Description of event
Physics of Blast Injury
Although the exact details of an explosion are rarely available to emergency providers, a basic understanding of explosives and blast-wave physics can help illustrate the underlying pathophysiology of blast injuries and the unique risks facing blast survivors. An explosive blast is an intense exothermic reaction generated by the rapid chemical conversion of a solid or a liquid into a gas. Conventional explosive devices are typically categorized into low- (or “ordinary”) and high-order explosives. Low-order explosives include materials such as propellants (e.g., gunpowder), which undergo a process of rapid burning known as deflagration , at speeds less than 1000 m/s. If low explosives are ignited in the open, they will often burn rather than result in an explosion. Encasing low explosives in small enclosures such as pipes and pressure cookers significantly increases the speed at which the material is ignited and the amount of pressure generated.
High-order explosives (e.g., trinitrotoluene [TNT]) undergo a process known as detonation . In detonation, the ignition of a small amount of the explosive or an igniting agent generates a shock wave, which propagates through the explosive at speeds above 4500 m/s. The remaining explosive ignites within microseconds, releasing tremendous amounts of energy in the form of heat and pressure as the newly formed gas expands, displacing the surrounding air and creating a blast wave. ,
The leading edge of the blast wave, or blast front, consists of a thin layer of compressed air, traveling faster than the speed of sound. When the blast front encounters an object, it causes a virtually instantaneous rise in pressure from ambient pressure to a peak overpressure. This initial wave is followed by a subsonic phase of sustained overpressure, at significantly lesser magnitude than the peak, known as the positive pressure phase . Low explosives can generate substantial positive pressure, but will not generate a shock wave. The total duration of overpressure is typically less than 100 ms for conventional explosive devices. This displacement of air generates a “blast wind,” which can propel objects and people at speeds in excess of 800 miles per hour (357 m/s). The final portion of the explosion is a negative pressure phase, representing the vacuum created by the gas displaced by the explosion, and a return to atmospheric pressure. Although complex formulae exist for modeling explosions, the Friedlander curve ( Fig. 71-1 ) provides a useful illustration of the phases of a blast.

Several factors govern the destructive effects of an explosion and its potential to cause injury. The most significant is distance from the explosion. The overpressure of the explosion decreases proportionally to the inverse cube of the distance from an explosion; thus a person twice as far away from an explosion experiences approximately an eighth of the overpressure. The medium in which an explosion occurs is also significant. As fluid is incompressible, underwater explosions propagate more quickly, with more energy conserved over distance and time, tripling the lethal radius of an underwater explosion versus an explosion in midair.
Finally, the location of an explosion relative to buildings and other solid surfaces can profoundly increase its lethality. Blast waves may wrap around objects and walls and are often reflected by solid surfaces. Reflected waves can undergo constructive interference, resulting in overpressure amplified far beyond that of the initial blast wave. Victims adjacent to or behind a wall may experience worse injuries than those out in the open. This is particularly acute in enclosed spaces, as the resulting reflections of a blast extend the duration of overpressure leading to very complex wave patterns. If a pressure wave is reflected from a solid object, the pressures generated from the reflecting surfaces may be more than 20 times that of the incident wave. Consequently, a blast wave capable of causing only minor injuries in an open space may be lethal to victims in closed spaces. , ,
Physiology of Blast Injury
Blast injury is described in terms of primary, secondary, tertiary, and quaternary mechanisms. Blast victims often suffer from multiple mechanisms of injury, and the categories illustrate how the different effects of blasts cause specific patterns of trauma. , Primary-blast injury (PBI) is a direct result of blast overpressure forces. , Overpressure forces tend to damage air-containing organs and those with varying internal densities, such as the ear, lungs, intestines, and brain. PBI can be further subdivided into shearing, spalling, and imploding. , , Shearing occurs when two adjacent objects of different densities are acted on by the same force. The lighter object accelerates faster than the denser object, creating a shearing stress at their shared boundary. Spalling is the tendency of a dense medium under pressure to displace and fragment into a less-dense medium. The fragments then act as secondary projectiles into the less-dense medium. This occurs frequently in the body when the contents of a fluid-filled organ or vessel are driven into an air-filled or potential space. Implosion occurs when the force of overpressure compresses gas within a tissue. When the period of overpressure ends, the compressed gas expands, disrupting the surrounding tissue.
Secondary blast injury results from projectiles generated by an explosion’s overpressure and blast winds. These projectiles have a much greater radius of effect than the explosion itself. An explosion that causes PBI at a radius of tens of meters may launch projectiles hundreds or even thousands of meters away, causing blunt and penetrating trauma. Blast-related debris may include fragments originating from the casing of the charge, intentional shrapnel enclosed with the explosive (e.g., ball bearings and nails), and secondary fragments, such as pieces of glass, wood, stone, and other materials. Even low-order explosives such as pipe bombs can accelerate shrapnel to speeds equivalent to bullets.
