Fig. 4.1
Focus of interest is on the voxel-based lesion—symptom mapping on the stroke patients showing the relationship between brain lesions and pantomime performance. Configural-spatial errors were linked to lesions in a network involving left inferior and temporal lobe areas (b), while body-part-as-object errors were associated with lesions to left middle and inferior gyri of the frontal lobe and the underlying white matter particularly the superior longitudinal fasciculus (c) (Reprinted with permission Manuel AL, Radman N, Mesot D, Chouiter L, Clarke S, Annoni JM, et al. Inter- and intrahemispheric dissociations in ideomotor apraxia: a large-scale lesion-symptom mapping study in subacute brain-damaged patients. Cereb Cortex. 2012 Sep 17)
Errors in the context of a movement sequence such as demonstrating how to make a cup of instant coffee may involve omission of actions in the sequence or performance of the actions in the wrong order. Individual actions in the sequence may be performed incorrectly with errors similar to those described for single gestures. In some cases these aberrations in the individual actions may arise from perseverations, that is, repetition of the preceding action or some feature of it. Errors in a sequence have been described in the Naturalistic Action Test [27].
In summary, several types of apraxia have been defined on the basis of the input modality, pantomime, imitation, or tool/object use, whether the task involves a single gesture or a sequence of gestures, and in the case of single gestures whether the gesture is meaningful (transitive versus intransitive) or meaningless, and finally whether the apraxia affects both hands or not.
Neural Correlates of Apraxia
Apraxia occurs more often in patients with left as opposed to RHD and damage to the left hemisphere also results in a more severe apraxia [10, 28]. Nevertheless apraxia has been found after stroke to the right hemisphere, suggesting that the right hemisphere may also play a role in praxis [29, 30]. This observation may reveal the basis for sparing of some praxis tasks, such as intransitive gestures and recognition of gestures in cases of LHD. Interestingly, support for right hemisphere involvement in praxis was seen in an electroencephalogram (EEG) study of patients with apraxia associated with LHD that examined activation associated with practice of a tool-use gesture. The analysis showed a greater activation of a right frontoparietal network relative to controls, suggesting that training on a tool-use gesture in the face of LHD led to recruitment of the right hemisphere [10, 31].
While much work on the neural correlates of apraxia has focused on hemispheric asymmetries, considerable research has examined for evidence of localization within the left hemisphere. A number of studies have not been able to show a reliable association between lesion locus and apraxia severity (e.g., [32, 33]). Many others though have associated IMA with damage to the parietal (left inferior parietal) and frontal (left dorsolateral frontal) cortices [23, 34] as well as the white matter tracts connecting these areas. A number of studies have linked damage in the angular and supramarginal gyri of the inferior parietal lobe to IMA [35, 36] and impairments in imitation [37]. Others have observed an association between damage in the superior parietal lobe and the ability to integrate visual and somatosensory information in gesture production [35, 38]. Lesions in the frontal and premotor areas have also been linked to apraxia [24, 35, 39]. Interestingly, it has been difficult to dissociate between lesions in the parietal and frontal lobes since lesions in these areas have been linked to similar deficits [23, 35]. Spatial errors, for example, have been associated only with more posterior lesions [23, 24, 27]. Errors in producing internal hand positions were seen with both anterior and posterior lesions, while deficits in gesture recognition and discrimination have been found with posterior but not anterior lesions [24, 40]. This connection between structure and function has largely been reported based on studies of patients with focal lesions such as those seen in stroke or in neurodegenerative conditions, such as corticobasal syndrome, which affect frontoparietal areas [41–43]. Apraxia may also be seen arising from damage in brain regions other than frontal and parietal areas such as temporal, occipital, and subcortical areas [44–46]. Anterior callosal lesions have also been associated with apraxia. In this case the apraxia would be more unilateral, affecting just the left hand and is attributed to a disconnection of the premotor and motor cortices in the right hemisphere from praxis control exerted by the left hemisphere. Damage to the basal ganglia has also been implicated in the expression of apraxia. Apraxia has been seen in various movement disorders such as corticobasal syndrome (CBS) [41–43], Parkinson’s disease [47], and Huntington’s disease [48]. The basal ganglia are thought to contribute to praxis through the control of sequencing, selection of appropriate motor programs, and performance of overlearned movements. There is some question as to whether damage isolated to the basal ganglia causes marked apraxia in that the majority of the cases involve surrounding white matter tracts and frontoparietal areas.
