In 1616, William Harvey described the relationship of the right ventricle (RV) to the pulmonary circulation. For many years after that, this cardiac chamber has been underappreciated. Indeed, in 1943, after demonstrating that ablation of the RV free wall in dogs had little effect on central venous pressure (CVP), Starr concluded that the RV was merely a passive conduit.
In 1974, the RV’s importance reemerged when Cohn and colleagues noticed that RV myocardial infarction (MI) was common and difficult to manage. We now appreciate that RV involvement in inferior MI increases mortality eightfold, and RV dysfunction in acute pulmonary embolism (PE) is a predictor of mortality.
RV failure is defined as the inability of that chamber to provide adequate blood flow through the pulmonary circulation at a normal CVP. Right ventricular failure is common and coexists with a broad range of critical illnesses, including respiratory failure, sepsis, PE, and right ventricular MI. Nonetheless, the RV still remains poorly studied when compared with the left ventricle (LV). Cardiologists focus on the LV, pulmonologists tend to concentrate on the causes and treatment of pulmonary arterial hypertension (PAH), and both neglect the RV. In fact, the RV is barely mentioned in American College of Cardiology (ACC)/American Heart Association (AHA) practice guidelines, and no guidance is provided for management of RV dysfunction.
The heterogeneity of illnesses and varying degrees of disease severity make randomized controlled trials difficult to conduct in the critically ill patient with RV dysfunction. Most intensive care unit (ICU) therapies are instituted on the basis of pathophysiologic considerations and extrapolation from trials in other settings. Because of these difficulties, this review begins with brief consideration of normal and abnormal RV function.
Physiology
The physiology of the RV differs dramatically from that of the LV. The RV is not simply a weak LV. The RV wall is 3 to 4 times thinner than the normal LV wall. RV contraction moves from the apex to its outflow tract in a peristaltic-like motion. The normal RV generates one sixth of the work of the LV while moving the same volume of blood. The easily distensible RV pumps blood into the low-pressure pulmonary circuit, allowing the chamber to accommodate dramatic variations in venous return while maintaining constant cardiac output. Global function of the RV depends on contributions from the interventricular septum and the RV free wall.
Pathophysiology
The RV first responds to increased afterload by increasing contractility and later by dilating according to the Frank-Starling mechanism. Guyton showed that, with progressive constriction of the pulmonary artery, generated RV pressure increases until the RV can no longer compensate, at which point systemic pressure (SP) and cardiac output fall (see Fig. 54-1 ). As RV SP increases, ischemia may ensue.

When RV failure occurs, either due to excessive contractile demand or impaired contractile function, CVP will increase. RV dilation ultimately occurs. Eventually, as increased wall stress impairs contraction and impinges on the LV through the interventricular septum, the process becomes maladaptive ( Fig. 54-2 ).

Diagnosis
No one sign, symptom, or laboratory test perfectly identifies RV failure. However, RV failure is not present if the jugular venous pressure is normal. A parasternal heave, right third heart sound, loud P 2 , tricuspid regurgitation murmur, hepatomegaly, ascites, and peripheral edema may be present in RV failure. Electrocardiography (ECG) findings are nonspecific, but right axis deviation, R/S greater than 1 in V 1 , or P-pulmonale may be seen. Absence of pulmonary congestion with elevated CVP has been considered most specific for RV failure. However, severe RV failure can shift the interventricular septum and increase left ventricular end diastolic pressure (LVEDP), which may cause pulmonary congestion (see Fig. 54-2 ). Even in the absence of LV dysfunction, serum brain natriuretic peptide (BNP) level may be increased with RV volume overload or RV pressure overload, although lower values are lower than those observed in LV failure. BNP levels are predictive of survival in acute RV failure from PAH. In one study of patients with chronic thromboembolic PAH, BNP levels greater than 168 ng/mL identified patients with RV dysfunction with a sensitivity of 88% and specificity of 86%.
Assessment of RV function can be challenging because of the ventricle’s complex geometry. Cardiac magnetic resonance imaging (MRI) is now an accepted standard because the attendant spatial resolution may demonstrate the RV’s complex geometry. However, MRI is limited in the ICU setting because it may not be available and because it is difficult to continuously monitor critically ill patients in the scanner. Radionuclide scanning is limited by poor spatial resolution, the need for background radiation correction, and lack of portability. Contrast ventriculography is invasive and provides limited incremental information when compared with echocardiography.