Tertiary blast injury results from victims being accelerated by explosions, who are then knocked into hard surfaces or launched into the air. Once a victim’s body is in motion, they are also at serious risk of being impaled with stationary objects. Children are at particularly high risk of tertiary injury due to their lower weight. Tertiary injury also encompasses crush injuries from collapsing buildings, and when passengers collide with the inside of a vehicle. , ,
Quaternary blast injury is a broad category of injury including thermal and chemical burns and inhalational exposure. Heat generated by the initial explosion may cause flash burns. The release of hot gases, secondary fires in the surrounding environment, and contact with hot dust-laden air can also cause conventional burns. Chemical exposures can result from the release of chemicals in the surrounding environment, along with the inhalation of dust, chemically complex smokes, and carbon monoxide. , ,
Historical context
Increasing Prevalence and Lethality of Explosive Devices
In 1917 the accidental collision of two ships, the Imo and the Mont Blanc , in Halifax Harbor, Nova Scotia, demonstrated the potential for vast death and destruction from an explosive blast. Their cumulative cargoes included 35 tons of benzene, 2300 tons of picric acid, 10 tons of gun cotton, 200 tons of TNT, and 300 rounds of ammunition. The collision resulted in the largest human-made, nonnuclear explosion in history. Casualties reportedly included over 2000 deaths and 9000 injuries. Parts of the ships were found several miles away; approximately 2.5 km of the city was leveled, and windows were shattered as far as 100 km away.
In the modern era, injuries caused by explosives have become an important issue for civilian health care providers because of the dramatic increase in terrorist bombings worldwide within the past few decades. Throughout the 1990s, terrorist bombings led to approximately 3203 fatalities and 19,329 injuries. In the following decade from 2000 to 2009, there were upwards of 30,992 fatalities and 91,571 injuries resulting from terrorist bombings globally.
Even though the great majority of bombings within the United States have been encased low-explosive devices such as pipe bombs, terrorist bombings against military and civilian targets in Iraq and Afghanistan, as well as in many other recent conflicts, have demonstrated a sharp increase in sophistication and lethality. , In part, this has stemmed from the wide-scale availability of military-grade munitions in these regions (primarily high explosives). However, observers have also noted that independent terrorist groups worldwide have dramatically increased the rate at which they learn new tactics, techniques, and procedures of improvised explosive devices (IEDs) use. This is attributed to a greater degree of information sharing between terrorist groups, which can result in a rapid sophistication in tactics and technology within months during a bombing campaign.
Prognostic Factors and Critical Mortality Rates
An instructive explosive attack occurred in 1983 in Beirut, Lebanon, where terrorists utilized a truck-borne, ammonium-nitrate-based explosive device equivalent to roughly 6 tons of TNT to bomb the U.S. Marine Corps barracks. The blast resulted in the near complete collapse of a four-story building, and 234 immediate deaths (68% of all victims). Several first responders were killed by sniper fire from waiting terrorists carrying out a “second hit” assault, an asymmetric tactic occurring more frequently in present-day attacks. Most of the survivors had noncritical injuries. However, 19 survivors (17%) suffered critical injuries and 7 of these initial survivors ultimately died. Timing of response is critical for survival. Six of the seven late deaths had been rescued and treated more than 6 hours after the blast. Conversely, only 1 of the 65 initial survivors rescued within 4 hours died. The reported causes of mortality in Marine barrack bombings included the following : head trauma as the leading cause of immediate death (71%) and late death (57%) ; chest trauma (29%) (rare among survivors) ; and burns (29%).
This event provides important lessons for responding to explosions. Most striking is the effect of early and aggressive resuscitation as a key prognostic factor for long-term survival. Rapid evacuation and treatment must be balanced with operational risks such as terrorist deployment of multiple devices or layered attacks targeting first responders. Building collapse also has a dramatic effect on survival, and the anatomic site and nature of an injury are important prognostic factors. In a review discussing the medical response to terrorist bombings, Frykberg et al. proposed the use of a “critical mortality rate” (the death rate only among those critically injured) as an appropriate measure when calculating vital statistics for a terrorist event. Because the number of noncritical survivors may falsely lower overall mortality rates, the critical mortality rate more accurately describes those victims actually at risk for death.
Triage Efficacy
In 1994 the Argentine Israeli Mutual Association (AMIA) of Buenos Aires, Argentina, was bombed with a large ammonium-nitrate explosive (430 lb TNT-equivalent), leading to the complete collapse of a seven-story building. There were 286 casualties and 82 (29%) immediate deaths. , In the immediate aftermath of the explosion, the closest hospital emergency department (ED) faced rapid overcrowding by ambulatory survivors and medical personnel offering to help. Of the 204 survivors, 40 were eventually hospitalized, 7% ( n = 14) in critical condition, among whom 4 later died. These figures represent a 3.4% overall mortality rate and a 29% critical mortality rate.
Among survivors, 58% ( n = 50) of those who visited the ED suffered minor injuries, 12 of whom were admitted. Moderate injuries were reported among 19% ( n = 16) of survivors presenting to the ED, with 13 ultimately admitted. Major injuries were reported among 21% ( n = 18) of presenting patients, 12 of whom were sent directly to the operating room or intensive care unit; 7 later died. A total of five survivors were extricated from rubble, two of whom later died. Only five patients underwent laparotomy, and hepatic lacerations were found in two of these. Three patients were found to have pneumothorax, and two others showed bilateral infiltrates consistent with PBI of the lung. ,
The AMIA bombing demonstrated that hospitals in close proximity to a major explosion suffer rapid and early overcrowding of their EDs with patients who have sustained minor and moderate injuries. Frykberg et al. compared the AMIA bombing with similar events, demonstrating a very strong correlation between the extent to which noncritical patients are overtriaged and mortality. This illustrates the need for disaster management plans that emphasize appropriate and effective triage to be carried out by experienced providers both in the field and at the ED entrance, as well as the need to appropriately manage providers who volunteer to help with the response.