In 2012, Hong et al. reported a case of a 67-year-old man with a stroke affecting the left frontal anterior part of the precentral gyrus and prefrontal cortex, as well as the centrum semiovale [49]. This case is interesting because of its use of multiple brain imaging techniques to investigate the neural correlates of apraxia. In this case, structural magnetic resonance imaging (sMRI) and functional magnetic resonance imaging (fMRI) along with diffusion tensor imaging (DTI) were used. On apraxia assessment the patient was determined to have both ideomotor and limb-kinetic apraxia. The IMA was linked with damage to the superior portion of the superior longitudinal fasciculus in the left hemisphere while the limb-kinetic apraxia was linked with a thinning of the fiber tracts from the premotor and supplementary areas in the left hemisphere. Interestingly, the fibers through the corticospinal tract were unaffected, indicating that the corticospinal pathways subserving movement were intact as one might expect in cases of apraxia (see Fig. 4.2 for details of imaging).
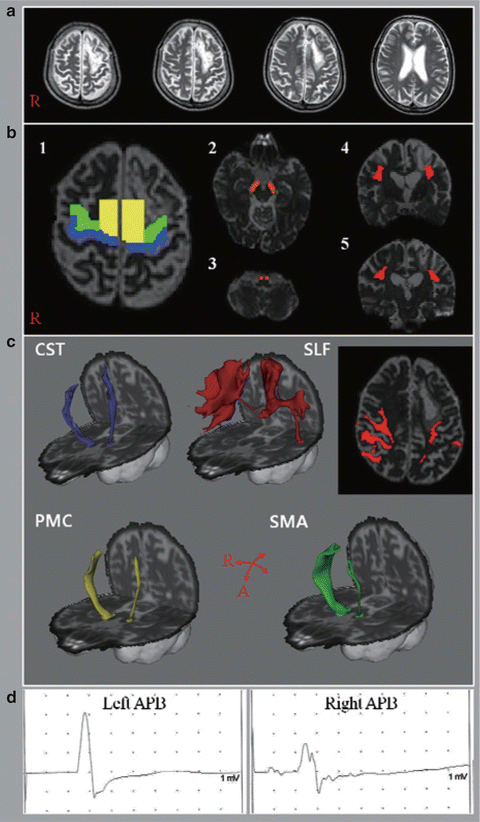
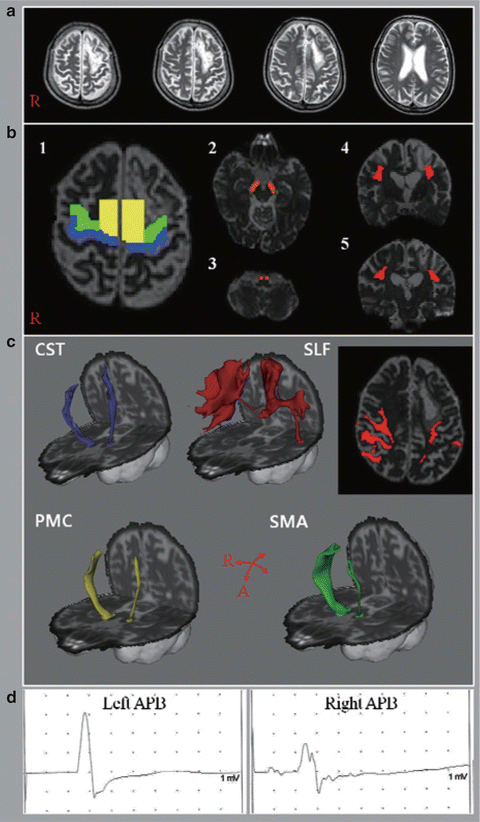
Fig. 4.2
Shows T2-weighted MR images (a) and diffusion tensor tractography (c), which shows disruption to the superior longitudinal fasciculus and fibers from the premotor cortex and the supplementary motor area but intact corticospinal tract on the affected left hemisphere. Note as well the reduced amplitude of the motor-evoked potential from the abductor pollicis brevis on the right hand (left hemisphere) (Reprinted from Hong JH, Lee J, Cho YW, Byun WM, Cho HK, Son SM, et al. Limb apraxia in a patient with cerebral infarct: Diffusion tensor tractography study. NeuroRehabilitation. 2012;30:255–259, with permission from IOS Press)
Evidence for left hemisphere dominance for praxis has also come from neuroimaging studies of healthy adults. Left hemisphere activation has been found when performing gestures with either hand [50, 51]. These neuroimaging studies have also revealed a network of brain areas subserving praxis. EEG coherence analysis revealed two networks: one involving the parietal, supplementary motor and motor areas, and the other parietal, lateral premotor and motor areas [31]. Using fMRI, Frey also identified a network associated with tool-use and communicative gestures (see Fig. 4.3) [50, 52]. Retrieving and planning transitive gestures for production using either hand was associated with increased activation in the left parietal, dorsal premotor, posterior temporal, and middle frontal areas. Interestingly, the same areas were activated when planning intransitive, communicative gestures. Frey argued that the network recruited might be task and context-dependent [53]. The parietal cortex is seen as playing an important role in selecting and producing actions based on the integration of sources of information from brain areas distributed throughout the cortex. The particular computations, and so the distributed brain areas involved, are dependent on the task demands. Pantomime of tool-use gestures involves accessing conceptual knowledge of tools in terms of their function and manipulation. The posterior temporal area appears to subserve the representation of this information. Pantomime to verbal cues may engage the dorsal premotor and middle frontal areas, while cues to pantomime involving visual tool information appear to engage the fusiform gyrus in the temporal lobe.