Echocardiography is a noninvasive, portable modality that can be used to assess the size and function of the RV. With transthoracic echocardiography (TTE), RV linear dimensions can be measured in end-diastole from the focused four-chamber view. One quantitative approach involves determination of the volumes at end-systole and end-diastole, but this method is limited by the fallacious assumption that the RV is a cylindrical structure. Three-dimensional echocardiography (3D echo) is now recommended to overcome these limitations of two-dimensional echocardiography (2D echo), but in critically ill patients, 3D echo has its own limitations because it relies on excellent image quality and absence of arrhythmias. A good rule of thumb is that the normal-sized RV should be two thirds the size of the LV. When it is larger, the RV is considered dilated.
Right and left heart hemodynamics can be estimated with Doppler techniques. Key measurements include the systolic excursion of the tricuspid annular plane (TAPSE), the fractional area change (FAC), or longitudinal strain. RV function can be visually estimated by examining the contractility of the RV free wall and interventricular septum using TAPSE. Abnormal TAPSE has been shown to have prognostic value, especially in patients with PAH. Although TAPSE has been shown to be the most accurate measurement of RV function in PAH, it may overestimate RV function when there is significant cardiac translation (e.g., because of a large pericardial effusion). Alternatively, TAPSE may underestimate RV function after cardiac surgery, when the pericardium may be inflamed. RV FAC gives an estimate of global RV function; values below 35% indicate RV dysfunction. RV global longitudinal strain has been shown to provide prognostic value in various disease states such as heart failure, acute MI, and pulmonary hypertension. Although they appear to be reproducible and feasible in clinical practice, there currently exists a need for normative data. 3D echo can also provide an RV ejection fraction (EF), with a normal value more than 45%. RV wall thickness, which increases in chronic states, can be measured by either M-mode or 2D echo.
Distinct echocardiographic patterns have been described in patients with RV failure with different causes. RV free wall hypokinesia that spares the apex is known as McConnell’s sign and was originally described in patients with massive or submassive PE; it was thought to be specific for that disorder. However, a retrospective study cast doubt on the specificity of McConnell’s sign; in PE, its sensitivity was 70%, its specificity was 33%, and the sign was present in 67% of patients with RV infarction. A D-shaped, flattened septum during diastole is seen in RV volume overload. Conversely, pressure overload causes right ventricular hypertrophy (RVH) and septal flattening throughout the entire cardiac cycle.
The main pitfall of TTE in ventilated critically ill patients is that the images are often suboptimal and technically limited. Transesophageal echocardiography can be used when TTE images are not interpretable.
Causes of Right Ventricle Dysfunction
The causes of RV failure can be divided into RV pressure overload, RV volume overload, decreased RV contractility, or a combination of these ( Fig. 54-3 ). Sepsis is a disease process that has two potential mechanisms of RV dysfunction: myocardial depression and increased pulmonary vascular resistance (PVR).

Management of Right Ventricle Failure
Definitive therapy for acutely decompensated RV failure requires primary treatment of the underlying condition in addition to hemodynamic support. The RV is very resilient and can recover substantial function if the underlying condition is successfully addressed. Examples include percutaneous coronary intervention for RV MI and thrombolysis or open surgical embolectomy for massive PE.
Right Ventricle Myocardial Infarction
RV MI is a distinct clinical entity, and there is a reasonable evidence base regarding its management. One third of inferior wall MIs are accompanied by RV MI. This typically occurs when there is acute thrombotic occlusion of the right coronary artery (RCA) proximal to the RV marginal branches. In acute RV injury, chamber enlargement, depressed contractility, and impaired ventricular emptying lead to elevated right-sided volume and pressure. RV compliance also decreases, further raising pressures. This leads to conformational change of the RV and affects the LV through ventricular interdependence.
The classic clinical features of RV MI are hypotension, systemic venous congestion, and clear lungs. ECG findings of ST elevation greater than 1 mm in right-sided lead V 4R in the presence of inferior wall injury are reliable and predictive of RV MI (88% sensitive, 78% specific). Other ECG findings include atrioventricular (AV) nodal block and right bundle branch block. Hemodynamic findings include elevated right atrium (RA) pressure in relation to left-sided filling pressures. Equalization of diastolic filling pressures among the RA, RV, and pulmonary capillary wedge (PCW) pressures can also be seen. The steep y descent of the RA pressure tracing and the characteristic dip and plateau of the RV pressure tracing make this entity more hemodynamically similar to constrictive pericarditis and differentiate RV MI from cardiac tamponade. 2D echo is useful in identifying RV chamber enlargement and wall motion abnormalities of the free wall. Paradoxical septal motion can be seen in the presence of RV pressure/volume overload.
