Ischemic Heart Disease and Coronary Artery Bypass Grafting
Lisa Q. Rong
Nikolaos J. Skubas
Fun-Sun F. Yao
A 57-year-old man with triple-vessel coronary artery disease (CAD) was scheduled for coronary artery bypass grafting (CABG). He had a myocardial infarction (MI) 7 months ago. He was taking nitroglycerin, digoxin, metoprolol, isosorbide dinitrate (Isordil), and nifedipine. His blood pressure (BP) was 120/80 mm Hg, and his heart rate (HR) was 60 beats per minute.
A. Medical Disease and Differential Diagnosis
What is triple-vessel CAD? Name the branches of the coronary arteries.
What are the indications for CABG?
What is percutaneous coronary intervention (PCI)? Discuss its indications, contraindications, and results.
What are the results of CABG?
B. Preoperative Evaluation and Preparation
Which preoperative tests would you order?
How would you evaluate the patient’s left ventricular function?
What are the three major determinants of myocardial oxygen consumption? How are they measured clinically?
Which factors determine myocardial oxygen supply?
Would you discontinue digoxin? Why? What is its half-life?
Would you discontinue the β-blocker (metoprolol)? Why? What is its half-life? What is the role of β-adrenergic blockers in treating congestive heart failure (CHF)?
If the patient who is on metoprolol develops hypotension intraoperatively, how would you manage it?
What is nifedipine? How does it work?
C. Intraoperative Management
C.I. Before Cardiopulmonary Bypass
How do you monitor the patient?
What is the Allen’s test?
Should you monitor temperature from multiple sites?
How do you know that the tip of the pulmonary artery (PA) catheter is in the right ventricle (RV) or the PA?
What is normal pulmonary artery occlusion pressure (PAOP)?
Is it necessary to monitor PA pressure for coronary artery operations?
What are the complications of PA catheterization?
What are the hemodynamic consequences of myocardial ischemia? How can you detect myocardial ischemia? Is PAOP a sensitive indicator of myocardial ischemia?
How would you monitor electrocardiogram (ECG)? Why V5? If you do not have precordial leads in your ECG machine, how can you monitor the left ventricle (LV)?
Discuss the principles and clinical applications of intraoperative transesophageal two-dimensional echocardiography.
How would you induce anesthesia?
How would you maintain anesthesia?
What is the better anesthetic agent for this operation—an inhalation or intravenous (IV) agent?
What are the cardiovascular effects of isoflurane, desflurane, sevoflurane, morphine, and fentanyl?
Is isoflurane dangerous for the patient with CAD?
What is the cardiovascular effect of nitrous oxide (N2O)?
What kind of neuromuscular blocker (NMB) would you use? Why?
If ST-segment depression is seen during surgery, how would you treat it? What is the relation between perioperative myocardial ischemia and postoperative MI?
Would you use prophylactic nitroglycerin during CABG to prevent intraoperative myocardial ischemia or perioperative MI?
How would you correct hypertension?
How would you treat hypotension?
What are the indications for IV metoprolol, labetalol, or esmolol during surgery? How much would you give? What are the relative contraindications?
How would you correct increased PAOP?
What should you do during sternotomy?
Would you monitor PAOP continuously? Why?
Discuss autologous transfusion and blood conservation for cardiac surgery.
C.II. During Cardiopulmonary Bypass
What anticoagulant would you give before cardiopulmonary bypass (CPB)? How much would you give? What is its mechanism?
What is the half-life of heparin? How is it eliminated?
How do you monitor heparin dosage? What is the activated coagulation time (ACT) test?
What is total CPB? What is partial bypass?
What is the purpose of venting the LV? How can it be done?
How many types of oxygenators are there? What are the advantages of each type?
What kind of priming solution would you use? How much priming solution would you use? When would you prime with blood? Why?
What are the advantages and disadvantages of hemodilution?
What kinds of pumps are used in CPB? Are they pulsatile or not?
How do you monitor the patient during CPB?
How much BP would you keep during CPB? Why?
How would you treat hypotension during CPB?
How would you treat hypertension (a mean arterial pressure [MAP] of over 100 mm Hg)?
How do you prepare an IV infusion of sodium nitroprusside, nicardipine, and nitroglycerin? What are the usual doses? Which do you prefer to use?
How much pump flow would you maintain during CPB?
How would you adjust the pump flow during hypothermia?
How would you adjust the pump flow during hemodilution?
What are the advantages of hypothermia? Does hypothermia offer neuroprotection?
How does blood viscosity change during hypothermia and hemodilution?
What are the main causes of death associated with accidental hypothermia?
Would you give anesthesia during CPB? Why?
Would you give muscle relaxants during CPB? How is the action of muscle relaxant affected during CPB?
How do you know the patient is well perfused during CPB?
How much gas flow would you use for the oxygenator? What kind of gas would you use? Why?
What are the disadvantages of hypocapnia during CPB?
The arterial blood gases and electrolytes during CPB are as follows: pH, 7.36; PaCO2, 42 mm Hg; PaO2, 449 mm Hg; CO2 content, 24 mEq per L; sodium (Na), 128 mEq per L; potassium (K), 5.8 mEq per L; and hematocrit (Hct), 20%. The patient’s temperature is 27°C (80.6°F). At what temperature are blood gases measured? How would you correct the blood gases according to patient’s body temperature? Would you treat the arterial blood gases at 37°C (98.6°F) or at patient’s body temperature?
If the blood level of the venous reservoir is low, what would you replace it with? Blood or balanced salt solution?
How do you estimate the fluid balance during CPB?
How would you preserve the myocardium during CPB?
What is the cardioplegic solution? How much would you use?
For how long a period can the aorta be cross-clamped?
Why would urine become pink in some cases of CPB? What is the renal threshold for plasma hemoglobin?
At what temperature can the patient be weaned from CPB?
Why does it take longer to rewarm than to cool the patient by the pump oxygenator?
How would you defibrillate the heart internally during CPB?
Why is calcium chloride usually administered right before the patient comes off the pump?
If the HR is 40 beats per minute, what should you do?
How does the blood sugar level change during CPB? Why? What is optimal glucose control intraoperatively? Does hyperglycemia increase neurologic complications during CPB?
What are the effects of CPB on platelet and coagulation factors?
How would you prepare for termination of CPB?
How would you decide the need for inotropic support?
C.III. After Cardiopulmonary Bypass
How would you reverse heparin? How much protamine would you use? What are the other drugs used to neutralize heparin?
What is the action mechanism of protamine?
What are the complications of too much protamine?
Why did the patient develop hypotension after protamine was administered? How do you treat and prevent this condition?
What are the indications for intra-aortic balloon pump (IABP)?
What are the principles of IABP?
What are the complications of IABP?
Can PAOP represent left ventricular end-diastolic volume (LVEDV) after CABG?
D. Postoperative Management
What are the postoperative complications?
Would you reverse the muscle relaxants? Why?
When will you wean the patient from the respirator?
What criteria would you use in deciding when to wean the patient from the respirator?
A. Medical Disease and Differential Diagnosis
A.1. What is triple-vessel CAD? Name the branches of the coronary arteries.
Triple-vessel CAD involves progressive atherosclerosis of the major branches of the coronary arteries with eventual luminal obstruction and myocardial injury. The intimal disease is typically segmental but can be diffuse in severe cases. The coronary arteries are the following:
The right coronary artery (RCA)
The left anterior descending (LAD) branch of the left main coronary artery (LMCA)
The left circumflex (CX) branch of the LMCA
The branches of coronary arteries are shown in Figure 5.1. The LAD and CX arise from the LMCA. The sinus node is supplied by the RCA in approximately 50% to 60% of people and by the CX artery in the remaining 40% to 50%. The atrioventricular (AV) node is supplied
by the RCA in 85% to 90% of people and by the left CX artery in the remaining 10% to 15%; therefore, the RCA is dominant in 85% to 90% of patients. The most common “targets” for coronary bypass grafting are LAD, obtuse marginal (branch of CX), and posterior descending (branch of RCA) arteries.
by the RCA in 85% to 90% of people and by the left CX artery in the remaining 10% to 15%; therefore, the RCA is dominant in 85% to 90% of patients. The most common “targets” for coronary bypass grafting are LAD, obtuse marginal (branch of CX), and posterior descending (branch of RCA) arteries.
Bonow RO, Mann DL, Zipes DP, et al, eds. Braunwald’s Heart Disease: A Textbook of Cardiovascular Medicine. 9th ed. Philadelphia, PA: Saunders Elsevier; 2012:465.
A.2. What are the indications for CABG?