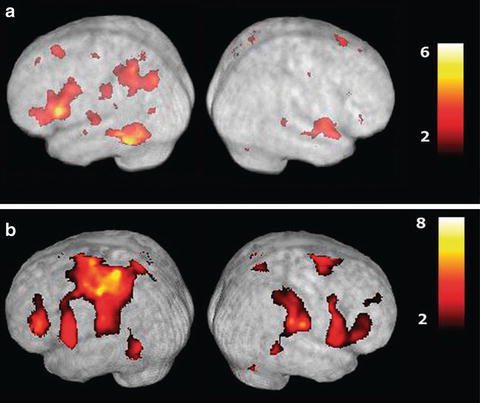
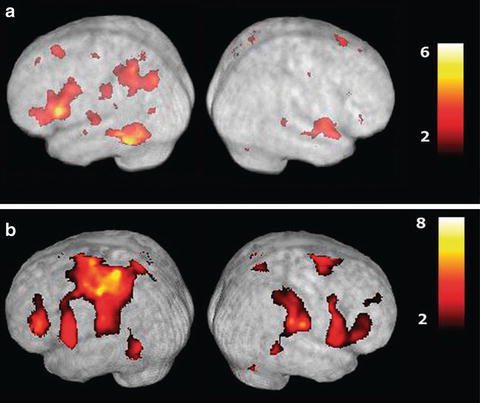
Fig. 4.3
Functional magnetic resonance imaging (fMRI) scans showing activations associated with planning and executing hand gestures. (a) Shows activation associated with planning tool-use gestures compared to random hand movements. Note major left hemisphere focused activations in the posterior parietal cortex, posterior temporal cortex, and dorsolateral prefrontal cortex. Gesture execution (b) recruits similar areas in addition to other regions associated with sensory and motor processes in both hemispheres (Reprinted with permission from Johnson-Frey SH, Newman-Norlund R, Grafton ST. A distributed left hemisphere network during planning of everyday tool use skills. Cereb Cortex. 2005;15 (6):681–695)
In contemplating knowledge of tools, Buxbaum found that patients with apraxia were impaired with regard to knowing how to manipulate tools but they were not impaired with regard to tool function [54]. Using fMRI in healthy adults, Buxbaum demonstrated activation in overlapping areas when making either manipulation or function judgments about tools [55]. These areas included regions of the left dorsolateral and ventrolateral frontal and prefrontal areas as well as posterior temporal and inferior parietal areas. There was, however, much greater activation in the left inferior parietal area bordering on intraparietal sulcus in the manipulation condition. These findings reinforce the context- and task-dependent nature of activation in tool-use gestures in the healthy brain as well as in the expression of apraxic deficits seen in neuropathologic conditions such as stroke.
Model of Apraxia
Several models have been proposed to understand the disruptions at various stages in gesture production thought to underlay apraxia [1, 56–58]. One model developed by Roy and colleagues proposes that there are three processing systems involved in praxis, i.e., the sensory-perceptual, conceptual, and production systems [42, 58–61]. The sensory-perceptual system receives and analyzes input information from the environment. This information serves as the first stage in identifying tools and actions based on their structural features. In addition, this system is involved in the analysis of visual-gestural information of a modeled gesture that is to be imitated. The conceptual system is a storage area for action-based knowledge. Within this system, three aspects of knowledge are incorporated: the knowledge of objects and tools and their functions, knowledge of actions independent of tools, and knowledge of action sequences. Selection of appropriate tools and actions in response to environmental demands is believed to be dependent on the integrity of this knowledge base.
