrias is similar, with specific diagnosis requiring laboratory analysis. In AIP, patients have a deficiency of porphobilinogen (PBG) deaminase activity. Because a complete deficiency would be incompatible with life, most patients have approximately 50% of normal PBG deaminase activity. The deficiency results in an increase in cellular δ-aminolevulinic acid (ALA). Most patients are generally asymptomatic until some event stimulates the production of ALA. The deficiency of PBG deaminase activity results in relative ALA overproduction and consequent symptoms. Precipitating factors that lead to an acute exacerbation include (1) stimulation of ALA synthetase production in the liver; (2) endocrine factors, including the female reproductive cycle; (3) fasting, especially in combination with alcohol intake; (4) induction of hepatic CYP450 that leads to ALA synthetase production through a reduction in inhibitory heme; and (5) emotional stress, including surgery and chronic illness. Clinical onset occurs most often after puberty and is more common in women, likely secondary to the effects of hormones and corticosteroids on the liver.
An acute attack is normally heralded by the presence of colicky abdominal pain, nausea, and vomiting, followed by the appearance of dark urine. Patients with AIP may also complain of diarrhea or constipation. Classically, neurologic symptoms follow the onset of visceral complaints and may be highly variable. Patients may experience seizures, peripheral neuropathy, and cranial nerve deficits. They may become psychotic. Hyponatremia may be observed secondary to the syndrome of inappropriate secretion of antidiuretic hormone (SIADH).
The cornerstone of treatment in AIP, as all acute porphyrias, includes the recognition and avoidance of precipitating factors. Once precipitating factors have been eliminated, glucose therapy (400 g/day) and/or heme arginate (3 mg/kg/day for 3 days) may be instituted. Glucose and heme arginate work to decrease ALA synthetase activity and reduce the urinary excretion of ALA and shorten the length of an acute attack.
In the patient with a history of acute porphyria, optimal perioperative care includes careful planning and communication among surgeons, anesthesiologists, and internists. Presurgical admission for IV hydration with glucose-containing fluids is an important step in the patient with a history of acute attacks. A large carbohydrate load may suppress the synthesis of ALA synthetase and may be beneficial. The selection of appropriate anesthetics and analgesics is important, because many drugs frequently used in anesthesia have the capacity to induce ALA synthetase and CYP450. Table 5-6 summarizes the safety of various drugs frequently used in anesthesia. Many otherwise-asymptomatic patients with AIP (or any acute porphyria) may present for anesthesia with a misdiagnosed “surgical” abdomen. Patients should be kept warm because cold-induced stress may precipitate an acute crisis. Regional anesthesia has been used with success. Liver transplantation has been used successfully to cure AIP. Attempts to treat other porphyrias with liver transplantation have met with mixed success.
Key Points
- ▪
Porphyrias are divided into acute and cutaneous forms.
- ▪
Acute intermittent porphyria is most common (1:10,000 to 1:20,000).
- ▪
Porphyria exacerbation can be precipitated by stimulation of ALA synthetase, endocrine factors (female reproductive cycle), fasting, induction of CYP450, and emotional stress.
- ▪
Anesthetic management of porphyria patients includes avoiding precipitating drugs, early IV hydration and glucose administration, and avoidance of hypothermia.
- ▪
Acute exacerbation may be treated with glucose and heme arginate.