Patients whose angina is not controlled by medical treatment or who have unacceptable side effects with such management should be considered for coronary revascularization. The indications for CABG are as follows:
Acute myocardial infarction if a nonsurgical intervention failed or there is ischemia at rest or hemodynamic instability/cardiogenic shock or complications, such as ventricular septal defect or ruptured papillary muscle and mitral regurgitation
Life-threatening ventricular arrhythmia in the presence of significant left main (>50%) or coronary artery (>70%) obstruction
At the same time with other cardiac surgical procedures
Bonow RO, Mann DL, Zipes DP, et al, eds. Braunwald’s Heart Disease: A Textbook of Cardiovascular Medicine. 9th ed. Philadelphia, PA: Saunders Elsevier; 2012:1353.
Hillis LD, Smith PK, Anderson JL, et al. 2011 ACCF/AHA guideline for coronary artery bypass graft surgery: a report of the American College of Cardiology Foundation/American Heart Association Task Force on Practice Guidelines. Circulation. 2011;124:e652-e735.
A.3. What is percutaneous coronary intervention (PCI)? Discuss its indications, contraindications, and results.
PCI has developed rapidly since its introduction in 1977. It is now an acceptable method of treating selected patients who have angina pectoris. This technique involves the passage of a small (3 French) catheter into the involved coronary artery and through the stenosis. With the balloon portion of the catheter straddling the stenosis, inflations are performed that result in enlargement of the stenotic lumen and a controlled injury involving, to a varying degree, plaque compression, intimal fissures, and medial stretching. Following the dilation, coronary stents are introduced to maintain long-term patency. The stents are either baremetal (cobalt-chromium alloy) or drug-eluting stents (DES).
PCI has redefined who is candidate for elective surgical myocardial revascularization. With the current available technology, PCI is considered a therapeutic option in any individual with disabling ischemic symptoms despite good medical therapy and focal obstructive coronary disease regardless of cause. The indications for PCI are as follows:
Isolated discrete proximal single- or double-vessel disease
Post-CABG with new stenotic lesions or stenosis at distal anastomosis
Restenosis following PCI
Contraindications to CABG
Coronary stenosis following cardiac transplantation
Occluded vessels within the last 6 months and less than 15 mm in length
Post-streptokinase therapy for revascularization
PCI is contraindicated in the following:
Left main CAD in which the distal coronary vessels are not protected by at least one completely patent large collateral vessel
Multivessel severe diffuse disease
Absence of a discrete obstructing lesion
The primary success rate of PCI is approximately 90%, but restenosis occurs at a rate of approximately 30%, 6 months postprocedure. Dilatation can be repeated with a 90% success rate. The artery tends to remain patent after the second angioplasty. With the introduction of
coronary stents, which act as a metal scaffold, the restenosis rate after PCI has been decreasing, and fewer patients require subsequent revascularization: 10% to 15% after stenting versus 25% to 35% after PCI alone. The decrease in luminal diameter following stent placement is evident within the first 6 to 9 months after stent placement and is the result of proliferative neointimal tissue growth in response to the associated injury and inflammation. The stent scaffold is coupled with polymers that elute or slowly release pharmacologic agents (sirolimus, an immunosuppressant, and paclitaxel, an antineoplastic) which inhibit the neointimal growth. The DES have reduced the need for repeat revascularization to an even lower rate, 4% to 6%.
coronary stents, which act as a metal scaffold, the restenosis rate after PCI has been decreasing, and fewer patients require subsequent revascularization: 10% to 15% after stenting versus 25% to 35% after PCI alone. The decrease in luminal diameter following stent placement is evident within the first 6 to 9 months after stent placement and is the result of proliferative neointimal tissue growth in response to the associated injury and inflammation. The stent scaffold is coupled with polymers that elute or slowly release pharmacologic agents (sirolimus, an immunosuppressant, and paclitaxel, an antineoplastic) which inhibit the neointimal growth. The DES have reduced the need for repeat revascularization to an even lower rate, 4% to 6%.
However, the PCI is a different therapeutic approach than CABG. PCI is targeting the area of lesion only, whereas CABG bypasses these lesion areas. This may be the reason that, at least in the intermediate term, CABG is considered to be superior for patients with multivessel disease.
Diegeler et al. showed that, for isolated high-grade lesions of the LAD, CABG through a left anterior thoracotomy is as effective as PCI with stent placement. Although stenting provided excellent short-term results with fewer periprocedural adverse events, CABG was superior with regard to the need for repeated intervention in the target vessel and freedom from angina at 6 months of follow-up. Further, Serruys et al. compared outcomes between PCI with stent and CABG surgery and found that at 5 years, there was no difference in mortality between stenting and surgery for multivessel disease. Importantly, the incidence of stroke or MI was not significantly different between the two groups. However, the rate of major adverse cardiac and cerebrovascular events was higher in the stent group, driven by the increased need for repeat revascularization. A recent report on 10-year outcomes also showed that for the treatment of unprotected LMCA disease, PCI with stent implantation showed similar long-term mortality and rates of death, Q-wave MI, or stroke. However, stenting, even with DES, was associated with higher rates of repeat revascularization than was CABG. Lastly, a recent comparison between stenting and CABG in patients with previously untreated three-vessel disease or LMCA disease concluded that CABG remains the standard of care for patients with three-vessel or LMCA disease because the use of CABG, as compared with PCI, resulted in lower rates of the combined end point of major adverse cardiac or cerebrovascular events at 1 year.
Bonow RO, Mann DL, Zipes DP, et al, eds. Braunwald’s Heart Disease: A Textbook of Cardiovascular Medicine. 9th ed. Philadelphia, PA: Saunders Elsevier; 2012:1301.
Diegeler A, Thiele H, Falk V, et al. Comparison of stenting with minimally invasive bypass surgery for stenosis of the left anterior descending coronary artery. N Engl J Med. 2002;347:561-566.
Hillis LD, Smith PK, Anderson JL, et al. 2011 ACCF/AHA guideline for coronary artery bypass graft surgery: a report of the American College of Cardiology Foundation/American Heart Association Task Force on Practice Guidelines. Circulation. 2011;124:e652-e735.
Moliterno DJ. Healing Achilles—sirolimus versus paclitaxel. N Engl J Med. 2005;353:724-727.
Park DW, Kim YH, Yun SC, et al. Long-term outcomes after stenting versus coronary artery bypass grafting for unprotected LMCA disease: 10-year results of bare-metal stents and 5-year results of drug-eluting stents from the ASAN-MAIN (ASAN Medical Center-Left MAIN Revascularization) Registry. J Am Coll Cardiol. 2010;56(17):1366-1375.
Serruys PW, Morice MC, Kappetein AP, et al. Percutaneous coronary intervention versus coronary-artery bypass grafting for severe coronary artery disease. N Engl J Med. 2009;360:961-972.
Serruys PW, Ong AT, van Herwerden LA, et al. Five-year outcomes after coronary stenting versus bypass surgery for the treatment of multivessel disease. J Am Coll Cardiol. 2005;46:575-581.
A.4. What are the results of CABG?
Kuan et al. reported a perioperative MI rate of 4% to 6%. The overall operative mortality rate of CABG at major medical centers is approximately 1%. Reoperation is associated with a higher operative mortality, approximately 2% to 3%. Rahimtoola et al. studied the status of patients who underwent CABG for unstable angina over a 10-year period. The 1-month mortality rate was 1.8%. The 5-year survival rate was 92%, and the 10-year survival rate was 83%. CABG was repeated at a rate of 1% to 2% per year; 81% of patients were anginafree or had only mild angina. Cameron et al. found observationally that the 16-year survival rate among the group receiving the internal mammary artery (IMA) graft, as compared with
the group receiving the vein grafts conveyed a greater survival benefit over time. Goldman et al. described a 10-year serial follow-up of patients operated on in the 1980s comparing saphenous vein grafts (SVG) to IMA grafts. They found that the 10-year patency rate for SVG was 61% versus 85% in IMA grafts. Overtime, SVG patency decreased from 95% at 1 week to 61% at 10 years versus 99% to 85% for IMA grafts.
the group receiving the vein grafts conveyed a greater survival benefit over time. Goldman et al. described a 10-year serial follow-up of patients operated on in the 1980s comparing saphenous vein grafts (SVG) to IMA grafts. They found that the 10-year patency rate for SVG was 61% versus 85% in IMA grafts. Overtime, SVG patency decreased from 95% at 1 week to 61% at 10 years versus 99% to 85% for IMA grafts.
A systematic overview of the seven randomized trials that compared coronary bypass surgery with medical therapy between 1972 and 1984 yielded 2,649 patients. Patients undergoing CABG had a significantly lower mortality at 5, 7, and 10 years, but by 10 years, 41% of the patients initially randomized to medical therapy had undergone CABG. Therefore, coronary bypass surgery prolongs survival in patients with significant left main CAD (irrespective of symptoms) and in patients with three-vessel disease that includes the proximal LAD coronary artery (irrespective of left ventricular function). Surgical therapy also prolongs life expectancy in patients with two-vessel disease and left ventricular dysfunction, particularly in those with a critical stenosis of the proximal LAD coronary artery. Although no study has documented a survival benefit with surgical treatment in patients with single-vessel disease, there is evidence of poor long-term survival rate with medical therapy alone in patients with impaired left ventricular function.