The production system is thought to involve four stages. The first stage, response selection, involves the selection of the appropriate movement from secondary memory based on the information received from the sensory-perceptual and conceptual systems. The second (image generation) stage involves generating an image of the action to be performed, which encompasses both visual and kinesthetic information. This stage is thought to play a role in organizing movement and in controlling movement by pre-tuning the motor system to perform the selected action and to provide information on the expected visual and kinesthetic feedback. This image also incorporates information about the tool and object to enable the patient to configure the hand and fingers to accommodate to the features (e.g., size and shape) of the tool. This image is then encoded in working memory, the third stage. Working memory is critical for the maintenance of the temporarily stored movement and tool and object information. The final stage involves response organization and control.
This model is thought to encompass three routes to action: the pantomime route and two imitation routes—concurrent and delayed imitation. The pantomime route involves producing the gesture from memory and may involve two sources of input through the sensory-perceptual system: speech-language input if performing to a verbal command or visual tool information if performing in response to a picture of a tool or the actual tool. This information is fed through the conceptual system where contact is made with the components of knowledge related to the gesture and tool. This information is then fed into the production system where the appropriate response is selected and the image of the action is generated. This image is then stored in working memory and then fed into the final stage of the production system to execute the action.
Imitation of gestures is seen to involve two routes. Both depend on analysis of the visual-gestural information in the modeled gesture. In concurrent imitation the examiner models the gesture while at the same time the patient attempts to imitate it. In this condition the product of the visual-gestural analysis is fed directly to the late stage of the production system for execution of the gesture. In delayed imitation the examiner models the gesture and then stops. Once the examiner has completed the demonstration the patient attempts imitation. In this condition the product of the visual-gestural analysis is encoded into and stored in working memory. From here this information is fed into the production system for execution of the imitated gesture.
These three routes to action are seen to give rise to eight patterns of apraxia, which reflect breakdowns at various stages in gesture production (see [58] for details). Our recent work with a large group of stroke patients has provided strong support for our model using a comprehensive apraxia battery [58]. These findings have implications for the clinical assessment of apraxia as well as for examining how apraxia may affect performance of activities involved in daily living.
Implications for Clinical Assessment of Apraxia
The model-based approach to understanding apraxia has important implications for the clinical assessment of apraxia. This approach identifies disruptions at different stages of gesture production and so demands the use of a comprehensive battery, which examines both the conceptual and motor components of gesture production.
The clinical assessment for apraxia, then, should include a comprehensive battery of tests, which tap into sensory-perceptual, conceptual, and production aspects of gesture. Some of these aspects of praxis are assessed in order to rule out several exclusion criteria. Assessment of some of the sensory-perceptual and production aspects of praxis involves looking for evidence of verbal comprehension deficits and visual object agnosia. The presence of these deficits from the input side would tend to rule out a diagnosis of apraxia. By the same token from the output side one needs to look for evidence of a basic motor deficit such as a hemiparesis. In such cases the presence of a hemiparesis would not rule out apraxia per se. Rather, the expression of apraxia in the affected limb would be difficult to dissociate from the weakness and sensory loss associated with the hemiparesis, and the patient would demonstrate apraxia in the limb not affected by the hemiparesis.
The Relationship of Apraxia to Functional Performance
The model-based approach to apraxia also has implications for how apraxia is related to the performance of activities of daily living (ADL). This relationship has been addressed through observation of function in daily living as well as examining the correlation between performance on tests of apraxia and function.
From an observational perspective, the problem of apraxia is sometimes quite evident in daily living when a person presents with unusual handling of objects or with improper sequencing of task steps. For example, obvious apraxic errors are seen in a woman with ideational apraxia (IA) performing her morning routine by combing her hair with a toothbrush rather than a hairbrush or attempting to use her hairbrush in an awkward spatial position as in IMA. There are many observable conceptual, sequencing, and production errors that have been presented earlier that might possibly emerge in functional performance. What is challenging in identifying this problem through observation alone is that the presentation of apraxic errors can be quite variable [62–64]. That is, they can occur in some situations and not in others depending on the attentional state of the person, familiarity of the environment and tools, and the competing demands on the person. Also complicating the identification of apraxic errors is that people with apraxia often achieve the goal of the task, but if carefully observed they take longer and are also sidetracked by unnecessary objects in the task environment [65]. Just as observation alone is unreliable in identification of apraxia in daily function, identification of apraxia through clinical testing does not necessarily indicate that functional performance will be compromised.