An example of efficient triage can be found in the Boston Marathon Bombing of 2013. During the bombing, two improvised explosive devices, pressure cookers filled with metallic debris and low-order explosives, exploded within 13 seconds of each other near the finish line of the marathon. Three victims were killed immediately. In total, 264 individuals were injured. Although many of the victims suffered traumatic lower-extremity amputations and required critical care and operative management, there were no further fatalities.
The low fatality rate after the Boston bombing was due to several factors. First, the detonations were in close proximity to multiple Level 1 Trauma Centers, allowing for rapid movement to specialized care. Second, emergency medical services (EMS) personnel had established a nearby triage area at the marathon prior to the bombing, and prehospital providers made a concerted effort to distribute critical patients evenly between the area hospitals to avoid overwhelming the services of any one facility. Third, Boston area hospitals and emergency providers had taken significant measures to prepare for a similar disaster, including multiple mass-casualty drills run by the area’s hospitals in coordination with emergency medical service providers. Finally, many EMS providers had also received training in the use of tourniquets, which were employed effectively for many of the extremity injuries.
Building Collapse
The bombing of the Alfred P. Murrah Federal Building in Oklahoma City, Oklahoma, in 1995 demonstrated the profound effect that structural considerations can play in a bombing. In this incident, an ammonium-nitrate-based bomb exploded with the equivalent of two tons of TNT, causing 759 total casualties with a 21% immediate mortality rate ( n = 162). Fourteen percent of survivors ( n = 83) were hospitalized, of which 52 (62%) were critically injured. Five of these later died (representing a 9.6% critical mortality rate). As with the AMIA bombing, the highest mortality and most severe morbidity in Oklahoma City occurred among victims who were located in collapsed portions of buildings at the time of blast.
There were 506 survivors of the bombing. The injury pattern among survivors included 85% who suffered soft-tissue injuries, including lacerations, abrasions, contusions, and puncture wounds. Among survivors, 210 (35%) had musculoskeletal injuries, 60 suffered from fractures or dislocations, and the remainder had sprains or strains. Fewer patients had severe soft-tissue or musculoskeletal injuries. The majority of survivors were injured by flying glass, collapsed ceilings, and other debris.
Open Air Versus Confined Space
Explosions in confined spaces cause markedly different patterns of injury. In a review of open air (OA) versus confined space (CS) terrorist bombings in Israel, Leibovici and colleagues compared 297 victims from two OA bombings and two bus bombings in which similar explosive devices were used. The CS group suffered a significantly greater incidence of PBI, with higher mortality and a greater severity of injury, whereas the OA caused a larger number of less severe injuries. These findings may be due to unique blast wave behavior within the relatively small CSs of buses, and the ability for OA blasts to launch shrapnel in a much wider radius. A study by Golan et al. reviewed 22 terrorist attacks against civilian buses, comparing explosions that occurred adjacent to buses with explosions within them. This study confirmed the earlier group’s finding of a higher mortality rate in CS explosions, as well as a higher rate of pulmonary blast injury.
Medical treatment of casualties
Most severely injured survivors of blasts have multiple injuries. , The most common injury patterns seen among explosion survivors are due to secondary and tertiary blast injury. Given the relative similarity of these injury patterns to the patterns of penetrating trauma, blunt trauma, and burns commonly seen in civilian EDs, the following discussion will emphasize PBI. Anatomically, the structures at greatest risk for PBI are the auditory system, thorax, and abdomen. , PBI may also lead to injuries of the central nervous system (CNS), eyes, and extremities. The greatest potential for avoiding preventable deaths by PBI lies in the appropriate treatment of thoracic PBI. The incidence of PBI varies according to explosive charge, proximity of the victim to the blast, and the environment in which it occurs. , Survivors of explosions that occur in CSs or underwater may manifest a higher incidence of PBI. ,
Primary Stabilization
The initial response to bombings is a high-risk operation that must address issues of security and casualty care. The principles of Tactical Emergency Casualty Care (TECC), discussed in detail in other sections, offer threat-based principles of prehospital care to address major potentially preventable causes of death in terrorist attacks: specifically, early hemorrhage control, casualty evacuation, and rapid medical assessment. The majority of survivors injured by a blast are likely to have suffered secondary blast injuries from shrapnel. Accordingly, immediate control of potentially life-threatening extremity hemorrhage is critical, and the rapid use of tourniquets has been shown to significantly improve outcomes.
Patients at risk for PBI should not be intubated in the prehospital setting by less-experienced providers, but should receive very limited fluid resuscitation. In the ED, the initial management of blast victims begins with the conventional priorities outlined in the advanced-trauma life-support protocols; however, there are several important exceptions.
