Introduction
The contents of the cranium can be divided into three major constituents. The brain or tissue compartment accounts for approximately 85% of the total intracranial volume, cerebrospinal fluid (CSF) contributes approximately 10%, and the blood in the vasculature contributes approximately 5%. The majority of the cerebral blood volume (CBV) resides in the low-pressure venous system, whereas only 15% of the CBV is found in the arteries and 15% in the venous sinuses.
Intracranial pressure (ICP) is closely regulated, even in the presence of a space-occupying lesion, as long as compensatory mechanisms are operational and the pathologic process evolves slowly. Any increase in intracranial volume must be compensated for by volume reduction of one of the other compartments to maintain normal ICP. The CSF system has the greatest buffering capacity through displacement of CSF from the cranium to the spinal subarachnoid space. CBV reduction occurs first by compression of the low-pressure venous system, followed by capillary collapse, and then arterial compression leading to cerebral ischemia. The impact of ICP on outcome lies in its role in determining cerebral perfusion pressure (CPP) (CPP = mean arterial pressure [MAP] − ICP). There is evidence, at least in head trauma, that a CPP less than 50 mm Hg is associated with a poor outcome. However, an improved outcome does not necessarily result from a higher CPP. For the calculation of CPP, the arterial pressure transducer should be at the level of the ear.
Increased ICP may be caused by changes in the volume of any one or a combination of the intracranial compartments, including hematomas caused by vascular rupture, increases in brain and interstitial volumes caused by tumors, and vasogenic and cytotoxic edema secondary to hypoxia and infection. Increased ICP can also result from obstruction of CSF pathways and alteration of CSF production or reabsorption.
Options
Management strategies include decisions about the choice of (1) anesthetic drugs, (2) ventilation, (3) hyperosmolar therapy, (4) head and body position, and (5) decompressive craniectomy ( Box 62-1 ). The effects are influenced by whether the ICP increase developed rapidly or slowly; in the latter case, usually some compensation can take place.
Standards
No prophylactic hyperventilation
Guidelines
Monitoring of ICP
Barbiturate infusion for intractable increased ICP
Use of mannitol or hypertonic saline
Options
Cerebral perfusion pressure 50-70 mm Hg
Brief hyperventilation for acute ICP increase
Propofol infusion for sedation
Positioning patient head up
Evidence/Controversies/Areas of Uncertainty/Guidelines
What Are the Targets for Intracranial Pressure and Cerebral Perfusion Pressure?
The ICP should be kept below 20 mm Hg because higher values are associated with poorer neurologic outcomes. A CPP greater than 70 mm Hg should be avoided if it requires massive fluid infusion and high-dose catecholamines because hypervolemia and catecholamine therapy increase the incidence of acute respiratory distress syndrome. A spontaneous increase of CPP above 70 mm Hg can be accepted as long as cerebrovascular autoregulation is intact or the neurologic state seems to benefit clinically. When autoregulation is intact, an increase in CPP is associated with autoregulatory vasoconstriction, thereby reducing CBV and ICP. The critical lower threshold for CPP lies between 50 and 60 mm Hg. Therefore a CPP below 50 mm Hg should be avoided.
What Are the Effects of Anesthetics on Intracranial Pressure?
The choice of anesthetic agents and adjunctive drugs is based on consideration of their effects on cerebral blood flow (CBF), CBV, cerebral metabolic rate of oxygen (CMRO 2 ), ICP, cerebrovascular autoregulation, and carbon dioxide (CO 2 ) reactivity. Most randomized trials have focused on these surrogate endpoints rather than on clinical or neurologic patient outcomes.
Volatile anesthetics depress cerebral metabolism in a dose-dependent fashion while directly inducing cerebral vasodilation, which results in increases in CBV and ICP. Sevoflurane causes less cerebral vasodilation compared with isoflurane or desflurane. Cerebrovascular autoregulation and CO 2 response remain intact with sevoflurane up to 1 minimum alveolar concentration (MAC); therefore it is suitable for neurosurgical patients as long as ICP is not markedly or rapidly increasing.
Nitrous oxide is a potent cerebral vasodilator causing a resultant increase in ICP. Although no outcome studies have demonstrated a deleterious effect, nitrous oxide should not be used in patients with rapidly elevated ICP.