The studies examining the relationship to function in ADL differ in their methods of testing apraxia and how functional dependency is measured. For example, the earliest study by Bjorneby and Reinvang [66] examined 120 stroke patients on their level of function at admission by rating functions identified in the occupational therapy notes as independent or dependent and at 6 months after discharge based on a 25-item questionnaire. IMA was assessed through pantomime to command and imitation of meaningful and nonmeaningful gestures and ideational apraxia through object use and picture sequencing. Limb-kinetic and constructional apraxias were also assessed. The relationship between ADL function and apraxia was found to be stronger in the 6-month post-discharge period than on admission. In 1988, Sundet et al. [67] repeated this study with 145 stroke patients at 6-months post discharge using a questionnaire similar to that used in the Bjorneby and Reinvang study. Age, sex, hemianopia, and hemiplegia explained 22 % of the variance in dependence at 6 months post discharge. IMA as measured by imitative finger positioning and hand movement accounted for an additional 12 % of the variance in ADL function. Nonverbal memory, constructional apraxia, aphasia, and nonverbal intelligence explained the remaining 8 % of the total variance. Similarly, in 1999, Giaquinto et al. [68] found that IMA was also part of a predictive model for the discharge Functional Independence Measure (FIM™) [69–72] of 248 subjects with stroke. IMA, measured by De Renzi’s test of gesture imitation [73], along with the cognitive and sphincter sub-items of the FIM admission scores and neglect measures of these patients, explained 72 % of the variance on the FIM™. Variables not contributing to the model included sex, lesion side, depression, comorbidities, body mass index, FIM™ motor sub-items, and aphasia [73].
Hanna-Pladdy et al. [74] found a strong relationship between apraxia severity as measured by the Florida Assessment Battery [75] and performance on the Physical Self-Maintenance Scale [76]. Apraxia severity and independence in ADL were correlated significantly (r = 0.664, p = 0.0001). Interestingly while functional performance was strongly correlated with gesture production, impairments in the conceptual system as reflected in gesture comprehension were not correlated with functional performance. This study was, however, quite small and involved only ten stroke patients and ten controls.
In one of the largest studies examining apraxia and functioning in daily living, Pedersen et al. [77] examined 618 persons with stroke to determine the relationship between the Barthel Index (BI) [78] and a test of manual apraxia. While apraxia had a weak association with the BI at discharge, a relationship was suggested that persons without manual apraxia were more likely to be discharged to an independent living environment. This study tested apraxia in a very superficial fashion—a three-item transitive pantomime test that was done either by pantomime for nonaphasic or imitation for aphasic participants. This raises the question as to how best to select measures to most clearly determine the extent of this relationship.
Indeed current literature and theory would suggest that the selection of tests to measure apraxia and functional performance needs to be done carefully. For example, mixing transitive and intransitive assessments as measures of apraxia or using them exclusively, as in the Pederson et al. [77] study, affects the ability to detect severity of apraxia, as it is generally known that intransitive tests are performed better than transitive tests [79–83]. Such a difference in severity would affect the potential for finding a relationship between apraxia and function in daily living.
This factor of severity is seen in a 2002 study by Butler who examined the performance of 17 patients with stroke on four test batteries of apraxia [64]. Three of the batteries were purported to measure IMA and the fourth ideational apraxia. Functional independence was also evaluated on the BI. The severity of apraxia varied for each patient based on which battery was the focus of the assessment. Further, while performance on these tests of apraxia was not significantly correlated with the measure of function in daily living, the BI, the correlations varied from r = 0.25 to r = 0.46 indicating that the different batteries present a very different picture of the relationship between apraxia and function in daily living.
Studies examining functional performance after intervention for apraxia have also revealed that how performance is measured is critical in examining this relationship. Indices of performance such as the BI are not well predicted by apraxia tests whereas observational measures such as the ADL Observation test do demonstrate a relation to apraxia performance [84, 85].