RV MI may be complicated by cardiogenic shock and high-grade AV block, both of which affect mortality. RA dilation can lead to atrial fibrillation, which may further affect hemodynamics.
Treatment of RV MI includes close monitoring in a specialized cardiac unit. Unlike LV infarction, the initial treatment is volume expansion. In general, nitrates, morphine, diuretics, and other vasodilators should be avoided. Although patients with RV failure are often preload dependent, volume loading has the potential to overdistend the ventricles and increase wall tension, decrease contractility, increase ventricular interdependence, impair LV filling, and reduce systemic cardiac output. The utility of volume loading appears to depend on various factors, including the baseline cardiovascular function of the patient, the degree of RV afterload, and volume status. A clinical study of fluid resuscitation in patients with RV MI showed that the RV achieves its maximum stroke work with RA pressure from 0 to 14 mm Hg; the optimal PCW pressure was 17 mm Hg in this study. An initial trial of volume may be appropriate for patients with decompensated RV failure, provided there is no evidence of pulmonary edema or increased right-sided preload conditions. If signs of RV volume overload, including a CVP of greater than 15 mm Hg, or septal shift is noted on echocardiography, then the initiation of inotropic support without additional volume administration may be prudent. Pulmonary artery catheterization may be helpful in determining the ideal volume loading conditions.
Hemodynamic support of the patient with decompensated RV failure may require combinations of vasopressors and inotropes. The normotensive patient with evidence of decreased cardiac output should be started on inotropic therapy, with vasopressors added if hypotension develops. The hypotensive patient with decreased cardiac output should receive vasopressors along with inotropes. Dobutamine has been shown to have beneficial effects on RV contractile function in pulmonary hypertension without affecting PVR. Milrinone, a selective phosphodiesterase (PDE)-3 inhibitor, has inotropic and vasodilatory effects, decreasing PVR and increasing RV EF in acute and chronic pulmonary hypertension, but its use may be limited by hypotension. Norepinephrine has inotropic effects through β 1 agonism, but concomitant α 1 stimulation causes vasoconstriction and increased RV afterload. Levosimendan is a calcium sensitizer that increases cardiac contractility and has vasodilatory effects by activating the adenosine triphosphate (ATP)-sensitive potassium channels in vascular smooth muscle. In an animal model of RV failure, levosimendan decreased afterload and increased RV contractility better than dobutamine because of its additional pulmonary vasodilatory effects. Levosimendan is currently not approved in the United States, but it is used in Europe.
One study examined the effects of inhaled nitric oxide (NO) in 13 patients with right ventricular infarction and cardiogenic shock. Acute hemodynamic improvement was seen, with a 24% increase in cardiac output along with a 12% decrease in RA pressure, a 13% decrease in pulmonary artery pressure, and a 36% decrease in PVR. Systemic blood pressure and PCW pressure were unchanged. The presumed mechanism was selective pulmonary vasodilation.
Maintenance of sinus rhythm and AV synchrony can also be crucial in maximizing RV preload and function. Intra-aortic balloon pump counterpulsation may be considered when there is ongoing ischemia or refractory hemodynamic instability.
Fibrinolytic therapy has demonstrated limited benefit in acute inferior MI with RV involvement for various reasons. First, reocclusion has been shown to be more common when the RCA is the infarct-related artery. Second, mortality from acute inferior MI is considerably less than for anterior MI. Finally, RV function has been shown to improve spontaneously over time even in the absence of reperfusion therapy.
A retrospective analysis of 1110 patients enrolled in phase II of the Thrombolysis in Myocardial Infarction trial showed that fibrinolysis reduced the frequency of RV dysfunction in patients with inferior infarction as demonstrated by radionuclide ventriculography. In a prospective trial, tissue plasminogen activator (t-PA) was administered along with antithrombotic and antiplatelet therapy within 4 hours of symptom onset to 90 patients presenting with inferior MI with or without RV involvement. Coronary angiography performed later in the hospital course found that normal coronary flow was more likely in those without RV MI. In RV MI, complications were higher and late vessel patency was only 29% at 12 days after t-PA administration.