Hannan et al. studied prospectively 37,212 patients who underwent CABG and 22,102 patients who underwent stenting; CABG was associated with a significantly higher likelihood of survival (hazard ratios for death 0.75 for two-vessel CAD and 0.64 for three-vessel CAD, compared with stenting). The CABG patients had significantly lower ejection fractions, were more likely to have had an MI in the week before the procedure, had a significantly higher prevalence of comorbidities, and were significantly more likely to have three-vessel disease.
Magovern et al. reported that use of perioperative statin therapy significantly reduces mortality in high-risk patients undergoing CABG surgery.
Bonow RO, Mann DL, Zipes DP, et al, eds. Braunwald’s Heart Disease: A Textbook of Cardiovascular Medicine. 9th ed. Philadelphia, PA: Saunders Elsevier; 2012:1301.
Cameron A, Davis KB, Green G, et al. Coronary bypass surgery with internal-thoracic-artery grafts—effects on survival over a 15-year period. N Engl J Med. 1996;334:216-219.
Collard CD, Body SC, Shernan SK, et al. Preoperative statin therapy is associated with reduced cardiac mortality after coronary artery bypass graft surgery. J Thorac Cardiovasc Surg. 2006;132(2):392-400.
Goldman S, Zadina K, Moritz T, et al. Long-term patency of saphenous vein and left internal mammary artery grafts after coronary artery bypass surgery: results from a Department of Veterans Affairs Cooperative Study. J Am Coll Cardiol. 2004;44(11):2149-2156.
Haase J, Jung T, Störger H, et al. Long-term outcome after implantation of bare metal stents for the treatment of coronary artery disease: rationale for the clinical use of antiproliferative stent coatings. J Interv Cardiol. 2003;16(6):469-473.
Hannan EL, Racz MJ, Walford G, et al. Long-term outcomes of coronary-artery bypass grafting versus stent implantation. N Engl J Med. 2005;352:2174-2183.
Kandzari DE, Leon MB, Popma JJ, et al. Comparison of zotarolimus-eluting and sirolimus-eluting stents in patients with native coronary artery disease: a randomized controlled trial. J Am Coll Cardiol. 2006;48(12):2440-2447. Kuan P, Bernstein SB, Ellestad MH. Coronary artery bypass surgery morbidity. J Am Coll Cardiol. 1984;3:1391.
Magovern JA, Moraca RJ, Bailey SH, et al. Preoperative statin is associated with decreased operative mortality in high risk coronary artery bypass patients. J Cardiothorac Surg. 2010;5:8.
Rahimtoola SH, Nunley D, Grunkemeier G, et al. Ten-year survival after coronary bypass surgery for unstable angina. N Engl J Med. 1983;308:676-681.
B. Preoperative Evaluation and Preparation
B.1. Which preoperative tests would you order?
In addition to the routine systemic examinations of all organ systems, special attention should be paid to the cardiovascular status.
Renal function—urinalysis, blood urea nitrogen, creatinine
The routine evaluation of the hepatic function is not any more the standard, unless specifically indicated, that is, previous history of hepatitis or jaundice or the patient is drug abuser.
Pulmonary function—chest x-ray film (absolutely indicated in reoperated sternotomies to investigate the proximity of the sternum to the anterior surface of the heart), baseline arterial blood gases, and spirometry if indicated
Hematologic function—complete blood count, prothrombin time, partial thromboplastin time, platelets (platelet function if available and/or indicated; namely, for those patients previously receiving antiplatelet therapy)
Metabolism—electrolytes and blood sugar
Cardiovascular function—resting and exercise ECG (if a stress test was performed preoperatively), cardiac catheterization and coronary angiography, location and severity of coronary occlusion, echocardiography, and left ventricular function (from catheterization, magnetic resonance imaging, enhanced multidetector CT, and/or echocardiography)
B.2. How would you evaluate the patient’s left ventricular function?
Medical history: history of MI and presence or absence of angina
Symptoms and clinical signs of left ventricular failure (dyspnea at rest or exercise, nocturnal orthopnea) and/or right heart failure (ascites, pitting edema, jugular vein distention)
Cardiac catheterization, angiography, and echocardiography
Ejection fraction (normally ˜65%)
LVEDP or PAOP: normal 6 to 15 mm Hg
Left ventricular wall motion (by echocardiography)—normal (wall thickening of >30%), hypokinesis (wall thickening of 10% to 30%), akinesia (wall thickening of <10%), or dyskinesia (outward motion during systole) (Fig. 5.2)
Cardiac index (normal >2.2 L/min/m2)
Myocardial viability studies (contrast echocardiography or positron emission tomography study)
Abnormal regional wall motion (RWM) is the result of CAD; the interruption of perfusion results in abnormal motion in the related myocardial segments. There is a gradation of wall motion abnormality that consists progressively of hypokinesis, akinesis, and, subsequently, dyskinesis in which a wall moves away from the center of the ventricle. Wall thickening and endocardial motion are intrinsically tied, and all regional wall motion abnormalities (RWMA) are expected initially to be associated with abnormalities of thickening as well as endocardial motion (see also Chapter 7, section A.3).
Bonow RO, Mann DL, Zipes DP, et al, eds. Braunwald’s Heart Disease: A Textbook of Cardiovascular Medicine. 9th ed. Philadelphia, PA: Saunders Elsevier; 2012:245.
Pagel PS. Cardiac physiology. In: Kaplan JA, Reich DL, Savino JS, eds. Kaplan’s Cardiac Anesthesia: The Echo Era. 6th ed. Philadelphia, PA: Elsevier Saunders; 2011:98-131.
Schuleri KH, Centola M, George RT, et al. Characterization of peri-infarct zone heterogeneity by contrastenhanced multidetector computed tomography: a comparison with magnetic resonance imaging. J Am Coll Cardiol. 2009;53(18):1699-1707.
B.3. What are the three major determinants of myocardial oxygen consumption? How are they measured clinically?
The three major determinants of myocardial oxygen consumption are myocardial wall tension, contractility, and HR.
Myocardial wall tension is estimated by the following:
The Laplace equation states that the myocardial wall tension (T) is analogous to the diameter of the ventricle (R, radius) and the intracavitary pressure (P) and inversely proportional to the myocardial wall thickness (Th): T = P × R ÷ 2Th
Diastolic wall tension is related to the diastolic radius (R) of the ventricle, which is considered a surrogate of preload or LVEDV; the latter is assessed by the PAOP (because neither LVEDP or left atrial pressure can be directly measured).
Afterload is approximated via the systolic BP, which equals systolic ventricular pressure if there is no aortic stenosis.
Contractility is evaluated by:
Invasive techniques. Maximal velocity of contraction (Vmax), dP/dt (pressure time indices of ventricle: how fast [dt] the intraventricular pressure [dP] develops), or left ventricular end-systolic pressure/volume ratio
Noninvasive technique. Pre-ejection period/left ventricular ejection time, and global and regional ventricular wall motion by echocardiography (Fig. 5.3)
Bonow RO, Mann DL, Zipes DP, et al, eds. Braunwald’s Heart Disease: A Textbook of Cardiovascular Medicine. 9th ed. Philadelphia, PA: Saunders Elsevier; 2012:1278.
O’Brien ERM, Nathan HJ. Coronary physiology and atherosclerosis. In: Kaplan JA, Reich DL, Savino JS, eds. Kaplan’s Cardiac Anesthesia: The Echo Era. 6th ed. Philadelphia, PA: Elsevier Saunders; 2011:132-156.
B.4. Which factors determine myocardial oxygen supply?
Myocardial oxygen (O2) supply = coronary blood flow × arterial O2 content
The coronary blood flow depends on the following:
Diastolic aortic pressure (DAP)
LVEDP
Patency of coronary arteries
Coronary vascular tone
And it can be determined by the formula:
Coronary blood flow = coronary perfusion pressure ÷ myocardial vascular resistance, or, Coronary blood flow = (DAP – LVEDP) ÷ myocardial vascular resistance
The arterial O2 content (CaO2) is determined by the following equation:
CaO2 = 1.34 (mL/g) × hemoglobin concentration (g/dL) × O2 saturation (%) + (0.0031 × PaO2)
O’Brien ERM, Nathan HJ. Coronary physiology and atherosclerosis. In: Kaplan JA, Reich DL, Savino JS, eds. Kaplan’s Cardiac Anesthesia: The Echo Era. 6th ed. Philadelphia, PA: Elsevier Saunders; 2011:132-156.
B.5. Would you discontinue digoxin? Why? What is its half-life?
In order to prevent digitalis intoxication after CPB, digitalis preparations are usually discontinued one half-life (1.5 to 1.7 days for digoxin, 5 to 7 days for digitoxin) before surgery. Digitalis intoxication is quite possible, especially after CPB when acid-base and electrolytes are abnormal. If a digitalis-dependent patient is in CHF, digitalis is continued until the night before surgery. However, the predisposing factors to digitalis intoxication, especially hypopotassemia and hypercalcemia, have to be prevented.