Thoracic Injury
The primary organ at risk from PBI to the thorax are the lungs. Blast lung injury (BLI) is a major cause of death in patients who survive initial resuscitation from blast injury. In victims exposed to significant overpressure, blast waves cause an inward displacement of the chest wall that compresses air within the lung parenchyma more slowly than in the hollow respiratory tracts. , The alveoli implode and reexpand, compromising the alveolar walls, surrounding parenchymal tissue, and capillaries, whereas spalling leads to the extrusion of capillary contents and extravascular fluid into the alveoli. This dramatically decreases lung compliance, and can introduce air emboli into the vascular space. , The resulting lesions can range from scattered or multifocal petechiae to large confluent hemorrhages involving an entire lung. , Pleural and subpleural hemorrhages tend to be bilateral, but are usually more extensive on the side facing the source of blast.
Patients with BLI may present with dyspnea, cough, hemoptysis, chest pain, and a clinical picture that resembles pulmonary contusion or acute respiratory distress syndrome (ARDS). , Clinical signs associated with BLI include tachypnea with rapid, shallow breathing; poor chest wall movement (owing to decreased compliance); dullness to percussion; decreased air movement; hemopneumothorax; subcutaneous emphysema; and retinal artery emboli. Progression of BLI may be due to the cumulative effect of the initial blast injury, inhalation injury, hypovolemic shock, sepsis, aspiration, and aggressive fluid resuscitation. Ultimately, the diagnosis of blast lung is suspected on clinical grounds but confirmed with radiographs. Signs and symptoms usually develop within hours after an explosion, but may be delayed as long as 24 to 48 hours. , ,
Chest radiography is required for all patients exposed to blast forces to gauge the initial severity of injury and should be obtained in patients with any significant clinical change. Bruising of the intercostal spaces unprotected by ribs often appear on initial chest x-rays as rib markings. , Diffuse pulmonary opacities may develop within a few hours and will become maximal within 24 to 48 hours. They typically occur in a “butterfly distribution” of bilateral patchy infiltrates. Changes that develop after 48 hours may be due to complications such as ARDS or pneumonia. Other radiological findings may include hemopneumothorax, pneumomediastinum, subdiaphragmatic free air, subcutaneous emphysema, and foreign body impaction. Symptomatic patients without clear radiographic evidence of injury should undergo emergent CT imaging. CT has a higher sensitivity for early parenchymal lesions in patients with nonblast-related pulmonary contusion, and may be useful in predicting which patients will require mechanical ventilation.
The general management of BLI is similar to that of pulmonary contusion and ARDS. However, the relatively higher risk of significant barotrauma (i.e., alveolar rupture, systemic air emboli, and pneumothorax) in patients with BLI is critically important. , , Positive pressure ventilation, especially high levels of positive end-expiratory pressure, increases the risk of pulmonary barotrauma and should therefore be avoided when possible. Further, patients with BLI are at increased risk of complications from overly aggressive fluid resuscitation. ,
Several measures have been shown to reduce the risk of complications in BLI. Permissive hypercapnia and lower oxygen saturations coupled with reduced tidal volumes (e.g., 5 to 7 mL/kg) and peak inspiratory pressures have shown good outcomes. High-frequency oscillatory ventilation may benefit refractory cases. Although data are limited, some authors recommend bilateral tube thoracostomy for pulmonary PBI patients requiring aeromedical evacuation. , The use of dual-lumen endotracheal tubes (i.e., independent lung ventilation) or high-frequency jet ventilation is often recommended for patients diagnosed with bronchopleural fistulae. ,
The treatment of arterial air emboli resulting from BLI also remains controversial. Even though lowered positive end-expiratory pressure (PEEP) should be continued to prevent the entrainment of further emboli, a number of authors recommend the administration of 100% inspired oxygen to aid in the reabsorption of existing emboli. Extracorporeal membrane oxygenation may be indicated in extreme cases. ,
A retrospective study of patients sustaining BLI from explosions on civilian buses in Israel established that the first 24 hours after injury are critical for effective management. The BLI severity score (BLISS), derived from objective signs of hypoxemia, chest radiograph findings, and the presence of bronchopleural fistula, helps to direct treatment and can predict outcome during the initial stabilization phase. Based on the initial BLISS, none of the patients in this study who were classified as mildly injured went on to develop any form of lung injury. Of those classified as moderately injured, 33% went on to develop ARDS. All those classified as severely injured either developed ARDS or later died. This study showed that aggressive and complex interventions, guided by stratification of BLI severity, can lead to dramatic improvements in outcome. Most patients who survive BLI will regain good lung function within 1 year. ,
Cardiovascular Injury
Victims of blast injuries may have hemodynamic instability that is not explained by conventional causes such as hemorrhage. Much of what is known about the PBI of the cardiovascular system has been gleaned from experimental studies in animal models and historical inquiry. A World War II study of 200 casualties assessed immediately after blast exposure showed that more than 25% of them had heart rates of less than 60 bpm and that over 90% had heart rates of less than 80 bpm. This review also found that hypotension was nearly universal, with blood pressures of 80 to 90 mm Hg (systolic) and 40 to 50 mm Hg (diastolic) being common. This bradycardia has been consistently reproduced in animal studies and may be a vagal effect, as experiments have shown the bradycardia can be prevented with bilateral vagotomy. ,
Irwin and colleagues proposed that these vagally mediated cardiovascular changes are initiated by the so-called pulmonary defensive reflex. This reflex acts when acute pulmonary congestion and edema lead to fluid shifts in the lung, stimulating pulmonary C-fibers, and triggering increased cholinergic activity with systemic effects. This mechanism is supported by the clinical observation of brief periods of apnea after blast exposure, followed by rapid shallow respirations. This phenomenon has also been observed in experimental models using artificial stimulation of pulmonary C-fibers. Several studies have reported a greater than 50% decrease in mean arterial pressure in animals immediately after blast exposure, with spontaneous resolution over time.