Total intravenous anesthesia has received attention in neuroanesthesia as a means of avoiding the vasodilating effects of nitrous oxide and volatile anesthetics. Intravenous agents such as propofol and etomidate produce cerebral vasoconstriction and a reduction in CBF, CBV, and ICP secondary to a decrease in CMRO 2 while preserving autoregulation. Propofol should be used intraoperatively in patients with markedly or rapidly increasing ICP, at least until the mass or the ICP has been reduced. In the intensive care unit propofol can be used for up to 3 to 4 days in a maximal dosage of 4 mg/kg/h. Propofol administered for longer or at higher concentrations might induce the propofol infusion syndrome, which includes hyperkalemia, lipemia, metabolic acidosis, myocardial failure, rhabdomyolysis, and renal failure potentially resulting in death. All patients with prolonged propofol sedation should be monitored for symptoms of the propofol infusion syndrome. Dexmedetomidine, an alpha-2-adrenergic agonist that is used for sedation of patients in the intensive care unit seems to provide adequate sedation and has no negative influence on ICP.
Barbiturates similarly exert their ICP-lowering effects through vasoconstriction and cause a reduction of CBF and CBV secondary to suppression of cerebral metabolism. Barbiturates can produce ICP control and improved CPP in patients with severe head trauma when other treatments have failed, but there is no evidence that prophylactic barbiturate therapy improves outcomes. Furthermore, high doses of barbiturates decrease immune function and can cause hypokalemia. The slow plasma clearance of barbiturates is another disadvantage because it causes a substantial delay in awakening. Barbiturate coma should be titrated to achieve an electroencephalogram (EEG) burst suppression ratio of 5% to 10% or ICP control.
Propofol and barbiturates reduce MAP, and intravenous fluids and vasopressors to elevate CPP are associated with a better chance of survival of patients in a barbiturate or propofol coma. Etomidate causes less cardiovascular depression and may be the drug of choice in cardiovascular disease or hypovolemia, but its use should be confined to induction because it suppresses the adrenocortical response to stress.
There has been controversy about the effect of opioids on ICP. In one study transient increases in ICP without changes in middle cerebral artery blood flow velocity occurred concomitant with decreases in MAP, whereas in patients with stable blood pressure, ICP was unchanged. This suggests that increases in ICP seen with sufentanil and other opioids may be due to autoregulatory vasodilation secondary to systemic hypotension. This is consistent with a more recent study of remifentanil in patients with head trauma.
Nondepolarizing neuromuscular relaxants have no effect on CBF, CMRO 2 , and ICP, whereas succinylcholine may transiently increase ICP. Pretreatment with a nondepolarizing neuromuscular relaxant avoids the effect of succinylcholine on the ICP. When appropriate for rapid sequence intubation, rocuronium should be used.
What Is the Effect of Hyperventilation on Intracranial Pressure?
Arterial CO 2 tension is a potent modulator of cerebrovascular tone and CBF. Arterial hypercapnia dilates cerebral blood vessels, decreases cerebrovascular resistance, and increases CBF, CBV, and ICP, whereas hypocapnia has the opposite effect. Hyperventilation is often used in patients with increased ICP to reduce CBV. However, hyperventilation reduces ICP through vasoconstriction of cerebral arteries, and this could critically affect the oxygen and glucose delivery to vulnerable brain areas.
Hyperventilation lowers global hemispheric CBF but does not alter CMRO 2 . This mismatch between low CBF and normal or elevated CMRO 2 caused by hyperventilation after severe traumatic brain injury may lead to cerebral ischemia, which might further compromise neuronal outcome.
Although hyperventilation may produce a rapid reduction in ICP and high ICP is one of the most common precursors of death or neurologic disability, there is no evidence to suggest that hyperventilation improves clinically relevant outcomes. Patients with severe traumatic brain injury were randomly assigned to a hyperventilated group (PaCO 2 , 25 ± 2 mm Hg) or a normoventilated group (PaCO 2 , 35 ± 2 mm Hg) for 5 days. Patients in the hyperventilation group had a significantly worse outcome at 3 months than did those in the normocapnic group.
In elective supratentorial craniotomy, aggressive hyperventilation (PaCO 2 , 25 ± 2 mm Hg) has been found in a multicenter randomized trial to reduce ICP and improve operating conditions as assessed by surgeons unaware of treatment group allocation. This effect was independent of the use of total intravenous propofol anesthesia or less than 0.8 MAC isoflurane. The hyperventilation was not maintained for the duration of the surgery, and the study did not attempt to determine whether the hyperventilation altered neurologic outcomes. Therefore short periods of hyperventilation to manage rapidly increasing ICP can be accepted.
What Is the Effect of Mechanical Ventilation on Intracranial Pressure?