Royster RL, Butterworth J, Groban L, et al. Cardiovascular pharmacology. In: Kaplan JA, Reich DL, Savino JS, eds. Kaplan’s Cardiac Anesthesia: The Echo Era. 6th ed. Philadelphia, PA: Elsevier Saunders; 2011:239-297.
B.6. Would you discontinue the β-blocker (metoprolol)? Why? What is its half-life? What is the role of β-adrenergic blockers in treating congestive heart failure (CHF)?
The β-blocker (metoprolol) should be continued not only up until surgery but also throughout the perioperative period. In patients with unstable angina, sudden withdrawal of β-blocker may produce an exacerbation of symptoms and may precipitate acute MI. The dose of β-blocker does not need to be reduced before surgery for fear of bradycardia, hypotension, or difficulty in weaning from CPB. The half-life of oral propranolol is 3.4 to 6 hours and of metoprolol 3 hours. Both propranolol and metoprolol are metabolized in the liver.
Propranolol disappears from the plasma and atria within 24 to 48 hours after discontinuing doses of 30 to 240 mg per day. It has been shown that with a 0.5-mg dose of propranolol IV, blood levels as high as 50 ng per mL are obtained but rapidly drop off to immeasurable levels within 5 to 10 minutes. No myocardial depression has been seen with these small IV doses. Current advanced cardiac life support guidelines suggest a 5-mg bolus of metoprolol at 5-minute intervals, and a 0.1 mg per kg bolus dose of propranolol divided into three equal doses at 2- to 3-minute intervals.
Propranolol disappears from the plasma and atria within 24 to 48 hours after discontinuing doses of 30 to 240 mg per day. It has been shown that with a 0.5-mg dose of propranolol IV, blood levels as high as 50 ng per mL are obtained but rapidly drop off to immeasurable levels within 5 to 10 minutes. No myocardial depression has been seen with these small IV doses. Current advanced cardiac life support guidelines suggest a 5-mg bolus of metoprolol at 5-minute intervals, and a 0.1 mg per kg bolus dose of propranolol divided into three equal doses at 2- to 3-minute intervals.
Reductions in HR with a β-blocker (propranolol or metoprolol) occur at lower serum levels than depression of myocardial contractility. Accordingly, as drug levels decrease after discontinuation of therapy, reductions in chronotropic response last longer than reductions in inotropy. This is an important concept in treating tachycardias in patients with significant ventricular dysfunction and CHF: a small dose is appropriate and indicated, whereas a large dose may suppress even further the inotropic state.
Numerous studies have confirmed improvements in cardiac function, exercise capacity, and long-term survival in patients with heart failure resulting from MI, hypertrophic cardiomyopathy, or idiopathic dilated cardiomyopathy with β-antagonists. β-Antagonists may also be of benefit in patients with diastolic dysfunction, secondary to hypertension.
Potential benefits of β-adrenergic blockade in heart failure include decreased HR and normalization of β-receptor function. Slower HRs improve diastolic function by increasing the diastolic filling time and myocardial perfusion and by decreasing the myocardial oxygen consumption. β-Adrenergic receptors are downregulated in heart failure, but their response is normalized by long-term β-blockade. Partial β-agonists may provide baseline sympathetic drive but act as antagonists against excessive sympathetic stimulation.
Hillis LD, Smith PK, Anderson JL, et al. 2011 ACCF/AHA guideline for coronary artery bypass graft surgery: a report of the American College of Cardiology Foundation/American Heart Association Task Force on Practice Guidelines. Circulation. 2011;124:e652-e735.
Royster RL, Butterworth J, Groban L, et al. Cardiovascular pharmacology. In: Kaplan JA, Reich DL, Savino JS, eds. Kaplan’s Cardiac Anesthesia: The Echo Era. 6th ed. Philadelphia, PA: Elsevier Saunders; 2011:239-297.
B.7. If the patient who is on metoprolol develops hypotension intraoperatively, how would you manage it?
The more common causes of intraoperative hypotension, such as hypovolemia, deep anesthesia, and surgical manipulation, should be corrected first. There are no specific antagonists for metoprolol. In rare instances, it is necessary to administer atropine for bradycardia or epinephrine, isoproterenol, glucagon, calcium, or digitalis to counteract the negative inotropic state associated with β-blockade. Cardiogenic hypotension is usually associated with high PAOP and low BP.
Royster RL, Butterworth J, Groban L, et al. Cardiovascular pharmacology. In: Kaplan JA, Reich DL, Savino JS, eds. Kaplan’s Cardiac Anesthesia: The Echo Era. 6th ed. Philadelphia, PA: Elsevier Saunders; 2011:239-297.
B.8. What is nifedipine? How does it work?
Nifedipine is a calcium channel blocker. At present, verapamil (a phenylalkylamine); diltiazem (a benzothiazepine); nicardipine, nifedipine, isradipine, amlodipine, felodipine, nimodipine, and clevidipine (dihydropyridines); and bepridil (a diarylaminopropylamine ether) are approved for clinical use in the United States. Calcium channel blockers inhibit excitation-contraction coupling of myocardial and smooth muscle by blocking the calcium influx at cellular membranes. This results in decreased myocardial contractility and vasodilation, respectively, and as a consequence, decreased myocardial oxygen consumption. Calcium channel blockers are effective for the treatment of variant angina (Prinzmetal angina), angina pectoris, and possibly acute MI.
Calcium also plays a key role in cardiac electrical activity. The electrical activity of the sinoatrial (SA) and AV nodal cells are especially dependent on the calcium or “slow” current, whereas the remaining of the specialized conduction system is more dependent on the sodium or “fast” current. Verapamil has a more profound influence on the calcium current of the SA and AV nodes. This drug has been most useful in the treatment of supraventricular tachyarrhythmias, which are often caused by reentry through the AV node. In contrast,
nifedipine and nicardipine have less influence on the SA node and no effect on AV conduction time. Therefore, nifedipine and nicardipine might be used when further suppression of AV conduction is undesirable. The relative cardiovascular effects of calcium channel blockers are shown in Table 5.1. Verapamil was found to profoundly depress the cardiovascular system during high concentrations of halothane or isoflurane anesthesia. However, because of a tendency for increased incidence of sinus arrest and bradycardia and more hemodynamic depression during enflurane anesthesia, Rogers et al. concluded that IV verapamil is better tolerated during low-dose isoflurane and halothane anesthesia.
nifedipine and nicardipine have less influence on the SA node and no effect on AV conduction time. Therefore, nifedipine and nicardipine might be used when further suppression of AV conduction is undesirable. The relative cardiovascular effects of calcium channel blockers are shown in Table 5.1. Verapamil was found to profoundly depress the cardiovascular system during high concentrations of halothane or isoflurane anesthesia. However, because of a tendency for increased incidence of sinus arrest and bradycardia and more hemodynamic depression during enflurane anesthesia, Rogers et al. concluded that IV verapamil is better tolerated during low-dose isoflurane and halothane anesthesia.
TABLE 5.1 Relative Cardiovascular Effects of Oral Calcium Channel Blockers | ||||||||||||||||||||||||
---|---|---|---|---|---|---|---|---|---|---|---|---|---|---|---|---|---|---|---|---|---|---|---|---|
|
Curran MP, Robinson DM, Keating GM. Intravenous nicardipine: its use in the short-term treatment of hypertension and various other indications. Drugs. 2006;66(13):1755-1782.
Gradman AH, Vivas Y. New drugs for hypertension: what do they offer? Curr Hypertens Rep. 2006;8(5):425-432.
Kaplan JA, Reich DL, Savino JS, eds. Kaplan’s Cardiac Anesthesia: The Echo Era. 6th ed. Philadelphia, PA: Elsevier Saunders; 2011:239-297.
Rogers K, Hysing ES, Merin RG, et al. Cardiovascular effects of/and interaction between calcium-blocking drugs and anesthetics in chronically instrumented dogs, II: verapamil, enflurane and isoflurane. Anesthesiology. 1986;64:568-575.
C. Intraoperative Management
C.I. Before Cardiopulmonary Bypass
C.I-1. How do you monitor the patient?
ECG—simultaneous leads V5 and II, multiple-lead ST-segment analysis
Arterial line for BP and arterial blood gases
PA catheter—pulmonary and central venous pressures (CVP), hemodynamic parameters (cardiac output, stroke volume, systemic and pulmonary vascular resistance)
CVP line only—if the patient has good left ventricular function and no problems are expected
Urine output
Temperature—esophageal (or PA) and bladder (or rectal, tympanic, or nasopharyngeal)
Laboratory—arterial blood gases, electrolytes, Hct, ACT, and oxygen saturation of the mixed venous blood (SVO2)
Airway pressures and respiratory gases analyzer
End-tidal carbon dioxide (CO2) analyzer
Pulse oximeter for plethysmography (adequacy of peripheral pulse, pulse rate) and arterial oxygenation
Transesophageal echocardiography (TEE) if not contraindicated. Absolute contraindications are esophagectomy or esophagogastrectomy, active upper gastroesophageal bleeding, or recent surgery, oropharyngeal trauma, and presence of esophageal pathology (tracheoesophageal fistula, stricture, trauma, tumor, scleroderma, Mallory-Weiss tear, and diverticulum). However, the anticipated benefits should be weighed against potential risks in every patient, prior to deciding whether to insert a TEE probe.