Various electrocardiographic changes have been observed immediately after blasts and are thought to be due to blunt thoracic trauma and/or coronary air emboli. These may include atrioventricular and bundle branch blocks, nonspecific ventricular ectopy, low-voltage QRS complexes, and T-wave and ST-segment abnormalities. Blast-induced electrocardiographic changes typically revert to normal sinus rhythm within a few minutes, but may deteriorate into sustained dysrhythmias, including lethal ventricular arrhythmias. Ischemic changes may also occur because of complications of air emboli.
Clinically, cardiovascular PBI may be complicated by coexisting hemorrhage. Given the importance of judicious fluid administration (including blood products) in resuscitation, and the potential to exacerbate soft-tissue injury by underresuscitation, invasive monitoring is often necessary to guide therapy. ,
Abdominal Injury
Nonlethal abdominal PBI is almost exclusively found in gas-containing organs and is likely caused by forces including shearing, spalling, and implosion. Solid-organ damage may present as subcapsular hematomas or lacerations of the liver, spleen, and kidney. These injuries are thought to be due to acceleration-deceleration caused by either the initial effects of the blast wave or from secondary and tertiary mechanisms of injury. The incidence of intestinal PBI may be higher in victims of underwater and closed-space explosions.
The classic lesions of intestinal PBI involve small, multifocal intramural hematomas. Initial bleeding in submucosal regions may range from scattered petechiae or large confluent hematomas. Partial- or full-thickness lacerations may occur, as well as separation of individual layers of the intestinal wall, leading to immediate or delayed perforation of the bowel. Shearing may also lead to separation of the intestine from its vascular supply and subsequent ischemia. The cecum and terminal ileum are the most likely areas to contain gas and are the most prone to perforation during blast exposure. ,
Because it is relatively uncommon, intestinal PBI presents a diagnostic challenge. Signs and symptoms of intestinal PBI include abdominal or testicular pain, nausea, vomiting, tenderness, absence of bowel sounds, and other peritoneal signs. Initial evaluation may be complicated by the overall acuity of a multiply injured patient or prior administration of pain-control medication. , Gastrointestinal injury may be delayed as long as 48 hours after the initial blast exposure. Intestinal hematomas have been reported to rupture up to 2 weeks after the initial injury. , This may be due to latent injury with delayed perforation as opposed to delayed diagnosis. Currently, no reliable clinical predictors exist to help clinicians determine which patients may progress to delayed perforation.
Ultrasound and abdominal CT are essential for the evaluation of intestinal PBI. , Although abdominal CT has excellent utility in detecting solid-organ injury and existing perforation, because of the potential for abdominal PBI to result in delayed perforation, a negative abdominal CT should not be used to exclude injury in symptomatic patients. , Indications for urgent laparotomy are similar to those established for blunt abdominal trauma.
Musculoskeletal/Extremity Injury
Even though most blast injuries involving the musculoskeletal system are due to secondary and tertiary blast injury, many traumatic amputation in blast victims are due to PBI. In traumatic amputations, the initial shock wave may cause diffuse microfractures of bone, whereas sustained positive pressure from the blast wave leads to significant damage to the periosteum, marrow, and surrounding soft tissue. The blast wave also imposes significant shearing stress at the site of the initial microfractures, causing amputation of the limb with a typically oblique pattern. The characteristic blast-related traumatic amputation traverses the proximal third of the tibia, resulting both from the aforementioned forces and the movement allowed by the knee and ankle joints. , These injuries are associated with very high rates of immediate and delayed mortality and should prompt an extensive search for other injuries. Traumatic amputations should be treated immediately with proximal tourniquets.
Primary blast forces have also been implicated in compartment syndrome. Although the exact mechanism of soft-tissue injury by PBI is unknown, a combination of tissue disruption, the release of inflammatory mediators, and aggressive fluid resuscitation may contribute to the relatively high rate of compartment syndrome in otherwise uninjured limbs exposed to blasts. , , Secondary orthopedic blast injury results from materials projected by the blast into tissue and bone. Secondary bony injury commonly results in two patterns of fracture depending on the velocity and size of the object causing the trauma. High-energy objects often cause bones to shatter on impact, leading to comminuted fractures with significant amounts of devascularized fragments, which are frequently contaminated. Lower energy objects have a tendency to result in injuries to a single bony cortex, leading to a characteristic “drill-hole” pattern, which often does not require operative management. ,
When blast fragments pass through soft tissue at high velocity, they tend to create cavities that are much larger than the trajectory of the fragment itself. The passage of the fragment through tissue causes a high-pressure compression wave to travel through the surrounding tissues. The resulting expansion leads to low pressure in the fragment pathway, sucking debris inward through the entry and exit wounds. As the fragments projected by a blast tend to be more irregularly shaped than bullets, they also transfer a much greater amount of energy to the surrounding tissues, further increasing cavity size. In particular, the cavitation associated with gluteal injuries (which are prevalent in ground-level blasts) can extend to involve nearby major vessels.