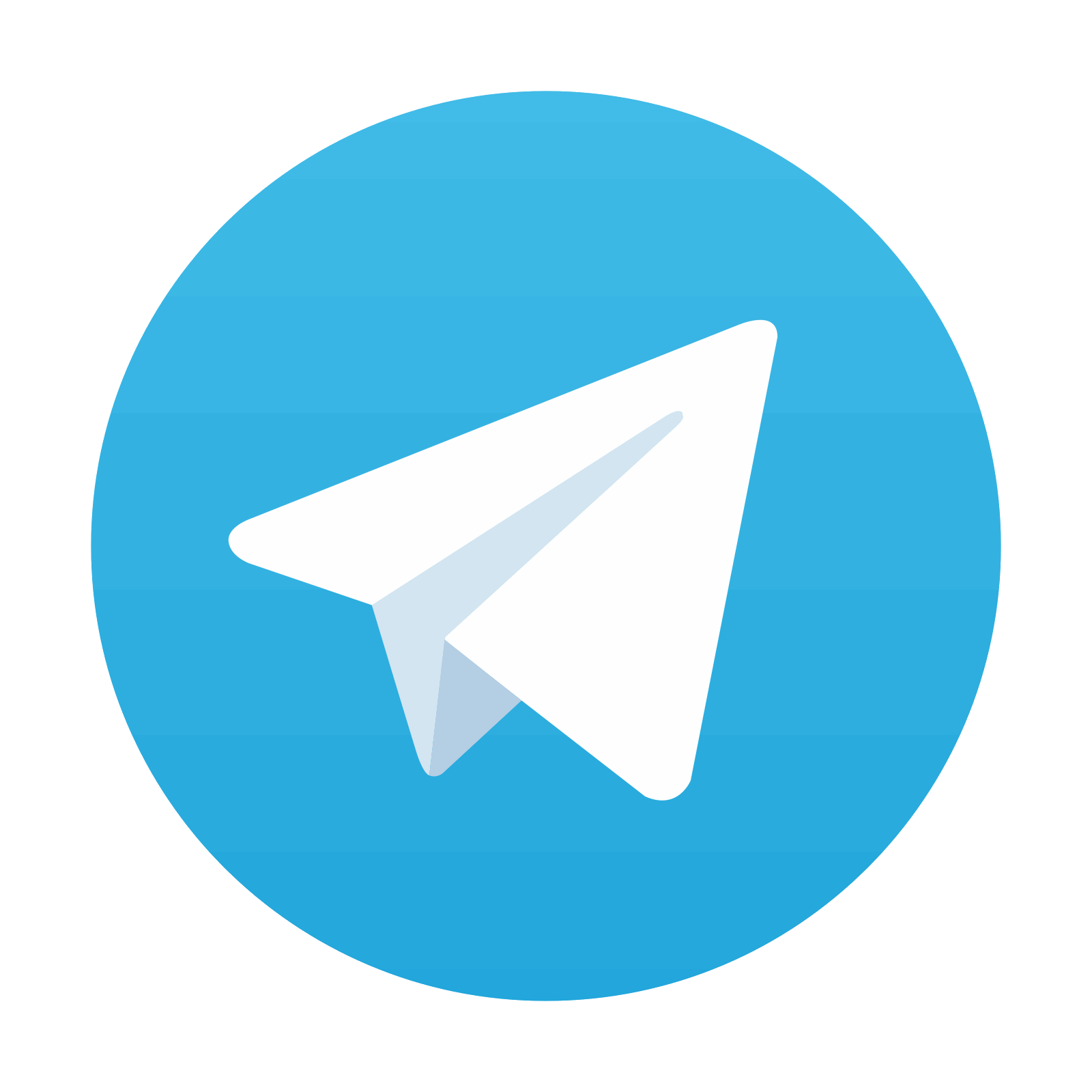
Stay updated, free articles. Join our Telegram channel

Full access? Get Clinical Tree
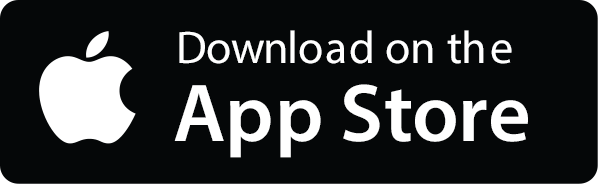
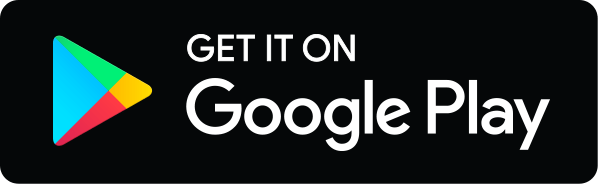