The advantages of percutaneous transluminal coronary angioplasty (PTCA) over fibrinolysis include better infarct-related artery patency rates, lower incidence of intracranial hemorrhage, and decreased recurrent ischemia. In a study of 53 patients with inferior and RV MI taken for emergent PTCA, restoration of flow to the major RV branches was achieved in 77% of patients, and those with successful reperfusion had early recovery of RV function, sometimes as early as 1 hour. Those who had unsuccessful reperfusion had protracted hemodynamic compromise requiring inotropic support, with a mortality rate of 58% compared with 2% in the reperfused group. Emergency revascularization efforts in these patients with RV MI is a class I recommendation in ACC/AHA guidelines for the treatment of acute MI.
Mechanical ventilatory support for patients with acute RV failure should aim to improve oxygenation and ventilation without worsening RV impedance, venous return, or diastolic function. Hypoxemia and acidosis should be reversed because they can contribute to increased PVR. A low respiratory rate and low tidal volume should be used to limit air trapping, which may increase PVR. Lower positive end-expiratory pressure settings may also moderate the effect of mechanical ventilation on PVR.
Vasodilator Therapy
The goal of vasodilator use in RV failure is to improve right-sided cardiac output by reducing afterload. There is substantial evidence concerning vasodilator therapy in PAH and fewer data in secondary pulmonary hypertension ( Table 54-1 ). Available therapies include NO, prostaglandins, PDE inhibitors, and endothelin (ET) antagonists.
Authors Drug | Descriptor | Number of Patients NYHA Class Etiology | Results |
---|---|---|---|
Barst et al. Epoprostenol IV (1996) | Multicenter open comparison conventional therapy alone vs. conventional therapy along with an intravenous infusion of epoprostenol | 81 Class III-IV PPH | At 12 weeks:
|
Badesch et al. Epoprostenol IV (2000) | Multicenter open comparison conventional therapy alone vs. conventional therapy along with an intravenous infusion of epoprostenol | 111 Class II-IV scleroderma and mod PHTN | At 12 weeks:
|