Tertiary musculoskeletal injuries involve similar forces and mechanisms to blunt trauma in the civilian setting; however, the location and direction of the forces exerted by explosives can lead to patterns of trauma that are less common in civilian blunt trauma. Low-lying explosives can lead to calcaneal fractures and the “umbrella-ing” of the tissue planes of the lower leg (particularly in land mine-related injuries), whereas explosions under vehicles can cause dramatic axial loading of the occupants and significant spinal trauma, including chance and low-lumbar burst fractures, as well as lumbosacral dissociations.
When there is substantial hemorrhage from extremity wounds, tourniquets should be applied immediately. In the prehospital setting, grossly deformed fractures should be reduced if doing so will not significantly delay transport. Open fractures should be externally fixated quickly after irrigation. Fractures of the upper extremities can also be splinted when feasible. ,
Extremity injuries are at high risk of infection. Approximately 15% of patients with extremity injuries will develop osteomyelitis, of which about 17% will develop recurrent infections. Soil contamination of wounds is frequent, and may include multidrug-resistant Acinetobacter baumannii and Pseudomonas aeruginosa , extended-spectrum β-lactamase-producing Klebsiella and Escherichia coli , and methicillin-resistant Staphylococcus aureus . Current U.S. military and the Infectious Diseases Society of America (IDSA) guidelines derived from casualty data from the conflicts in Iraq and Afghanistan recommend rapid administration of IV or oral antimicrobials as soon as possible. There is considerable evidence that administering antibiotics within 3 hours of injury improves outcomes, and animal models demonstrate improvements with even more rapid administration. As with any soft-tissue injury, updated tetanus prophylaxis should be given to appropriate patients.
Cefazolin remains the initial antibiotic of choice, with clindamycin as an appropriate alternative for patients allergic to β-lactam antibiotics. This is due to the fact that bacteria that initially contaminate wounds are usually gram-positive organisms sensitive to this regimen, and are effectively halted when antibiotics are combined with expedient large-volume low-pressure irrigation and debridement. Most of the bacteria responsible for multidrug-resistant wound and bone infections are actually nosocomial. The current advised regimen consists of 2 g of IV cefazolin every 6 hours for 5 days. If no infection is present on reassessment at day 3, therapy may be halted. Exceptions are made for delayed presentations (after 72 hours) and land mine injuries, for which adding IV metronidazole is recommended.
In the case of suicide bombings, or when the remains of other victims may contaminate wounds, hepatitis B vaccination is prudent for unimmunized individuals. Explosives may be intentionally contaminated with feces or other biological materials. Even though there are no established guidelines for this situation, extensive debridement and irrigation remain appropriate, with the addition of metronidazole to the initial antibiotic regimen. To date, there have been no large-scale accounts of explosives being used to spread specific agents of biological warfare, such as tularemia, smallpox, or anthrax; however, providers should remain aware of this serious possibility.
The conventional military approach to small-fragment wounds has involved treating penetrating wounds in an aggressive fashion with early exploration, debridement, and delayed primary closure. However, recent evidence suggests that a more conservative approach may be appropriate, provided that bacterial colonization is prevented with appropriate antibiotic coverage. This strategy is valid when the following criteria are met : involvement of soft tissue only with no breach of pleura or peritoneum and no major vascular involvement and an entry or exit wound of less than 2 cm in maximum dimension that was not frankly infected and not caused by a mine blast.
Wound closure for blast-related extremity wounds is typically delayed to approximately 5 days from the time of injury to ensure that there is no evidence of infection prior to closure. Exceptions are made for open dural injuries and facial wounds. Studies are currently underway to investigate whether earlier closure may provide lower rates of nosocomial infection, and there are a number of emerging therapies for the treatment of extremity wounds. Early administration of negative pressure wound therapy after debridement has gained widespread acceptance, and antimicrobial-impregnated bead therapy and biofilms are promising technologies currently under investigation.
Central Nervous System Injury
Traumatic brain injury (TBI) is a common result of blasts, and it ranges from minor concussions to fatal intracranial hemorrhage. The primary effects of blast overpressure can lead to neurologic injury; however, the majority of severe TBIs are thought to be due to secondary and tertiary mechanisms. This is due to the fact that the brain (within the skull and cerebrospinal fluid [CSF]) is significantly more tolerant of high pressures than the lungs, and pressures capable of causing fatal pulmonary injuries in a majority of subjects will cause only mild concussive injury to the brain. ,
Mechanisms for isolated PBIs without secondary or tertiary injuries to the brain remain a topic of intense debate. In part, this may stem from the fact that significant PBIs are often associated with other mechanisms of injury. In a study of blast-related TBI survivors by Mac Donald et al., using diffusion tensor imaging to evaluate for axonal injury, all subjects had a mechanism of head injury in addition to PBI. Additionally, more subtle PBIs to the brain may be too small for the resolution of in vivo neuroimaging. Animal models for PBI-induced brain injury suffer from significant differences in the terms of geometry and scale of the human brain versus those of other animals.