The effect of positive end-expiratory pressure (PEEP) on ICP has been reported by many investigators without a clear consensus. Mechanical ventilation and PEEP can increase intrathoracic pressure, may increase ICP by impeding venous drainage, or could reduce CPP by reducing blood pressure. Studies suggest that if CPP is maintained, PEEP (up to 15 cm H 2 O) seems to have no significant adverse effect. If an adverse effect of PEEP on ICP occurs, it can often be overcome by placing the patient in the head-up position.
Volume recruitment maneuvers with high-peak intrathoracic pressure reduce MAP, increase ICP, and decrease CPP. This technique affects cerebral hemodynamics and can only be recommended when severe lung injury is leading to hypoxia, which, in turn, can increase neuronal injury. Recruitment maneuvers should be performed carefully and under continuous control of ICP and CPP.
What Is the Effect of Hyperosmolar Therapy on Intracranial Pressure?
The administration of mannitol (0.25 to 1.0 g/kg) has been a cornerstone of ICP management. Because of side effects (e.g., tubular necrosis of the kidney) a dose of 4 g/kg/day should not be exceeded and the serum osmolarity has to be kept below 320 mOsm/L. The prophylactic administration of mannitol can cause side effects, so it should only be used in patients with increased ICP.
Mannitol has an immediate plasma-expanding effect that reduces blood viscosity, thereby increasing CBF, which, in turn, induces autoregulatory vasoconstriction. This rheologic effect might explain the early decrease in ICP. Osmotic agents withdraw more water from the brain tissue than from other organs because the blood–brain barrier (BBB) impedes penetration of the osmotic agent into the brain, thus maintaining an osmotic diffusion gradient. Osmotic diuretics may also reduce ICP by retarding CSF formation. However, when the BBB is disrupted, hyperosmolar fluids may enter and raise brain osmolality, pulling water back into the brain (rebound). Interstitial accumulation of mannitol is most marked with continuous infusions; therefore it is recommended that mannitol be administrated as repeated boluses rather than as a continuous infusion. The evidence supporting mannitol is sufficiently strong to warrant guideline status. A bolus of mannitol is also recommended in patients with transtentorial herniation or progressive neurologic deterioration not attributable to extracranial causes.
Hypertonic saline is an alternative to mannitol for hyperosmotic therapy. It also reduces the cerebral water content, and its effect on ICP seems to be equal or even superior to mannitol, especially when short-term ICP outcome is assessed. Hypertonic saline also does not cause systemic hypotension secondary to osmotic diuresis. The tested concentrations of hypertonic saline for clinical use range from 2% to 23.5%, but no consensus exists regarding the optimal concentration. At the moment it is unclear whether hypertonic saline also promotes a rebound phenomenon.
What Is the Effect of the Patient’s Position on Intracranial Pressure?
Flexion or torsion of the neck can obstruct cerebral venous outflow and increase brain bulk and ICP. A simple change in head position can immediately decrease ICP. Between 30 and 40 degrees head-up or reverse Trendelenburg position is also effective in reducing ICP as long as MAP is maintained.
What Is the Effect of Decompressive Craniectomy on Intracranial Pressure?
Decompressive craniectomy and opening of the dura mater may be a useful option when maximal medical treatment has failed to control ICP. In children, decompressive craniectomy is recommended as a therapy to control ICP. The prognosis after decompression depends on the clinical signs and symptoms at the time of admission, the patient’s age, and the existence of major extracranial injuries. One criticism of decompressive craniectomy is that more patients survive in a vegetative state. So that this outcome can be avoided, decompressive craniectomy should be restricted to patients younger than 50 years without multiple traumatic injuries or patients younger than 30 years in the presence of major extracranial injuries; it should never be used in patients with a primary brainstem lesion.
A significant decrease in ICP and length of stay in the intensive care unit was shown after decompressive craniectomy in 155 adults with severe diffuse brain trauma in the DECRA study, but patients’ neurologic outcomes deteriorated. However, in this study no patients with mass lesions were included, the operative technique might have been inadequate, and the ICP in patients was only at the upper normal limit. This might explain the unfavorable outcome of the DECRA study. It is hoped that the Randomised Evaluation of Surgery with Craniectomy for Uncontrollable Elevation of Intra-Cranial Pressure (RESCUEicp) study will clarify this existing confusion about the indication for a decompressive craniectomy in patients with head trauma. After a massive ischemic stroke and elevated ICP, surgical decompression reduced the risk of death as a short-term outcome in patients who were 60 years or younger.