Cerebral oximeter especially for patients with high risk of postoperative neurologic outcomes, such as those undergoing hypothermic circulatory arrest
Bispectral index to ensure adequate level of unconsciousness and eliminate the risk of intraoperative awareness
C.I-2. What is the Allen’s test?
The Allen’s test is used to detect the presence of adequate collateral ulnar arterial circulation. The radial and ulnar arteries are occluded by the examiner’s fingers, and the patient is then asked to make a tight fist repeatedly until the palmar skin is blanched (to empty the blood from the hand). If the patient is under anesthesia, the blood in the hand may be drained by a third person squeezing the hand. The hand is held above the heart level to help venous drainage. Then, the fist is opened slowly and put down to the heart level. Only the ulnar compression is released, and the flush of the palmar skin is watched. The Allen’s test is:
Normal—if the flush appears in less than 7 seconds (presence of adequate collateral connections between the superficial [ulnar] and deep [radial] palmar arches)
Borderline—7 to 15 seconds
Abnormal—greater than 15 seconds
A modified Allen’s test may be done with a Doppler detector or a pulse oximeter. The results of Allen’s test are abnormal in approximately 3% of young healthy individuals. However, Slogoff et al. studied the complications following radial artery cannulation in 1,699 cardiovascular surgical patients. They concluded that in the absence of peripheral vascular disease, Allen’s test is not a predictor of ischemia of the hand during or after radial artery cannulation, and that radial artery cannulation is a low-risk, high-benefit monitoring technique that deserves wide clinical use. However, in the current litigious setting and in the face of some evidence to the contrary, it is probably prudent to continue to perform Allen’s test and document the results. Some authorities still consider the use of Allen’s test as the standard of care.
Alastruey J, Parker KH, Peiró J, et al. Can the modified Allen’s test always detect sufficient collateral flow in the hand? A computational study. Comput Methods Biomech Biomed Engin. 2006;9(6):353-361.
Barbeau GR, Arsenault F, Dugas L, et al. Evaluation of the ulnopalmar arterial arches with pulse oximetry and plethysmography: comparison with the Allen’s test in 1010 patients. Am Heart J. 2004;147(3):489-493.
Brzezinski M, Luisetti T, London MJ. Radial artery cannulation: a comprehensive review of recent anatomic and physiologic investigations. Anesth Analg. 2009;109(6):1763-1781.
Cederholm I, Sørensen J, Carlsson C. Thrombosis following percutaneous radial artery cannulation. Acta Anaesthesiol Scand. 1986;30:227-230.
Hildick-Smith D. Use of the Allen’s test and transradial catheterization. J Am Coll Cardiol. 2006;48(6):1287.
Reich DL, Mittnacht A, London M, et al. Monitoring of the heart and vascular system. In: Kaplan JA, Reich DL, Savino JS, eds. Kaplan’s Cardiac Anesthesia: The Echo Era. 6th ed. Philadelphia, PA: Elsevier Saunders; 2011:416-451.
Slogoff S, Keats AS, Arlund C. On the safety of radial artery cannulation. Anesthesiology. 1983;59:42-47.
C.I-3. Should you monitor temperature from multiple sites?
In many cardiac surgery centers, temperature is measured from two or more sites in order to estimate the accurate core temperature and achieve even distribution of body heat during cooling and rewarming by extracorporeal circulation (ECC). Esophageal, nasopharyngeal, bladder, or tympanic sites measure core temperature; the axillary and rectal sites measure peripheral temperature. Urinary bladder temperature monitoring through a urinary catheter is quite convenient and popular and reflects a body temperature between core and periphery. During cooling and rewarming by ECC, the core temperature changes rapidly, whereas the peripheral temperature changes slowly. The opposite changes are witnessed during surface cooling or warming.
Temperatures are measured during cooling on ECC to ensure that the organs most susceptible to potential hypoperfusion, such as the brain, actually receive the protective effect of the desired hypothermia. In this regard, nasopharyngeal or tympanic membrane temperatures are the monitoring sites, although they, at times, overestimate or underestimate the actual brain temperature. During rewarming, cerebral hyperthermia should be avoided (by maintaining a gradient of 5°C between the arterial blood and brain, with the brain having the lower temperature) and should be corrected immediately because hyperthermia increases the cerebral oxygen consumption and may exacerbate postoperative neuropsychological dysfunction.
Bar-Yosef S, Mathew JP, Newman MF, et al. Prevention of cerebral hyperthermia during cardiac surgery by limiting on-bypass rewarming in combination with post-bypass body surface warming: a feasibility study. Anesth Analg. 2004;99:641-646.
Gravlee GP, Davis RF, Stammers AH, et al, eds. Cardiopulmonary Bypass: Principles and Practice. 3rd ed. Philadelphia, PA: Lippincott Williams & Wilkins; 2008:567-568.
Grocott HP, Stafford-Smith M, Mora Mangano CT. Cardiopulmonary bypass management and organ protection. In: Kaplan JA, Reich DL, Savino JS, eds. Kaplan’s Cardiac Anesthesia: The Echo Era. 6th ed. Philadelphia, PA: Elsevier Saunders; 2011:838-887.
C.I-4. How do you know that the tip of the pulmonary artery (PA) catheter is in the right ventricle (RV) or the PA?
There are three main differences in the pressure tracings, as shown in Figure 5.4.
Diastolic pressure is higher in PA than in RV because the closed pulmonary valve maintains a diastolic gradient between the PA and the RV.
PA pressure: 20 to 25/5 to 10 mm Hg
RV pressure: 20 to 25/0 to 5 mm Hg
Pressure contour
PA pressure tracing has a dicrotic notch, which is created by the closure of pulmonary valve, and the diastolic part is descending (decreasing).
RV pressure tracing has a sharp drop and a plateau in early diastole, and the diastolic part is ascending (increasing).
Ventricular filling
In the late diastolic phase, PA pressure is decreasing because of diastolic runoff in the pulmonary circulation, whereas the RV pressure is increasing because of ventricular filling.
C.I-5. What is normal pulmonary artery occlusion pressure (PAOP)?
Normal—4 to 12 mm Hg
Borderline—13 to 17 mm Hg
Heart failure—over 18 mm Hg
Barash PG, Cullen BF, Stoelting RK, et al. eds. Clinical Anesthesia. 7th ed. Philadelphia, PA: Lippincott Williams & Wilkins; 2013.
C.I-6. Is it necessary to monitor PA pressure for coronary artery operations?
The indication to monitor PA pressure includes the following:
Diagnostic
Diagnosis of shock states
Differentiation of high- versus low-pressure pulmonary edema
Diagnosis of primary pulmonary hypertension
FIGURE 5.4 Pressure tracings of right atrium (RA), right ventricle (RV), pulmonary artery (PA), and pulmonary artery occlusion pressure (PAOP).
Diagnosis of valvular disease, intracardiac shunts, cardiac tamponade, and pulmonary embolus
Monitoring and management of complicated acute MI
Assessing hemodynamic response to therapies
Management of multiorgan system failure and/or severe burns
Management of hemodynamic instability after cardiac surgery
Assessment of response to treatment in patients with primary pulmonary hypertension
Therapeutic: Aspiration of Air Emboli
The indication to monitor PA pressure depends on the ventricular function. For patients who have good left ventricular function (ejection fraction >50% and normal ventricular wall motion), the CVP is considered to correlate well with the PAOP; therefore, PA pressure monitoring may not be necessary for this group of patients. On the other hand, for patients with poor left ventricular function (ejection fractions <40% or prominent wall motion abnormalities), PA pressure monitoring is indicated. Other indications for monitoring PA pressure include the presence of pulmonary hypertension, combined coronary stenosis and valvular disease, and complex cardiac lesions.
However, results of several studies in recent past involving critically ill patients have consistently demonstrated that use of PA catheter was either associated with increased mortality and morbidity or no difference in fatal and nonfatal outcomes as compared to use of CVP alone or other noninvasive monitoring. Much debate exists regarding this topic, and it is unlikely that there ever will be a resolution. Nevertheless, there has been substantive reduction in the use of the PA catheter both in surgical and medical settings.
Binanay C, Califf RM, Hasselblad V, et al. Evaluation study of congestive heart failure and pulmonary artery catheterization effectiveness: the ESCAPE trial. JAMA. 2005;294(13):1625-1633.