Simonneau et al. Treprostinil SQ (2000) | Double blind Placebo vs. treprostinil | 470 Class II-IV PPH, connective tissue disease, congenital left-to-right shunt | At 12 weeks:
|
Galie et al. Beraprost PO (2002) | Double blind Placebo controlled | 130 Class II-III All PAH | At 12 weeks:
|
Barst et al. Beraprost PO (2003) | Double blind Placebo controlled | 116 Class II-III PPH, PAH related to either collagen vascular diseases or congenital systemic to pulmonary shunts |
|
Olschewski et al. Iloprost INH (2002) | Multicenter placebo controlled Used a combined endpoint of a 10% increase in patients’ scores on a 6-min walk test and improvement in NYHA functional class | 207 Class III-IV PPH, CTD, chronic thromboembolic | At 12 weeks:
|
Channick et al. Bosentan PO (2001) | Double blind Placebo | 33 Class III PPH or associated with scleroderma |
|
Rubin et al. Bosentan PO (2002) | Double blind Placebo | 213 Class III-IV PPH or associated with connective tissue disease |
|
Galie et al. Ambrisentan PO (2005) | Double blind Placebo | 64 Class II-III PPH or associated with collagen vascular disease, anorexigen use, or HIV | At 12 weeks:
|
Pulido et al. Macitentan (2013) | Multicenter Double blind, placebo controlled Macitentan (3 and 10 mg) Primary endpoint: time to occurrence of a composite endpoint of death, AS, lung transplantation, initiation of treatment with prostanoids, or worsening of PAH | 742 Class II-IV PPH or related to connective tissue disease, repaired congenital systemic-to-pulmonary shunts, HIV, or drug use/toxin exposure |
|
Galie et al. Sildenafil (2005) | Double blind, placebo controlled Sildenafil (20, 40, 80 mg) | 278 Class II-IV PPH, CTD, and repaired congenital disease | At 12 weeks:
|
Galie et al. Taladafil (2009) | Double blind, placebo controlled Taladafil (2.5, 10, 20, 40 mg) | 405 Class II-IV Idiopathic/heritable or related to anorexigen use, connective tissue disease, HIV infection, or congenital systemic-to-pulmonary shunts | At 16 weeks:
|
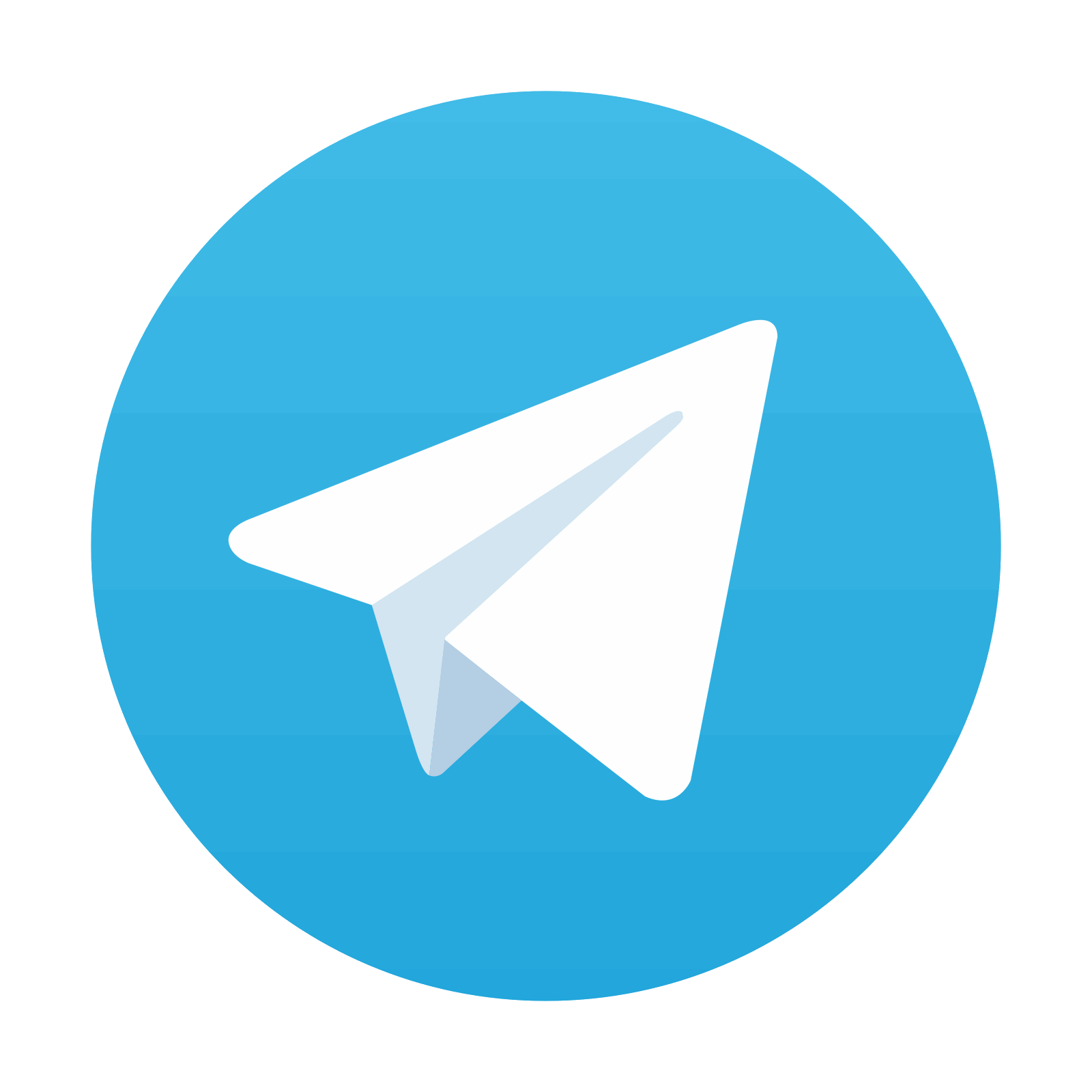
Stay updated, free articles. Join our Telegram channel

Full access? Get Clinical Tree
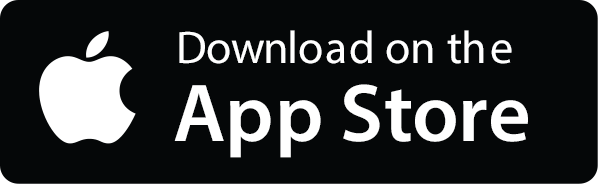
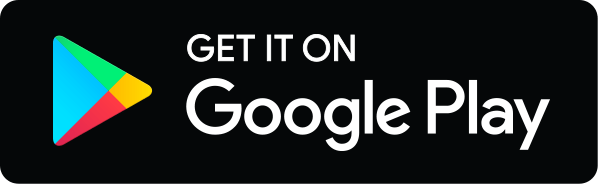