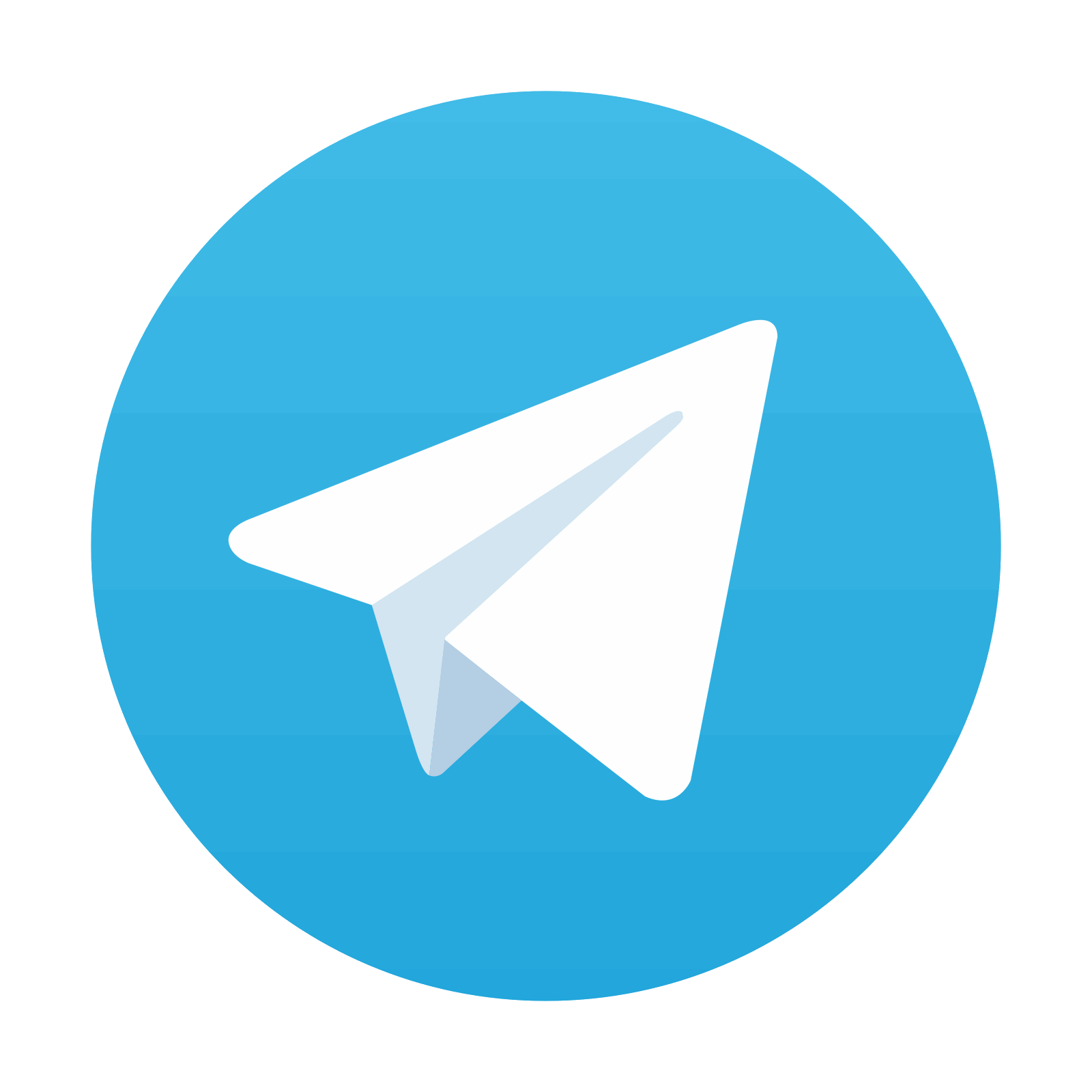
Stay updated, free articles. Join our Telegram channel

Full access? Get Clinical Tree
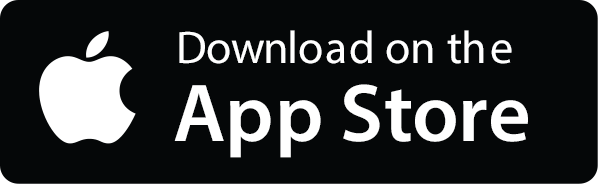
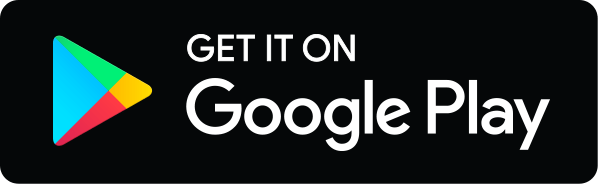