Djaiani G, Karski J, Yudin M, et al. Clinical outcomes in patients undergoing elective coronary artery bypass graft surgery with and without utilization of pulmonary artery catheter-generated data. J Cardiothorac Vasc Anesth. 2006;20(3):307-310.
Harvey S, Harrison DA, Singer M, et al. Assessment of the clinical effectiveness of pulmonary artery catheters in management of patients in intensive care (PAC-Man): a randomised controlled trial. Lancet. 2005;366(9484):472-477.
Harvey S, Young D, Brampton W, et al. Pulmonary artery catheters for adult patients in intensive care. Cochrane Database Syst Rev. 2006;(3):CD003408.
Hessel EA, Apostolidou I. Pulmonary artery catheter for coronary artery bypass graft: does it harm our patients? Primum non nocere. Anesth Analg. 2011;113:987-989.
Sandham JD, Hull RD, Brant RF, et al. A randomized, controlled trial of the use of pulmonary-artery catheters in high-risk surgical patients. N Engl J Med. 2003;348(1):5-14.
Schwann NM, Hillel Z, Hoeft A, et al. Lack of effectiveness of the pulmonary artery catheter in cardiac surgery. Anesth Analg. 2011;113:994-1002.
Shah MR, Hasselblad V, Stevenson LW, et al. Impact of the pulmonary artery catheter in critically ill patients: meta-analysis of randomized clinical trials. JAMA. 2005;294(13):1664-1670.
Vender JS. Pulmonary artery catheter utilization: the use, misuse, or abuse. J Cardiothorac Vasc Anesth. 2006;20:295-299.
C.I-7. What are the complications of PA catheterization?
From Venipuncture Sites (as for Central Venous Pressure) Common complications
Infection—sepsis
Hematoma
Air embolism
Thrombosis
Catheter shearing and embolization
Subclavian approach
Pneumothorax
Hemothorax
Hydrothorax
Internal jugular approach
Pneumothorax
Neck hematoma from puncture of carotid artery
Possible vagus nerve injury and brachial plexus injury
Thoracic duct perforation from left-side approach
The basilar or cephalic vein approach has fewer complications, but the failure rate is higher.
From Pulmonary Artery Catheter
Arrhythmias 1.5% to 11%, complete heart block, thromboembolism, pulmonary infarction from continuous wedging, massive hemorrhage from perforation of PA or right atrium (RA), failure to wedge, hemoptysis, intracardiac knotting, balloon rupture, endocardial thrombi, and tricuspid valve injury
Murphy GS, Vender JS. Monitoring the anesthetized patient. In: Barash PG, Cullen BF, Stoelting RK, et al, eds. Clinical Anesthesia. 6th ed. Philadelphia, PA: Lippincott Williams & Wilkins; 2009:707-708.
Reich DL, Mittnacht A, London M, et al. Monitoring of the heart and vascular system. In: Kaplan JA, Reich DL, Savino JS, eds. Kaplan’s Cardiac Anesthesia: The Echo Era. 6th ed. Philadelphia, PA: Elsevier Saunders; 2011:416-451.
C.I-8. What are the hemodynamic consequences of myocardial ischemia? How can you detect myocardial ischemia? Is PAOP a sensitive indicator of myocardial ischemia?
For four decades following classic observation on the effects of coronary occlusion on myocardial contraction, it was believed that transient severe ischemia caused either irreversible cardiac injury—that is, infarction—or prompt recovery. However, in the 1970s, it became clear that after a brief episode of severe ischemia, prolonged myocardial dysfunction with gradual return of contractile activity occurred, a condition termed myocardial stunning. Alternatively, severe chronic ischemia can result in diminished contractile performance manifested by chronic RWMA (hibernation).
Acute myocardial ischemia affects systolic and diastolic cardiac function. Diastolic dysfunction usually manifests before alterations in systolic function. The immediate impact on ventricular compliance is related to the etiology of the ischemic event. Decreased myocardial oxygen supply is initially accompanied by an increase in compliance. In contrast, increased myocardial oxygen demand is associated with an immediate loss of ventricular compliance (e.g., the ventricle becomes stiffer). Therefore, the ventricle requires a higher filling pressure (end-diastolic pressure) to maintain a given stroke volume. A cascade of events occurs, which may include wall motion abnormalities, dysrhythmias, and conduction block. An 80% reduction of coronary blood flow causes akinesis (<10% of radial systolic thickening), whereas a 95% decrease causes dyskinesis (thinning, instead of thickening, during systole). If the ischemia becomes severe, the continuously elevated LVEDP will lead to pulmonary edema (Fig. 5.5).
Although a number of sensitive techniques are available for detection of ischemia, such as magnetic resonance spectroscopy, radiolabeled lactate determinations, or direct measurement of end-diastolic pressure, but all are impractical intraoperatively. The most popularly recognized sign of ischemia is ECG ST-segment changes. The ECG criteria for ischemia are horizontal or down-sloping ST-segment depression at least 0.1 mV at 0.06 second from J-point, up-sloping ST-segment depression at least 0.2 mV at 0.08 second from J-point, and ST-segment elevation at least 0.15 mV (1 mV = 10 mm). Other ECG signs of ischemia include inverted T waves and a new onset of arrhythmias or conduction abnormalities.
RWMA, detected with two-dimensional echocardiography, have been shown to be the earliest and most sensitive sign of myocardial ischemia. RWMA appear with just 25% decrease in coronary blood flow in the absence of ECG changes, whereas ECG signs of ischemia are apparent with a 50% decrease in coronary blood flow. During exercise, patients with CAD develop RWMA after 30 seconds, whereas ECG changes do not occur until after 90 seconds. RWMA was four times more sensitive than ECG ST-segment change in detecting intraoperative ischemia. Moreover, ST-segment changes cannot be analyzed if the patient has conduction disturbances, such as bundle branch block or ventricular-paced rhythms. Worsening wall motion after CABG surgery should be considered a prognostic indicator of adverse cardiovascular outcome.
Measurement of PAOP has been suggested as an early and sensitive indicator of ischemia to be used when the ECG is not diagnostic. Although acute increases in PAOP or development of V waves may reflect ischemia, the absence of a change in PAOP does not ensure the absence of ischemia. Häggmark et al. reported that the sensitivity, specificity, and predictive value (positive and negative) of PAOP abnormalities for ischemia ranged between 40% and 60%. Leung et al. found that 61% of TEE wall motion abnormalities in CABG patients occurred without significant changes in HR, systolic arterial pressure, or PA pressure. Only 10% of episodes were accompanied by 5 mm Hg or greater changes in PA pressure. These studies question the value of PA catheterization and monitoring for detection of intraoperative ischemia.
Häggmark S, Hohner P, Ostman M, et al. Comparison of hemodynamic, electrocardiographic, mechanical, and metabolic indicators of intraoperative myocardial ischemia in vascular surgical patients with coronary artery disease. Anesthesiology. 1989;70:19-25.
Leung JM, O’Kelly B, Browner WS, et al. Prognostic importance of postbypass regional wall-motion abnormalities in patients undergoing coronary artery bypass graft surgery. SPI Research Group. Anesthesiology. 1989;71:16-25.
Mantha S, Ochroch EA, Roizen MF, et al. Anesthesia for vascular surgery. In: Barash PG, Cullen BF, Stoelting RK, et al, eds. Clinical Anesthesia. 6th ed. Philadelphia, PA: Lippincott Williams & Wilkins; 2009:1108-1135.
Mittnacht AJC, Weiner M, London MJ, et al. Anesthesia for myocardial revascularization. In: Kaplan JA, Reich DL, Savino JS, eds. Kaplan’s Cardiac Anesthesia: The Echo Era. 6th ed. Philadelphia, PA: Elsevier Saunders; 2011:522-569.
Swaminathan M, Morris RW, De Meyts DD, et al. Deterioration of regional wall motion immediately after coronary artery bypass graft surgery is associated with long-term major adverse cardiac events. Anesthesiology. 2007;107:739-745.
C.I-9. How would you monitor electrocardiogram (ECG)? Why V5? If you do not have precordial leads in your ECG machine, how can you monitor the left ventricle (LV)?
Multiple-lead ECG monitoring provides the best clinically available method of detecting perioperative ischemia. Based primarily on results obtained from exercise treadmill testing, 96% of ischemic events can be detected by combining ECG leads II and V5; therefore, leads II and V5 are the optimal leads for intraoperative myocardial ischemia. However, London et al. found that the standard combination of leads II and V5 was only 80% sensitive, whereas combining leads V4 and V5 increased sensitivity to 90% in patients with known or suspected CAD undergoing noncardiac surgery with general anesthesia. The sensitivity increased to 96% by combining leads II, V4, and V5. If only one lead can be displayed, V5 should be used because lead V5 has the greatest sensitivity: 75% intraoperatively and 89% during exercise treadmill testing. See also Chapter 14, section C.3.