Secondary and tertiary mechanisms of TBI can result in penetrating intracranial injury, skull fractures, laceration of major vessels, cerebral contusions (coup and countercoup effects), and acceleration-deceleration injuries as seen in more common causes of TBI. Even though the total contribution of PBI to blast-related TBI remains uncertain, a number of pathological features distinguish blast-related TBI from other causes, such as rapid development of edema, frequent rates of subarachnoid hemorrhage, and a higher tendency toward vasospasm and pseudoaneurysm development.
TBIs are classically stratified via the Glasgow Coma Scale (GCS), into severe (below 8), moderate, and mild. , , , Severe and moderate TBI patients often have profoundly altered mental status and focal neurologic deficits. The effects of mild TBI are subtle and can be easily missed, as the gross majority of these patients will have no obvious deficits. , Patients with moderate and severe TBI are at extremely high risk of delayed intracranial hemorrhage and cerebral edema and should be evacuated immediately to a center with neurosurgical capability. Early invasive monitoring and the capability of performing a decompressive craniotomy are vital. Providers should aggressively treat hyperthermia in severe blast-related TBI.
The management of patients with concurrent TBI and hemorrhagic shock is complicated. Even though a degree of permissive hypotension is advocated in the management of uncontrolled hemorrhage in thoracic and abdominal trauma, systolic blood pressures below 90 mm Hg are closely tied to worse outcomes in TBI (pressures at or below 80 mm Hg systolic have also been linked to worse outcomes in blast trauma to the thorax and abdomen). Similarly, both underoxygenation and overoxygenation are problematic in the treatment of TBI. In patients with TBI, oxygen saturation should be kept above 90%, and rapid sequence intubation (RSI) should not be provided in the prehospital setting unless administered by providers with sufficient training in its performance, because of the risk of hypoxia and delayed transport.
Mild blast-related TBI is associated with brief loss of consciousness or awareness and can lead to headache, confusion, amnesia, and anxiety. Although these symptoms often resolve within a few hours or days after the injury, they may lead to long-term changes in personality and intellectual function. , Patients with mild blast-related TBI may have some minor pathophysiologic and neuropsychiatric differences from those with mild TBI resulting from blunt trauma, although this does not affect immediate management. , Even though there is an evolving role for neuroimaging in the long-term assessment of blast-related mild TBI, a more promising route for the immediate diagnosis of mild TBI can be found in clinical assessment scales such as the Military Acute Concussion Evaluation (MACE), as well as a number of rapid computer-assisted neuropsychiatric tests under development.
Even though the effects of mild TBI are not by themselves life-threatening, missing a mild TBI can seriously endanger survivors and providers. The deficits caused by mild TBI can significantly impair judgment and concentration, putting victims at risk for repeated head injuries. So-called second impacts dramatically increase the potential morbidity and mortality of even minor head trauma. Accordingly, providers should strongly discourage blast victims at risk for TBI from returning to rescue efforts once they have been evacuated. There is a substantially increased incidence of posttraumatic stress disorder (PTSD) in patients after blast exposure, and the risk is particularly high in patients who have suffered a mild TBI. Providers should refer blast victims to appropriate mental health providers both for general counseling and to help screen for the development of PTSD or “second-victim” syndrome.
Auditory Injury
The ear is the organ most frequently injured by explosions, but auditory PBI is frequently overlooked in the context of more serious coexisting injuries during mass-casualty scenarios. The auditory system is uniquely predisposed to PBI because the tympanic membrane (TM) acts as an efficient means for transmitting pressure waves to the middle and inner ear. Although auditory PBI rarely requires emergent intervention, recognition and appropriate referral can profoundly reduce long-term morbidity. All survivors of a blast should have an otologic assessment and audiometry at some time in their aftercare.
Symptoms of auditory PBI include tinnitus, otalgia, and a feeling of fullness. Perforation of the TM is a hallmark of auditory PBI and is typically seen as multiple “punched out” lesions or radial lacerations. The pars tensa is usually involved. , Other signs include hearing loss, blood and/or debris in the ear canal, and disruption of the ossicles. The presence of tinnitus and hearing loss may be initially profound; these findings are usually self-limited and tend to improve quickly. Prolonged duration of these symptoms may be seen in survivors of blasts occurring in CSs.
Vertigo is uncommon in auditory PBI, and its presence should prompt evaluation for neurologic injury. The complication of perilymph fistula should be considered in a patient with vertigo and sensorineural hearing loss, particularly if these findings are fluctuating. This is the only component of auditory PBI requiring prompt surgical treatment (i.e., emergent tympanotomy and fistula repair). Cholesteatoma may be a late complication (12 to 48 months) of TM perforation. Eighty percent of TM perforations heal spontaneously, and nonoperative management is appropriate for most cases. , Large perforations may warrant surgical repair, but elective tympanoplasty can safely be delayed for up to 12 months with good outcomes. Antibiotics are not indicated unless underlying infection is suspected.