Generally Considered Safe | Unclear | Generally Considered Unsafe |
---|---|---|
INTRAVENOUS AGENTS | ||
Midazolam Lorazepam Propofol | Ketamine Diazepam | Barbiturates Etomidate |
INHALED AGENTS | ||
Nitrous oxide Desflurane | Isoflurane Halothane | Enflurane |
ANALGESICS | ||
Fentanyl Morphine | Alfentanil Sufentanil | |
MUSCLE RELAXANTS | ||
Succinylcholine Vecuronium | Atracurium Pancuronium | |
LOCAL ANESTHETICS | ||
Bupivacaine Procaine | Lidocaine | |
VARIOUS | ||
Atenolol Atropine Droperidol Labetalol Neostigmine | Glucocorticoids Hydralazine |
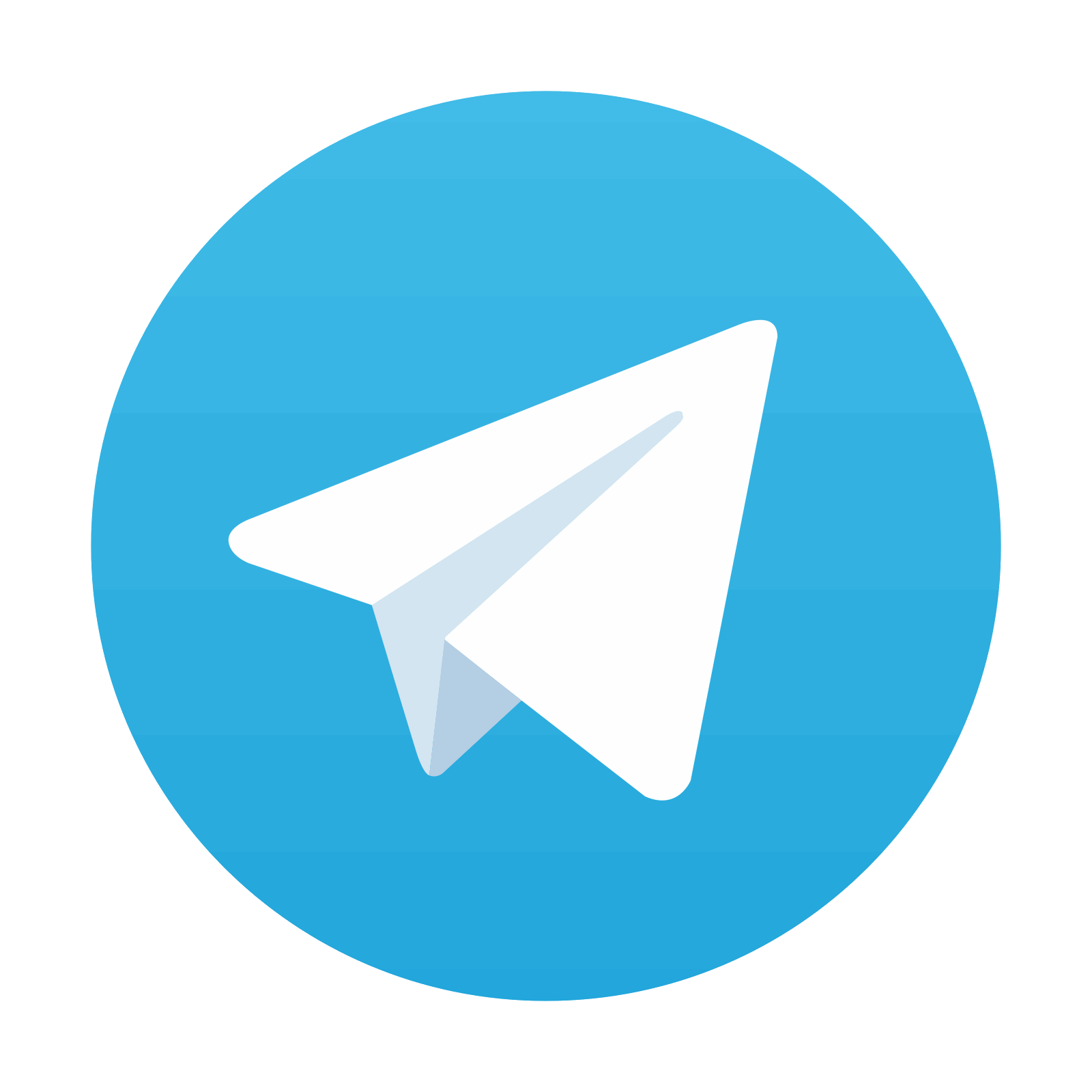
Stay updated, free articles. Join our Telegram channel

Full access? Get Clinical Tree
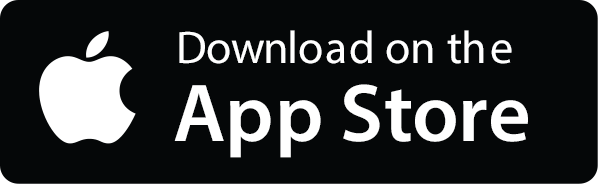
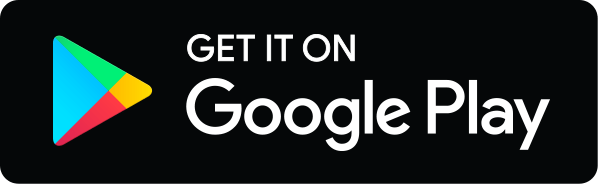