London MJ, Hollenberg M, Wong MG, et al. Intraoperative myocardial ischemia: localization by continuous 12-lead electrocardiography. Anesthesiology. 1988;69:232-241.
C.I-10. Discuss the principles and clinical applications of intraoperative transesophageal two-dimensional echocardiography.
TEE is a well-established technique to visualize cardiac anatomy and function. Echocardiography is based on fundamental ultrasonic principles. Ultrasound is defined as sound above the upper threshold of human hearing (20,000 Hz). The ultrasound waves (1 to 7 MHz) are created by applying an alternating electric current of 500 to 1,500 pulses per second on a piezoelectric crystal. A short burst (pulse) of high-frequency, low-intensity sound is then emitted and directed through the human body to detect boundaries between structures of different acoustic impedance. The ultrasound wave is partially reflected at the boundary of media with different acoustic impedance, a property that is primarily determined by the slight difference in density between different tissues. The transmission of ultrasound through the heart, with detection of the returning echoes detailing the position and movement of cardiac structures, is termed cardiac ultrasound or echocardiography. Two-dimensional ultrasound reconstructs cardiac anatomy in shades of gray, from the time delay and decreased intensity of the reflected ultrasound. The ultrasound beam sweeps in an arc to give a panoramic view of the heart that results in cross-sectional images that are anatomically recognizable. With M-mode ultrasound, the ultrasonic beam is aimed in one direction only and therefore depicts only one dimension of the target structure in an image that does not resemble cardiac structure. See also Chapter 7, section A.6.
Doppler echocardiography blood flow is measured by applying Doppler frequency shift analysis to echoes reflected by a moving target (red blood cells or cardiac tissue). According to the Doppler principle, the frequency of the reflected ultrasound is different from the frequency of the emitted ultrasound. This change in frequency is proportional to the speed of the moving target. Color-coded Doppler flow imaging (color flow Doppler) simultaneously presents real-time images of intracardiac blood flow and moving cardiac structures in two dimensions. Color flow Doppler permits the evaluation of valvular function and intracardiac shunts.
The clinical applications of intraoperative echocardiography are as follows:
Monitoring ventricular volume and function. When left ventricular short-axis cross section is monitored at the level of the midpapillary muscles, TEE provides the anesthesiologist with a direct, semiquantitative method to assess left ventricular preload and ejection in real time and guide the administration of fluids and inotropes.
Monitoring diastolic function. Both Doppler assessment of transmitral and transpulmonary vein flow patterns can provide important information about diastolic dysfunction that may be prevalent in as many as 50% of patients presenting for open heart surgery, namely, those with history of hypertension, aortic stenosis, and hypertrophic cardiomyopathy. It is important to note that in patients with valvular disease, a component of LV dysfunction arises from abnormal diastolic function.
Ischemic heart disease. RWMA, such as hypokinesia, akinesia, and dyskinesia, are the earliest signs of myocardial ischemia (Figs. 5.2 and 5.3), as discussed in section C.I-8.
Measurements of systolic wall thickening, another excellent sign of myocardial ischemia, are not reproducible because of a difficulty in delineating the epicardial border accurately in two-dimensional echocardiography. The impairment of the diastolic function can be qualitatively estimated, and the filling pressures can be estimated using a combination of spectral Doppler measurements of the blood flow through the pulmonary veins and the mitral valve.
Air embolism. In the left atrium, LV, and aorta, air embolism can be detected by TEE during open heart surgery. Therefore, TEE can be used to help the surgeon evacuate air bubbles from the left heart before the heart ejects blood and air into the systemic circulation.
Valvular regurgitation. Valvular regurgitation can be determined intraoperatively by color Doppler echocardiography before and immediately after conservative valve repair (annuloplasty, commissurotomy) or valve replacement. The degree of valvular incompetency can be accurately measured using a combination of two-dimensional and Doppler techniques (Figs. 5.6 and 5.7), and the function of the repaired or replaced valve can be quantitatively evaluated.
Valvular stenosis. Spectral Doppler echocardiography can be used intraoperatively to measure the pressure gradient across a valvular stenosis and calculate the area of the diseased valve.
Congenital heart disease. TEE techniques allow a more aggressive approach to complex cardiac reconstructions because the surgeon has the ability to visualize the heart and evaluate adequacy of the surgical repair immediately after the operative procedure.
Thoracic aorta. TEE can accurately diagnose thoracic aortic aneurysm, dissection, disruption, and atheroma. Epiaortic echocardiography is performed to examine the ascending aorta and arch if severe atheroma is found in the descending aorta or arch by TEE. Severe atheroma of thoracic aorta is an independent predictor of postoperative neurologic outcome. The atheroma is graded as follows: grade I, normal to mild intimal thickening; grade II, severe intimal thickening; grade III, atheroma protruding less than 5 mm into the lumen; grade IV, atheroma protruding greater than 5 mm; and grade V, atheroma with a mobile component (Fig. 5.8).
Cardiac tumors. Atrial myxoma can be easily diagnosed by TEE. Preoperative and postoperative TEE with contrast can assess the presence and severity of mitral regurgitation secondary to valve damage from a ball-valve effect of the myxoma.
Other cardiac pathology. Hypertrophic obstructive cardiomyopathy (HOCM) (idiopathic hypertrophic subaortic stenosis) can be identified by the combination of systolic anterior motion of the mitral valve and left ventricular outflow obstruction. Cardiac tamponade caused by a pericardial effusion or hematoma can be detected.
Neurosurgery. TEE was found to be the most sensitive monitor to detect venous air embolism during neurosurgical operations in the sitting position. Patent foramen ovale and paradoxical air embolism can be detected by TEE.
Monitoring for noncardiac surgery. Recent guidelines by Porter et al. suggest the utility of TEE in healthy patients who are acutely hemodynamically unstable during surgery, critically ill patients with significant respiratory and cardiac diseases undergoing noncardiac surgery, and patients undergoing high-risk noncardiac procedures, such as orthopedic surgery and kidney and liver transplants. TEE would be used as a hemodynamic diagnostic and monitoring tool to guide and evaluate effectiveness of therapeutic interventions.
Agricola E, Oppizzi M, Melisurgo G, et al. Transesophageal echocardiography: a complementary view of the heart. Expert Rev Cardiovasc Ther. 2004;2(1):61-75.
Kahn RA, Shernan SK, Konstadt SN. Intraoperative echocardiography. In: Kaplan JA, Reich DL, Savino JS, eds. Kaplan’s Cardiac Anesthesia: The Echo Era. 6th ed. Philadelphia, PA: WB Saunders; 2011:315-382.
Kühl HP, Hanrath P. The impact of transesophageal echocardiography on daily clinical practice. Eur J Echocardiogr. 2004;5(6):455-468.
Mahmood F, Matyal R. A quantitative approach to the intraoperative echocardiographic assessment of the mitral valve for repair. Anesth Analg. 2015;121:34-58.
Porter TR, Shillcutt SK, Adams MS, et al. Guidelines for the use of echocardiography as a monitor for therapeutic intervention in adults: a report from the American Society of Echocardiography. J Am Soc Echocardiogr. 2015;28(1):40-56.
Shapira Y, Vaturi M, Weisenberg DE, et al. Impact of intraoperative transesophageal echocardiography in patients undergoing valve replacement. Ann Thorac Surg. 2004;78(2):579-583.
C.I-11. How would you induce anesthesia?
Midazolam 1 to 2 mg is given as soon as the standard monitors are applied and prior to the arterial line is inserted (under local anesthesia). This is important in alleviating anxiety, which can exacerbate myocardial ischemia. A smooth induction is essential to prevent hypotension, hypertension, and tachycardia. Different techniques may be used to achieve a smooth induction. For patients with good left ventricular function, anesthesia is induced with fentanyl, 2 to 5 µg per kg, and propofol, 1 to 2 mg per kg. The patient is ventilated by mask with 100% oxygen. After administration of a nondepolarizing muscle relaxant (vecuronium, rocuronium), the trachea is intubated. The choice of nondepolarizing muscle relaxant varies extremely from center to among practitioners. Succinylcholine is used less frequently in cardiac surgery. If the patient has a history of hypertension or the initial systolic BP is over 150 mm Hg, lidocaine or a β-blocker (or a mixed α– and β-blocker) is administered. For patients with poor left ventricular function, potent inhalation agents such as isoflurane, sevoflurane, and desflurane should be used cautiously during induction of anesthesia because of their dose-dependent reduction in myocardial contractility and systemic arterial resistance. In lieu of the increasing incidence of delirium in the elderly postoperatively, benzodiazepines should be avoided in patients older than 70 years. Alternatively, etomidate 0.2 mg per kg may be administered for induction. However, it is more important to maintain stable hemodynamics than the choice of induction anesthetic agents. See also
Chapter 11, section C.6 for other measures to prevent tachycardia and hypertension at the time of intubation.
Chapter 11, section C.6 for other measures to prevent tachycardia and hypertension at the time of intubation.