Given the relatively low levels of pressure needed to rupture the eardrum relative to the pressure needed to injure other organ systems, a number of authors have suggested using eardrum rupture as a means of triaging patients. , It is important to note that TM perforation correlates poorly with PBI involving other organ systems and should not be used as a marker of latent PBI elsewhere in the body. This is particularly acute in closed-space explosions, where many survivors may demonstrate pulmonary blast injury without evidence of TM rupture. , However, in the case of OA blasts, current evidence suggests that patients who present with isolated TM perforation but are otherwise asymptomatic, with a normal chest x-ray, may be safely discharged after 6 hours of observation. ,
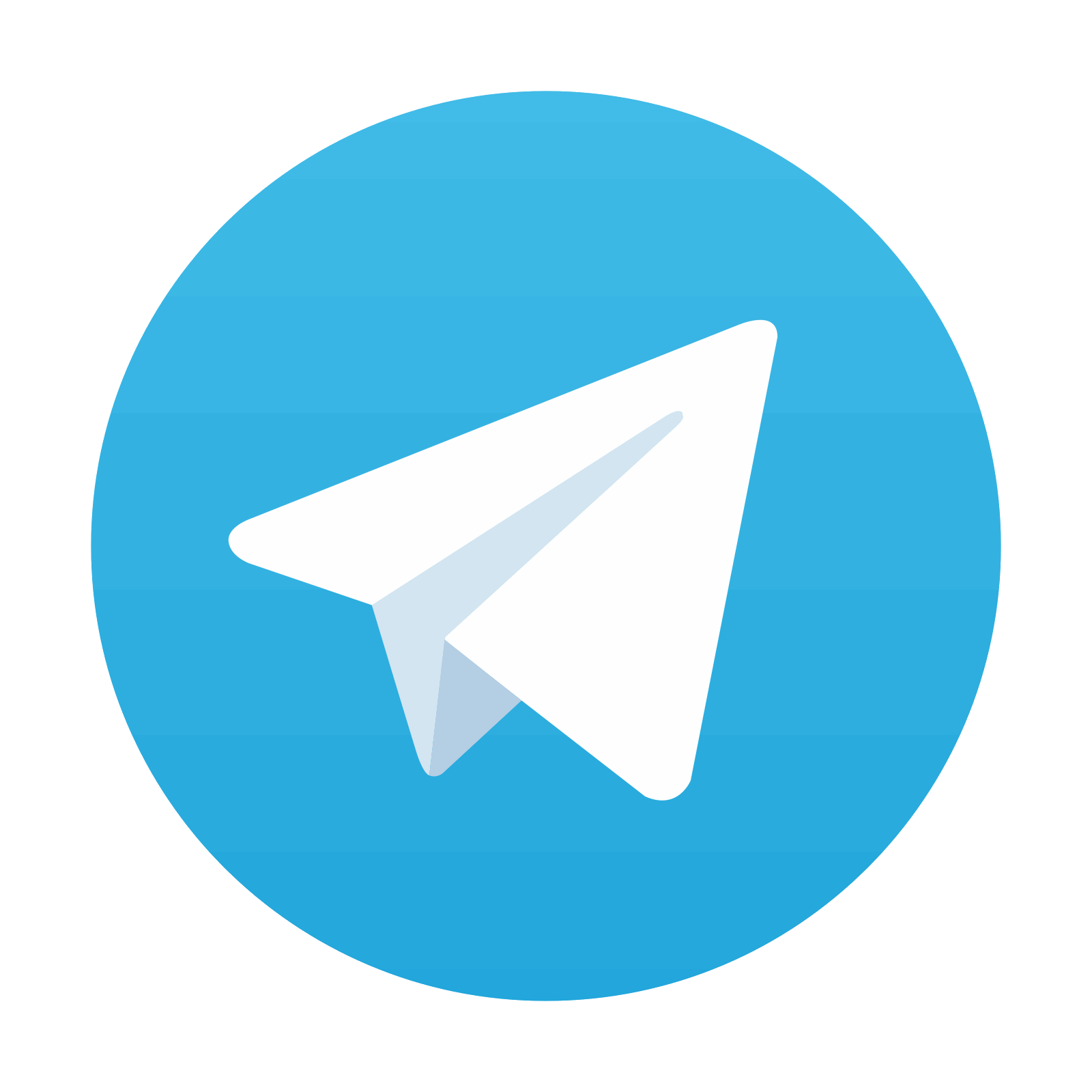
Stay updated, free articles. Join our Telegram channel

Full access? Get Clinical Tree
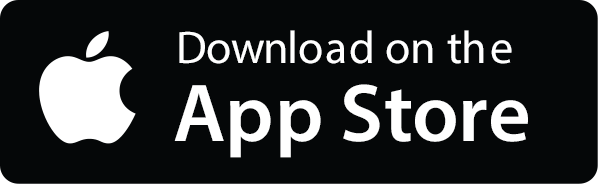
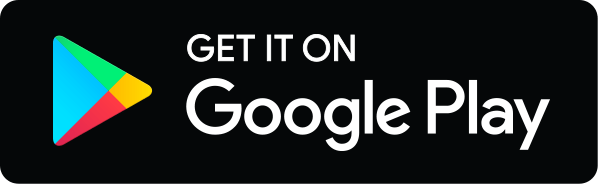