Kaplan JA, Reich DL, Savino JS, eds. Kaplan’s Cardiac Anesthesia: The Echo Era. 6th ed. Philadelphia, PA: Elsevier Saunders; 2011:522-569.
Mangusan RF, Hooper V, Denslow SA, et al. Outcomes associated with postoperative delirium after cardiac surgery. Am J Crit Care. 2015;24:156-163.
C.I-12. How would you maintain anesthesia?
Again, smooth anesthesia is essential to achieve a balance between myocardial oxygen demand and supply. Different agents and techniques may be used to accomplish the same goal. A combination of fentanyl (or another synthetic opioid) and isoflurane, sevoflurane, or desflurane (or propofol) is a popular choice. After the patient is intubated, a mixture of 60% air and 40% oxygen is administered to lessen the possibility of oxygen toxicity. Clearly, this ratio is subject to underlying pulmonary disease and ongoing intraoperative clinical conditions.
The depth of anesthesia must be titrated to meet the requirements of the varying intensities of surgical stimulation. Skin incision and sternal splitting are very painful. However, the strongest stimulation is usually from sternal retraction with the self-retaining retractor. Fentanyl, around 5 µg (0.1 mL) per kg (or another synthetic opioid), is given right before the skin incision, before sternotomy, and intermittently throughout surgery as needed to maintain adequate analgesia. Very high doses of fentanyl or sufentanil have been successfully used for cardiac anesthesia. There have been significant changes in practice over the past decade, whereby much lower doses of narcotic are administered to facilitate early extubation. Nowadays, maintenance of anesthetic is based on administration of volatile agents and smaller opioid dosages as was the practice in the 1980s and 1990s. Vasoactive agents are also used to regulate the hemodynamics (HR, systemic arterial pressure, and filling pressures).
Kaplan JA, Reich DL, Savino JS, eds. Kaplan’s Cardiac Anesthesia: The Echo Era. 6th ed. Philadelphia, PA: Elsevier Saunders; 2011:522-569.
C.I-13. What is the better anesthetic agent for this operation—an inhalation or intravenous (IV) agent?
Both inhalation, IV, and combined agents have been used successfully. Both have advantages and disadvantages. Understanding the cardiovascular effects of each anesthetic agent and careful titration of each drug will improve the balance between myocardial oxygen demand and supply. Early detection and appropriate control of the major determinants of myocardial oxygen consumption (BP, HR, PAOP) are mandatory if myocardial ischemia is to be avoided. Another consideration is the desirability of early extubation. High-dose narcotic and large doses of sedatives and muscle relaxants should be avoided if early extubation is planned.
Studies point toward a beneficial effect of volatile agents in preconditioning the myocardium against an ischemic injury. De Hert et al. reviewed various animal studies that demonstrate exposure of myocardium to volatile anesthetics before ischemia prevents ischemia-reperfusion injury, resulting in better recovery and cardiac function after ischemia. Although in two metaanalyses, Symons and Myles and Yu and Beattie reported that anesthetic choice did not affect incidence of perioperative morbidity and mortality, another two suggest that there is a benefit. In one of these, Landoni et al. performed a meta-analysis isolating studies comparing either desflurane or sevoflurane to total intravenous anesthesia (TIVA) and found that desflurane and sevoflurane significantly decreased the rate of MI and death in patients undergoing cardiac surgery as well as other end points of decreased cardiac biomarker release, MI, and postoperative use of inotropic drugs. Currently, there is significant variability in anesthetic technique during cardiac surgery and no consensus on which technique is superior for cardioprotection.
De Hert SG, Turani F, Mathur S, et al. Cardioprotection with volatile anesthetics: mechanisms and clinical implications. Anesth Analg. 2005;100:1584-1593.
De Hert S, Vlasselaers D, Barbé R, et al. A comparison of volatile and non volatile agents for cardioprotection during on-pump coronary surgery. Anaesthesia. 2009;64(9):953-960.
Guarracino F, Landoni G, Tritapepe L, et al. Myocardial damage prevented by volatile anesthetics: a multicenter randomized controlled study. J Cardiothorac Vasc Anesth. 2006;20(4):477-483.
Landoni G, Greco T, Biondi-Zoccai G, et al. Anaesthetic drugs and survival: a Bayesian network meta-analysis of randomized trials in cardiac surgery. Br J Anaesth. 2013;111:886-896.
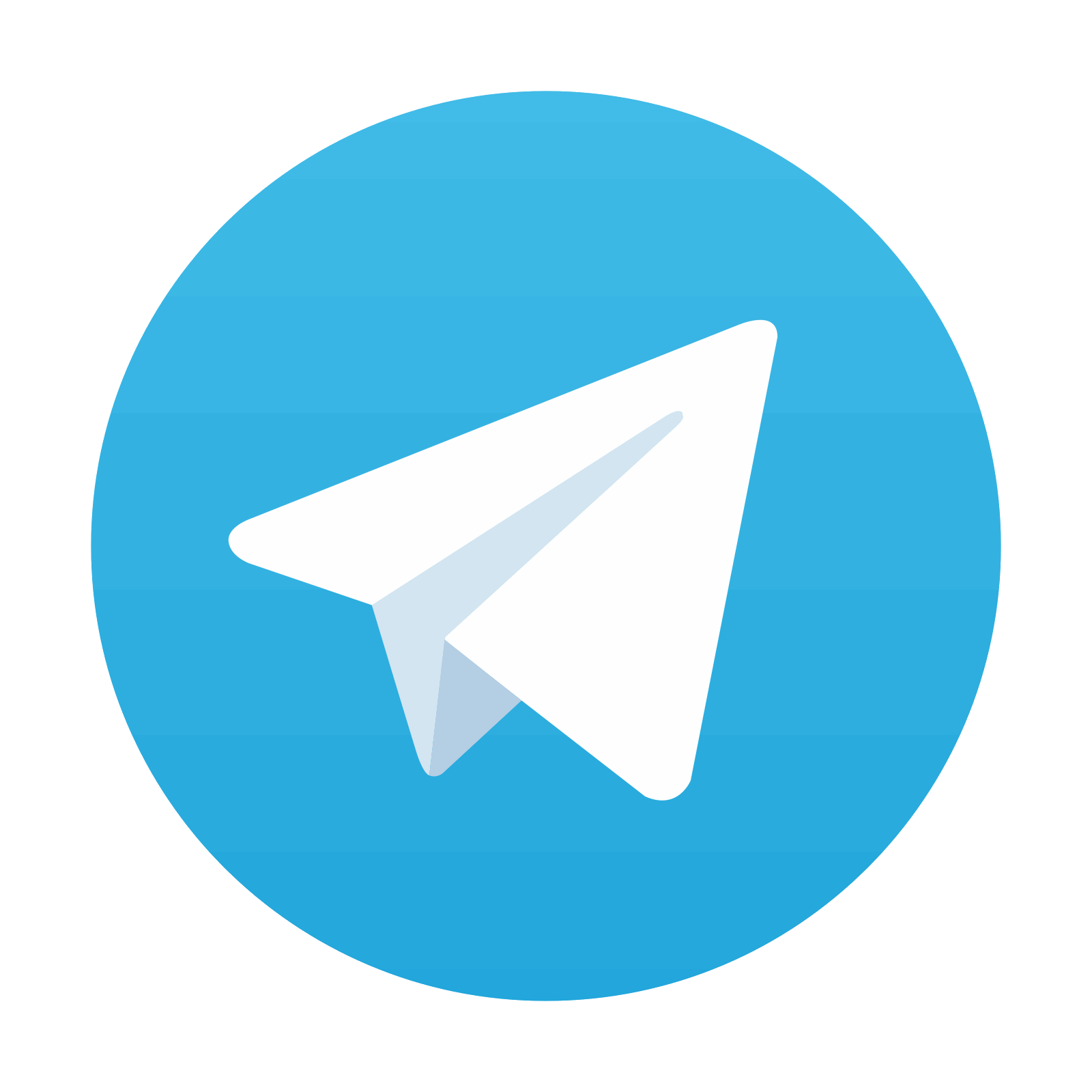
Stay updated, free articles. Join our Telegram channel

Full access? Get Clinical Tree
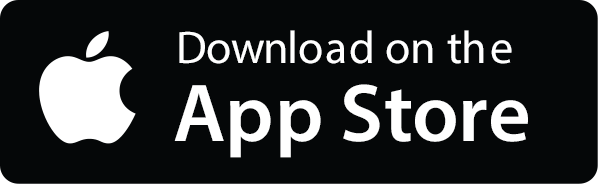
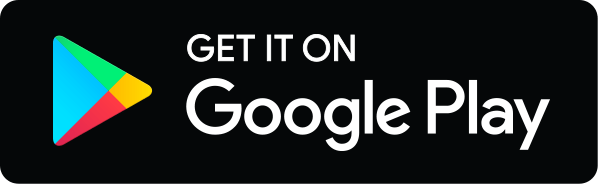
